ABSTRACT
The effects of the addition of crystal promoting additives to compound chocolate viscosity and melting characteristics were evaluated in this study. Three types of crystal promoters, namely CP1, CP2, and CP3, were added at varying concentrations (0.25, 0.5, 0.75, and 1.0%). The compound chocolate with no added crystal promoters served as a control. Increase in viscosity with increasing additive concentration was observed among all samples regardless of additive type. Addition of CP1 resulted in significant changes in dimensions and shape deformation after incubation at 40°C for 24 h except at 0.25% concentration. Samples with CP2 and CP3 were fairly stable at 40°C with only CP3 0.25% melting. Crystal promoter type influenced the melting properties of the samples, as determined through differential scanning calorimetry, particularly in terms of Tindex and ΔHM. The ΔHM of all the samples decreased, while the addition of CP1 decreased Tindex values, and adding CP2 and CP3 increased the Tindex. Additive type had limited effects on Tpeak, Tonset, and Tendset. Results suggest that crystal promoters added to compound chocolate affect their viscosity and thermal behavior.
Introduction
Compound chocolates provide confectioners with an alternative to chocolate that is both economical and convenient. Instead of using cocoa butter, compound chocolates are produced from a variety of vegetable fats of lauric or non-lauric origins, and can be used in combination with sugar, cocoa powder, and milk products. Compound chocolates can be used either to enrobe centers (biscuits, fruits, nuts, ice cream, etc.) or be formed into shells for subsequent filling.[Citation1] They have a good product structure as the vegetable fats used are solid at ambient temperatures and above, but still melt at mouth temperature (usually around 37°C). The usage of a high melting point fat increases the heat resistance of compound chocolate products. Since cocoa butter in chocolate starts to soften at about 28°C with consequent loss of structure as temperatures rises, compound chocolate offers a better option.
Cocoa butter crystallizes in six different polymorphic forms of which βV and βVI are the most stable. In chocolate production, the crystal form βV is the desirable form preferred by consumers, not only for its melting characteristics, but also its hardness.[Citation2] Cocoa butter replacers (CBRs) used in the production of compound chocolates are β’-stable, crystallizing directly into this crystal form without the need for tempering or anything more than a slight pre-crystallization.[Citation3] It is necessary for chocolate and compound chocolate to be in their stable crystal forms to attain high quality and to maximize product stability and shelf life. Crystallization, for instance, affects the melting behavior of chocolates. The presence of less stable polymorphs with lower melting points could result in chocolate that may melt too readily in the hand. Since finger temperature is just a few degrees lower than mouth temperature, the desired polymorph is one which has a melting point between that of hand temperature (typically 29–32°C) and mouth temperature.[Citation2]
The rheology or flow properties of compound chocolates are also controlled in order to obtain good quality products. When used in the enrobing process, for example, it is important to control the flow properties for proper coating thickness, protection against feet formation and to ensure pattern holding. Molten chocolate behaves as a non-Newtonian liquid, with flow properties quantified in terms of yield value, related to the amount of energy required to initiate fluid flow, and apparent (plastic) viscosity, the energy required to keep fluid in motion.[Citation4,Citation5] Processing conditions, particle size distribution and composition (fat content, emulsifier content and type, and moisture content) affect the flow properties of chocolate.[Citation6]
Emulsifiers are a class of food additives used in the production of chocolate having great importance on product quality. Some emulsifiers are used in chocolate formulations as rheological properties improvers acting as surface-active agents. These additives coat the solid surface of sugar particles and form a boundary layer between it and the fat, thus aiding flow.[Citation4] Addition of an emulsifier is a practical alternative to adding cocoa butter, the most expensive bulk ingredient in chocolate production, in reducing viscosity and obtaining optimum flow properties. The effect of using lecithin and polyglycerol polyricinoleate (PGPR) in chocolate, individually and in combination with each other, has been studied to a large extent.[Citation6] Over the years, the effects of using other additives such as ammonium phosphatide (YN), sorbitan and glycerol fatty acid esters, and citric acid esters (CITREM) have also been studied in chocolate.[Citation7–Citation9] Studies involving incorporation of additives to compound chocolate in order to modify viscosity properties, while available, are quite few and outdated.[Citation10–Citation12]
Some emulsifiers function as crystal modifiers to influence fat crystallization by affecting either crystal nucleation or growth or both, acting as crystal promoters (CP). The effect of these additives is associated with the creation of imperfections, wherein they may promote crystallization by favoring molecular displacements.[Citation13] The influence of additives in chocolate and cocoa butter crystallization is probably the application that has been investigated the most.[Citation14–Citation18] The role of additives as crystallization modifiers in compound chocolates has not been fully investigated in literature.
The aim of this research was to determine the effects of adding and using different concentrations of crystal promoter additives in terms of both viscosity and crystallization (melting) characteristics of compound chocolates.
Materials and methods
Materials
The compound chocolate ingredients, which included fully hydrogenated palm olein, sugar, cocoa powder, lecithin, PGPR, and vanillin, were kindly provided by Monde Nissin (Thailand) Co., Ltd. Three additives that acted as CP were supplied by Palsgaard A/S (Juelsminde, Denmark). The technical specifications of the fully hydrogenated palm olein and physico-chemical specifications of the additives are listed in and , respectively.
Table 1. Technical specifications of fully hydrogenated palm olein used.
Table 2. Composition and physico-chemical data of crystal promoters used.
Preparation of compound chocolate samples
Dark compound chocolate was prepared with fully hydrogenated palm olein (30% w/w), a CBR, sugar (48.9%), cocoa powder (20%), lecithin (0.5%), PGPR (0.5%), and vanillin (0.1%), through refining and conching by a Böhnke and Luckau Laboratory Ball Mill BL-B5 (Böhnke and Luckau GmbH, Wernigerode, Germany) at 60°C until particle size of less than or equal to 25 μm was obtained. Compound chocolates were formulated with total fat content of 33%. The three types of CP, which were added to the experimental samples at four concentrations (0.25, 0.5, 0.75, and 1.0%), were first dissolved in 10% of the liquid CBR before incorporating with the rest of the compound chocolate mixture after PGPR was added. The compound chocolate with no added CP was also prepared and served as a control. The viscosity of all samples was determined straight away after production. Compound chocolate was molded using silicon molds, allowed to cool to 9°C in a Toshiba GR-R32KD(SZ) refrigerator (Toshiba Thailand Co., Ltd., Bangkok, Thailand) for 2 h, before de-molding onto plastic trays and screened for melt resistance. Additional molded samples were stored in plastic containers (Calcium carbonate and Polypropylene, Fafa Company Ltd., Chachengsao, Thailand) at room temperature (30°C) prior to melting profile analysis within 1 week.
Viscosity
Viscosity measurements were carried out immediately after compound chocolate preparation prior to molding. The liquid chocolate (500 mL) was poured into a 600 mL beaker and the viscosity of the samples was determined at 40°C using a Brookfield viscometer DVII+ Pro (Brookfield Engineering Laboratories, Inc., Massachusetts). The following settings were used: 2.5 rpm, spindle 5, 60 s. Each sample was analyzed in triplicate.
Melting resistance
A modified method of determining the resistance to melting of compound chocolates based on the procedure mentioned in the invention of Alander et al.[Citation19] was used. Instead of storing for 3 h, molded chocolates that were freshly produced were stored in a Memmert UNE 500 incubator (Memmert GmbH + Co. KG, Schwabach, Germany) set at 40°C for 24 h. After which, evidence of melting was noted based on changes in sample dimensions before and after incubation. Height measurements were carried out on nine positions of the molded sample; that is, on the four corners of the mold, the mid between each corner and at the center of the mold. Length measurements, on the other hand, were assessed on the four sides of the mold. The physical dimensions were measured in millimeters using standard laboratory callipers. Changes in height (ΔH) and length (ΔL) were calculated by subtracting the initial value (before incubation) from the final value (after incubation), then dividing the result by the initial value, and multiplying by 100. The resulting sign indicates whether the change is a decrease (–) or an increase (+). Five replications were done for each sample.
Melting profile
The melting behavior of the compound chocolate samples was determined by differential scanning calorimetry (DSC). Approximately 2.0–3.0 mg of the sample was sealed in a stainless pan, crimped tightly closed with a sealing press, and placed in the sample chamber of the Perkin Elmer DSC Pyris1 (PerkinElmer, Inc., Massachusetts), with an empty pan as a reference. The temperature was set to −20°C and then raised by 10°C/min to 80°C.[Citation20] Sample scanning was done in an atmosphere of nitrogen. Melting characteristics of the sample were obtained from the DSC curve. Onset temperature (Tonset), peak temperature (Tpeak), end temperature (Tendset), and enthalpy of melting (ΔHM) were automatically calculated by the Perkin Elmer software. Melting index (Tindex) was computed as (Tendset–Tonset), as described by Vasanthan and Bhatty.[Citation21] All DSC measurements were performed in duplicate.
Statistical analysis
Experiments were done in triplicates according to randomized complete block design (RCBD) with replication as block. Data were analyzed using XLSTAT statistical software v.2007.1 (Addinsoft, New York). Analysis of variance (ANOVA) and Duncan’s Multiple Range Test (DMRT) were performed to determine the effects of variables and determine the differences between mean values.
Results and discussion
Viscosity
Significant increases in viscosity were observed for all compound chocolate samples as crystal promoter concentration increased, as shown in . This suggests that viscosity is affected by the amount of crystal promoter added to the compound chocolate mixture, regardless of type. The increase in viscosity of compound chocolate formulated with CP1, a mixture of mono- and diglycerides (MDG) and polyglycerol esters, showed a similar trend to cake batter with added polyglycerol esters, an α-tending emulsifier present in CP1. In cake batter, increased viscosity is a result of decreased batter flow, which indicates increased air incorporation, thus increasing foam stability. Polyglycerol esters stabilize aqueous foam by forming an interfacial film around the air bubbles and preventing coalescence of fat dispersed in the water phase.[Citation22] The effect of polyglycerol esters in liquid cake shortening products are also related to their ability to form an interfacial film, as described by Wootton et al.[Citation23] In low-fat spreads, polyglycerol esters were observed to generate stable emulsions, and when combined with monoglycerides, they tended to stabilize water-in-oil systems better than with low-fat spread based on monoglycerides only.[Citation24] The benefits of the addition of MDG in low-fat spreads include fine distribution of water droplets in the fat phase, smooth spreading consistency, stable crystalline structure, and a pleasant melt-in-the-mouth sensation for the consumer.[Citation25] In the study by Vernier,[Citation7] addition of polyglycerol esters of unsaturated fatty acid esters to chocolate tended to increase viscosity, resulting in improved coverage of non-fat particles with more solvent from the continuous phase being used for solvation of the fatty acid chains. Apparent reduction in the amount of continuous phase available for the particles to flow leads to increased friction that leads to increased viscosity values.
Figure 1. Relationship between viscosity and crystal promoter concentration. CP: crystal promoter. The numbers that follow indicate additive type and concentration. CP1: mono- and diglycerides, polyglycerol esters; CP2: mono- and diglycerides, lecithin, citric acid esters; CP3: fully hydrogenated triglycerides.
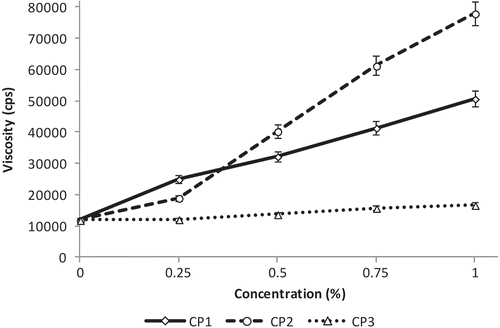
For CP2, the sharp increase in viscosity observed was partly a consequence of the CP2 additive increasing the amount of lecithin in the compound chocolate system. The addition of CP2, which was a blend of MDG, lecithin, and CITREM, increased lecithin content to more than the initially added concentration of 0.5% (control formulation). The results obtained were in contrast with the findings of Nebesny and Zyzelewicz,[Citation26] wherein an increase in lecithin content from 0.1 to 1.0% resulted in a drop in viscosity of milk and dark chocolates. Rector[Citation27] and Chevalley,[Citation28] however, suggested that too much lecithin (>0.5%) caused viscosity to increase in chocolate. This may be explained by the phase behavior of phospholipids and their tendency to form lamellar structures. Dhonsi and Stapley[Citation29] reported that lecithin migrates to sugar/fat interfaces and coats sugar crystals, aiding dispersion of sugar crystals in the continuous phase. When the sugar crystals are already adequately covered by phospholipids and lecithin is added in excess, the additional phospholipids do not simply dissolve in the fat phase but align with the other phospholipids to form lamellas. The second layer that is built on the “coated” crystals makes the outside of the entire structure more hydrophilic, which results in the increase in viscosity.[Citation30] In margarine and chocolate, CITREM are used as a substitute for lecithin.[Citation31] Because CITREM has properties similar to lecithin/PGPR combination,[Citation4] increasing its concentration by increasing CP2 could result in increased viscosity similar to the effect and mechanism of lecithin mentioned above. At higher concentrations (0.5–1%) CP2 was less effective than CP1 in lowering the viscosity of compound chocolates. The combined effects of lecithin, MDG and CITREM present in CP2 were more effective in reducing viscosity at a low concentration (0.25%) as compared to CP1. Chocolate formulations yielding higher viscosity values mean that they cannot be used for enrobing processes, which requires low viscosity to attain thinner chocolate coating. However, with application of mechanical vibrations, thicker chocolates could be utilized in molded and panned products, as well as in producing chocolate chips/drops.[Citation32]
The viscosities of the CP3 samples were closest to the control with only a gradual increase in values as additive concentration was increased suggesting minimal effect of the additive on viscosity. Oil viscosity depends on molecular structure and decreases with the unsaturation of fatty acids.[Citation33] In the study of Choi et al.,[Citation34] increasing the amount of canola oil blended with palm olein resulted in a minimal decrease in viscosity. But since the triglycerides (TAG) comprising CP3 are from fully hydrogenated canola oil, saturated fatty acids increased in the system as the additive was increased. Similar to the study of Talbot et al.,[Citation35] the increase of trisaturated TAG concentration resulted in increased viscosity. Saturated TAGs have rigid and fixed structures, and their presence in the compound chocolate mixture, while absent of double bonds, allowed close packing of molecules,[Citation36] consequently causing changes in viscosity.
Melting resistance
The images of compound chocolates after storage at 40°C are illustrated in . Complementary to these are the changes in the dimensions of the samples, which are listed in . The control sample that was incubated completely melted; that is, the sample was not able to retain its original shape after being subjected to the test temperature for 24 h. For CP1, it was only at 0.25% concentration in which the sample did not melt. At higher concentrations (0.5, 0.75, and 1.0%), CP1 addition resulted in significant height reductions coupled with length expansion, as well as shape deformation. Samples with CP2 were fairly stable at 40°C, with moderate changes in sample dimensions observed. For CP3, the sample containing 0.25% of the additive was the only one that melted, suggesting that the low amount of fully hydrogenated canola oil TAG present was not sufficient enough to form a synergistic effect with the CBR used. Non-melting products were produced with minimal addition of 0.5% CP3 and at higher concentrations. Oiling off was observed at the base of all the compound chocolate samples.
Table 3. Changes in sample dimensions illustrating resistance to melting.
Figure 2. Images of compound chocolates after storage at 40°C for 24 h. CP: crystal promoter. The numbers that follow indicate additive type and concentration. CP1: mono- and diglycerides, polyglycerol esters. CP2: mono- and diglycerides, lecithin, citric acid esters. CP3: fully hydrogenated triglycerides.
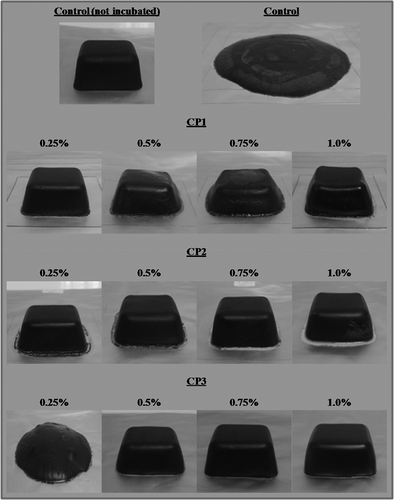
The type and concentration of crystal promoter additives present in the mixture affected the stability of compound chocolate in terms of resistance to melt. The oiling off observed suggests that the additives did not prevent the fat from melting but rather strengthened the chocolate network by preventing the product from collapsing even though the fat has partially melted. The strengthening of the network structure may have been influenced by the mesomorphic behavior of the polyglycerol esters in CP1 and the lecithin and CITREM in CP2 during the melt-mediated polymorphic phase transformation. In low water concentration environments, like chocolate, Dimick[Citation37] proposed that structural configurations of phospholipids and sterols and their esters could possibly form the inverse hexagonal HII mesophase orientation with trace amounts of water acting as the core. The polar head regions in the phospholipids and esters form very strong bonds with adjacent polar head groups, whereas the fatty acid hydrocarbon side chains associate through van der Waals forces.[Citation37] The effect of CP2 at increased concentrations was, however, observed to be better than that of CP1, possibly due to the synergistic interactions between lecithin, CITREM, and MDG present in CP2 and the other compound chocolate ingredients. Among the three crystal promoter additives, CP3 exhibited the best heat stability except at 0.25% concentration. Two possibilities as to the effect of CP3 at 0.25% concentration may have occurred. One is the formation of a monotectic, partial solid solution, wherein a slight amount of thermodynamic incompatibility between the two components became evident.[Citation38] Another is that a eutectic may have been formed between the TAG of CP3 and the TAG of the CBR. A monotectic occurs when the solid fat content of a mixture is less than that predicted by simple dilution alone, but still between that of the constituent fats.[Citation39] Eutectic effect, on the other hand, is a result of mixed crystals or co-crystallization in the fat continuous phase,[Citation40] wherein the solid fat content of the mixture containing the two different fats is lowered versus a simple summation of the contributions to the solid fat content of the two components.[Citation41] Either of the two phenomena happening would result in a softer, easily melted product. Increasing the concentration of CP3 effectively stabilized the network structure of the compound chocolates. According to Himawan et al.,[Citation42] increasing the difference between the melting temperatures of TAGs changes the phase behavior of the mixture from eutectic to monotectic. The reasons for this, however, are not known.[Citation43]
It was also observed that fat separation decreased with increase in additive concentration. This indicates that the compound chocolate network somehow minimized the flow out of molten fat by holding it within the compound chocolate structure of continuous fat phase and non-fat solid particles. To overcome the problem of oiling off at increased temperatures, Kempf and Downey[Citation44] suggested exposing the chocolate to a humid atmosphere at about 96% relative humidity (RH) and 29°C for approximately 2 min before cooling. This leads to the development of a thin sugar crust on the surface, similar when amorphous sugar is used as an ingredient.[Citation45]
Melting profile
The melting profiles of the different CP used, as well as, the compound chocolates formulated with these CP are shown in . In , the DSC melting profile of the CBR used is shown against the compound chocolate control sample. The DSC curve of CBR shows three melting peaks possibly corresponding to the different polymorphic forms associated with this type of fat. The first peak corresponds to the melting of the α polymorph followed by a two-step endothermic peak at higher temperatures corresponding to the melting of β’ and β polymorphs.[Citation38] A DSC curve can show two or more polymorphic forms that rearrange themselves with time to a more stable structure within those conditions.[Citation46] Once used to produce compound chocolate (Control), the CBR interacted with the rest of the ingredients used and generated a single endothermic peak. The single peak of the control suggests that the sample transitioned directly to the most stable crystal polymorph upon melting, which in this case is the β’ form associated with CBR crystallization. The DSC melting curves of the CP used are also shown in . The melting profile of CP1 shows three peaks all below 15°C, while CP2 and CP3 exhibit a single peak both at 59°C. Since CP1 and CP2 are mixtures of several emulsifiers it is difficult to presume the specific polymorphic form the DSC peaks represent. For CP1, peaks may correspond to α and β polymorphs of polyglycerol esters and MDG, respectively, which are the emulsifiers most stable crystal forms.[Citation22,Citation47] For CP2, while CITREM crystallizes in an α-like crystal form,[Citation48] its effect seems to be negligible compared to MDG, thus the DSC peak elucidated may correspond to the melting of β crystals. CP3 crystallization occurred in the β polymorph, the most stable crystal form of fully hydrogenated canola oil TAG.[Citation49]
Figure 3. DSC melting curves of A: cocoa butter replacer and crystal promoters used; B: compound chocolates formulated with CP1; C: compound chocolates formulated with CP2; and D: compound chocolates formulated with CP3. CP: crystal promoter. The numbers that follow indicate additive type. CP1: mono- and diglycerides, polyglycerol esters; CP2: mono- and diglycerides, lecithin, citric acid esters; CP3: Fully hydrogenated triglycerides; CBR: cocoa butter replacer.
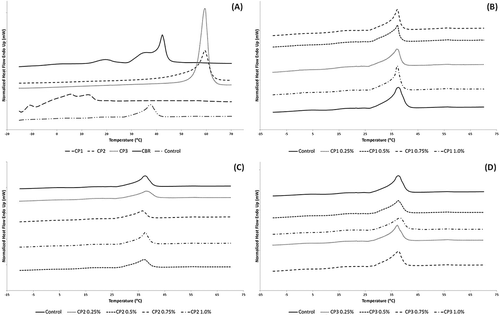
The compound chocolates formulated with different types and concentrations of CP exhibited similar distinct single peaks, with the peak temperatures differing slightly with each sample (–). According to McFarlane,[Citation50] peaks generated in DSC are observed in temperature ranges related to the melting of specific polymorphs, and in the case of this study, the peaks of the samples correspond to the stable β’ polymorph same as the control. Differences in peak shapes and widths indicate that varying the crystal promoter type and concentration added to compound chocolate produced changes in crystallinity and melting properties.
Peak onset (Tonset) corresponds to the temperature at which a particular crystal form starts to melt, while the end of melting (Tendset) signals the completion of liquefaction. The duration of melting is indicated by Tindex. The peak maximum (Tpeak) corresponds to the temperature at which melting rate is greatest. The melting enthalpy (ΔHM) is defined as the amount of energy needed to melt all of the fat. All these information are used to indicate crystal type.[Citation51] Peak height, position and resolution are dependent on sample composition and crystalline state distribution.[Citation50] shows values for the key DSC parameters of the compound chocolate samples analyzed.
Table 4. Melting properties of compound chocolate with CP1 additive at various concentrations.
Effects of CP1
In , the effects of adding CP1 in compound chocolates were illustrated by the DSC curves generated. The addition of CP1 at a range of concentrations resulted in steeper peaks than the control, with the peaks becoming broader as additive concentration increases. Compared to the control, the melting properties of the compound chocolates revealed significant differences (p < 0.05) in terms of Tindex and ΔHM, while only marginal changes in Tpeak, Tonset, and Tendset were observed (). Non-significant differences in Tpeak and Tonset imply that the maximum and starting temperature of melting are similar to the control and are independent of CP1 concentration especially for Tpeak. The Tendset tended to decrease compared with the control, suggesting that the compound chocolates with CP1 required slightly lower temperatures to complete melting. The Tindex and ΔHM also decreased compared with the control showing significant influence of CP1 addition on these parameters. Increasing CP1 concentration, however, caused a general increase in the Tindex and ΔHM of the samples, drawing near control values. This suggests that compound chocolates with higher CP1 concentrations had slower melting rates and required higher energies to complete melting. Results were in agreement with the findings of Sakamoto et al.,[Citation52] wherein polyglycerol-10 dodecabehenate added to palm mid fraction (PMF) at increasing amounts formed broader peaks with higher Tindex. Of the four concentrations of CP1 added to compound chocolate, it is at 0.5% concentration in which a shift in trend of DSC parameters tended to occur. And as noted in the results from melt resistance test, starting at 0.5% CP1 addition, compound chocolates were more prone to melting possibly as a result of changes in ΔHM. The low energy required to complete the melting of 0.25% CP1 was ideal in this case.
The effect of CP1 on crystallization of compound chocolates may be explained by the action of the polyglycerol esters present in the additive. According to Awad and Sato,[Citation53] polyglycerol esters are primarily absorbed at the interface of lipid systems as a thin film, which will crystallize prior to the bulk lipid of the oil droplet. The crystallized film will thus act as a nucleus in a heterogeneous nucleation and may promote the overall nucleation of the TAG present in the system. A similar mechanism may have taken place in the study by Sakamoto et al.,[Citation54] wherein the addition of polyglycerol fatty acid ester to palm oil affected the polymorphic crystallization and morphological properties of palm oil. Palm oil crystals with the addition of 1 wt. % of polyglycerol behenic acid esters promoted nucleation of palm oil, with β’ form preferentially crystallized. The additive may have crystallized faster than palm oil, inducing heterogeneous nucleation of palm oil as template.[Citation55]
Effects of CP2
The DSC curves in revealed the effect of CP2 addition on the crystallinity of the compound chocolate samples. Compared to the control, analysis of the DSC parameters showed significant differences (p < 0.05) amongst most of the samples in terms of Tindex and ΔHM (). The addition of CP2 had limited influences on temperatures for maximum, onset, and end melting as no significant differences were observed in terms of Tpeak, Tonset, and Tendset. It was, however, noted that Tonset tended to decrease compared with the control sample particularly at lower concentrations albeit insignificantly. In terms of ΔHM, values significantly decreased compared with the control. This implies that the compound chocolates with CP2 required lower enthalpies to melt than the control sample. The Tindex of the samples, on the other hand, significantly increased as opposed to the control especially at 0.25 and 0.5% concentrations. As the CP2 concentration increased, Tindex decreased moving toward the control. According to Afoakwa et al.,[Citation56] decreased Tindex suggests that chocolates containing lecithin, which is present in CP2, at lower concentrations might require relatively longer residence time to melt than those with higher lecithin levels, with likely impact on melting residence time of products during consumption. Also, the authors indicated that the lower melting index observed in products containing higher lecithin levels might be attributed to the sugar coating ability of lecithin during processing, and thus reducing their inter-particle interaction to induce chocolate melting properties. Thus, comparing the compound chocolate with 0.75% CP2 to the sample containing 0.25% CP2, the formers lower melting duration may be attributed to reductions in inter-particle interactions between CP2 components against each other as well as against the rest of the compound chocolate ingredients used. Of the compound chocolates containing CP2, the sample containing 0.5% concentration of the additive had the highest Tindex with the lowest Tonset, which in relation to melt resistance test results showed most notable changes in sample dimensions. The earlier commencement of melting and longer melting duration of this sample compared to the rest indicated a reduction in its melting stability.
Table 5. Melting properties of compound chocolate with CP2 additive at various concentrations.
When added to cocoa butter, lecithin had an impact on crystal growth and nucleation generating higher stable β polymorphs. In the case of this study which used CBR, stable β’ polymorphs may have been generated. Lecithin’s primary location is at the interface between fat and sugar particles, with their hydrophobic tail facing the fat phase.[Citation7] Based on the theory of heterogeneous nucleation, this creates a more favorable environment for fat crystals to evolve from, as compared to the hydrophilic sugar surface.[Citation57] Previous studies on phosphatidylcholine, a phospholipid constituting 15 wt. % of the total fat content in lecithin, have identified its seeding effect. Due to its tendency to form micelles in the HII mesophase, it provides an appropriate foundation for the nucleation of fat crystals.[Citation16] MDG present in CP2 may have also contributed to the initial nucleation during the crystallization process. Chaiseri and Dimick[Citation15] have reported that the presence of diglycerides high in stearic acid promoted fast nucleation of cocoa butter. In palm olein, the precise effect of diglycerides is dependent on their concentration and chemical structure. Dipalmitin and 1-palmitoyl-2-oleoyl- acylglycerol had positive and negative effects on the crystallization rate, respectively, while diolein showed a neutral effect.[Citation58] Similarly, unsaturated monoglycerides (from sunflower oil) do not impact the nucleation of crystals but the more saturated monoglycerides (derived from palm oil) promote nucleation.[Citation59] Both types of monoglycerides, however, accelerate the transformation from the α to the β′ polymorph.
Effects of CP3
The DSC curves of compound chocolates formulated with various concentrations of CP3 are shown in . Dissimilar peak shapes and sizes of the samples suggest differences in crystallinity between the products. The consequent melting parameters of the samples are listed in . Addition of CP3 caused significant changes (p < 0.05) in Tindex and ΔHM, with only minimal changes in terms of Tonset, Tpeak, and Tendset observed. ΔHM had a tendency to decrease with CP3 addition indicating that compound chocolates produced with CP3 would require lower energies to complete melting. In contrast, higher CP3 content tended to increase the duration of melting. The increase in Tindex indicates that compound chocolates with more CP3 additives would take relatively longer time to melt than less concentrated samples. Longer melting duration may be attributed to increased particle interactions between the TAG present in CP3 and those present in the CBR as a result of heterogeneity of the TAG. A shift in trend in DSC parameters tended to occur from absence of CP3 additive and at concentrations higher than 0.25% addition. In line with melt resistance results, the sample with 0.25% CP3 was predisposed to melting similar to the control due to the close DSC values obtained between them as compared to the rest of the compound chocolates. The lower Tindex coupled with higher ΔHM indicated a thermodynamic instability resulting to product meltdown.
Table 6. Melting properties of compound chocolate with CP3 additive at various concentrations.
The fully hydrogenated TAG in CP3 acted as crystallization seeds directing the crystallization in the compound chocolates. The binding of TAG molecules to a pre-existing crystal face is favored, without the energy requirement to create a crystal nucleus.[Citation60] The incorporation of CP3 promoted two effects associated with the control of crystallization, which are the availability of numerous additional nuclei and/or surfaces for crystal growth.[Citation61] In cocoa butter, crystallization seeds in the right crystal form are needed to take full advantage of seeding. For applications based on CBR and CBS, however, the type of crystallization seed is of less importance.[Citation62] In this study, the addition of CP3 may have further stabilized the β’ crystals of the compound chocolate products, accounting for the melting characteristics elucidated from DSC analysis.
Conclusions
The addition of CP to compound chocolates caused viscosity to increase as concentration increased. Whilst minimal changes were observed due to addition of CP3 compared with the control, extensive differences were observed for CP1 and CP2, with CP2 being more effective in reducing viscosity values than CP1 at a lower concentration (0.25%). In coating applications where compound chocolates are commonly used, lower viscosity values help achieve a constant small stream of flowing molten chocolate providing consistent thin coats. The addition of CP was also able to improve the heat stability of compound chocolates as indicated by the samples resistance to melting. Heat stability was achieved through the CP action of strengthening the compound chocolate network structure by preventing it from collapsing even though the fat has melted. The samples containing CP1 were fairly stable at 40°C but increased concentrations resulted in substantial changes in height and length dimensions along with shape deformations. All of the compound chocolates with CP2 showed rather strong resistance to melting. The same was true for the CP3 containing samples except at 0.25% concentration, which showed considerable melting after incubation. The improved heat stability would make it possible for compound chocolates to be marketed and consumed in countries with hot climates. At the same time, compound chocolates with CP would no longer be susceptible to melting and collapse during storage at high temperatures. The CP influenced to varying levels the degree of crystallinity and melting properties (Tindex and ΔHM) of the compound chocolates. Addition of CP1 resulted in decreased duration and enthalpy of melting. On the other hand, addition of CP2 and CP3 to compound chocolates resulted in decreased ΔHM and increased Tindex values. Neither CP1, CP2, nor CP3 influenced initiation (Tonset), end (Tendset), or maximum (Tpeak) temperatures to a large extent. All of the samples may have exhibited β’ polymorph crystallization, which is the most stable crystal form associated with CBR. Being aware of the physical properties of compound chocolates with added CP would enable the confectionery manufacturer to select the best option to use on the application or purpose desired. In addition, the results may provide information related to the sensory character of compound chocolate, which is an important quality parameter of the product that needs to be further investigated.
References
- Talbot, G. Introduction. In Science and Technology of Enrobed and Filled Chocolate, Confectionery, and Bakery Products; Talbot, G.; Ed.; Woodhead Publishing Limited: Cambridge, United Kingdom, 2009; 1–10.
- Smith, K. Ingredient Preparation: The Science of Tempering. In Science and Technology of Enrobed and Filled Chocolate, Confectionery, and Bakery Products; Talbot, G.; Ed.; Woodhead Publishing Limited: Cambridge, United Kingdom, 2009; 313–343.
- Talbot, G. Compound Coatings. In Science and Technology of Enrobed and Filled Chocolate, Confectionery, and Bakery Products; Talbot, G.; Ed.; Woodhead Publishing Limited: Cambridge, United Kingdom, 2009; 80–100.
- Beckett, S.T. Chocolate Flow Properties. In Industrial Chocolate Manufacture and Use, 4th Ed, Beckett, S.T.; Ed.; Blackwell Publishing Ltd.: Oxford, United Kingdom, 2009; 224–246.
- Goncalves, E.V.; Lannes, S. Chocolate Rheology. Food Science and Technology (Campinas) 2010, 30 (4), 845–851.
- Afoakwa, E.; Paterson, A.; Fowler, M. Factors Influencing Rheological and Textural Qualities in Chocolate—A Review. Trends in Food Science and Technology 2007, 18, 290–298.
- Vernier, F.C. Influence of Emulsifiers on the Rheology of Chocolate and Suspensions of Cocoa or Sugar Particles in Oil. Ph.D. dissertation, University of Reading, September 1997.
- Wells, M. Controlling the Rheology of Chocolate and Fillings. In Science and Technology of Enrobed and Filled Chocolate, Confectionery, and Bakery Products; Talbot, G.; Ed.; Woodhead Publishing Limited: Cambridge, United Kingdom, 2009; 255–284.
- Ackar, D.; Skrabal, S.; Subaric, D.; Babic, J.; Milicevic, B.; Jozinovic, A. Rheological Properties of Milk Chocolates As Influenced by Powder Type, Emulsifier, and Cocoa Butter Equivalent Additions. International Journal of Food Properties 2015, 18, 1568–1574.
- Moran, D.P.J. The Influence of Some Surfactants on the Viscosity of Confectionery Fat/Sugar Mixtures. Revue Internationale du Chocolat 1969, 24 (12), 478–482.
- Musser, J.C. The Use of Monoglycerides in Chocolate and Confectionery Coatings. Proceedings of the 34th Annual PMCA Production Conference, Lancaster, Pennsylvania, April 22–24, 1980; Drexel Hill, Pennsylvania, 1980; 51–59.
- Hogenbirk, G.Viscosity and Yield Value for Chocolate and Coatings—What They Are and How to Influence Them. Confectionery Production 1988, 8, 456–478.
- Aronhime, J.S.; Sarig, S.; Garti, N. Mechanistic Considerations of Polymorphic Transformations of Tristearin in the Presence of Emulsifiers. Journal of the American Oil Chemists’ Society 1987, 64, 529–533.
- Aronhime, J.; Sarig, S.; Garti, N. Emulsifiers as Additives in Fats: Effect on Polymorphic Transformations and Crystal Properties of Fatty Acids and Triglycerides. Food Structure 1990, 9, 337–352.
- Chaiseri S.; Dimick, P. Dynamic Crystallization of Cocoa Butter. II. Morphological, Thermal, and Chemical Characteristics During Crystal Growth. Journal of the American Oil Chemists’ Society 1995, 72, 1497–1503.
- Savage, C.M.; Dimick, P.S. Influence of Phospholipids During Crystallization of Hard and Soft Cocoa Butters. The Manufacturing Confectioner 1995, 75, 127–132.
- Loisel, C.; Lecq, G.; Keller, G.; Ollivon, M. Dynamic Crystallization of Dark Chocolate As Affected by Temperature and Lipid Additives. Journal of Food Science 1998, 63, 73–79.
- Weyland, M.; Hartel, R. Emulsifiers in Confectionery. In Food Emulsifiers and Their Applications, 2nd Ed; Hasenhuettl, G.L.; Hartel, R.W.; Eds.; LLC Springer Science—Business Media: New York, NY, 2008; 285–305.
- Alander, J.; Warnheim, T.; Luhti, E. Heat-Resistant Chocolate Composition and Process for the Preparation Thereof. US Patent 5,486,376, January 23, 1996.
- Tisoncik, M.A. Impact of Emulsifiers on Physical, Sensory, and Microstructural Properties in Formulated Dark Chocolate with an Innovative Educational Approach; M.S. dissertation, University of Illinois, May 18, 2010.
- Vasanthan, T.; Batty, R.S. Physicochemical Properties of Small and Large Granule Starches of Waxy, Regular, and High Amylase Barleys. Cereal Chemistry 1996, 73, 199–207.
- Norn, V. Polyglycerol Esters. In Emulsifiers in Food Technology, 2nd Ed.; Norn, V.; Ed.; John Wiley and Sons, Ltd.: Oxford, United Kingdom, 2015; 182–208.
- Wotton, J.C.; Howard, N.B.; Martin, J.B.; McOsker, D.E.; Holme, J. The Role of Emulsifiers in the Incorporation of Air into Layer Cake Batter Systems. Cereal Chemistry 1967, 44, 333–343.
- Anonymous. Low Fat Spread. Research Disclosure No. 32977, 1991, 689–691.
- Moonen, H.; Bas, H. Mono- and Diglycerides. In Emulsifiers in Food Technology; Whitehurst, R., Ed.; Blackwell Publishing Ltd.: Oxford, United Kingdom, 2004; 40–58.
- Nebesny, E.; Zyzelewicz, D. Effect of Lecithin Concentration on Properties Of Sucrose-Free Chocolate Masses Sweetened with Isomalt. European Food Research and Technology 2005, 220, 131–135.
- Rector, D. Chocolate—Controlling the Flow. Benefits of Polyglycerol Polyricinoleic Acid. The Manufacturing Confectioner 2000, 80 (5), 63–70.
- Chevalley, J. Chocolate Flow Properties. In Industrial Chocolate Manufacture and Use, 3rd Ed; Beckett, S.T.; Ed.; Blackwell Science: Oxford, United Kingdom, 1999; 182–200.
- Dhonsi, D.; Stapley, A.G.F. The Effect of Shear Rate, Temperature, Sugar, and Emulsifier on the Tempering of Cocoa Butter. Journal of Food Engineering 2006, 77, 936–942.
- Bueschelberger, H.-G. Lecithins. In Emulsifiers in Food Technology, Whitehurst, R.J.; Ed.; Blackwell Publishing Ltd.: Oxford, United Kingdom, 2004; 1–39.
- Gaupp, R.; Adams, W. Acid Esters of Mono- and Diglycerides. In Emulsifiers in Food Technology; Whitehurst, R.J.; Ed.; Blackwell Publishing Ltd.: Oxford, United Kingdom, 2004; 59–85.
- Afoakwa, E. Chocolate Science and Technology; Wiley-Blackwell: York, United Kingdom, 2010; 296 pp.
- Kim, J.; Kim, D.N.; Lee, S.H.; Yoo, S.-H.; Lee, S. Correlation of Fatty Acid Composition of Vegetable Oils with Rheological Behavior and Oil Uptake. Food Chemistry 2010, 118, 398–402.
- Choi, H.; Lee, E., Lee, K. Quality Evaluation of Noble Mixed Oil Blended with Palm and Canola Oil. Journal of Oleo Science 2014, 63, 653–660.
- Talbot, G.; Smith, K.; Zand, I. Effects of Trisaturated Triglycerides on Chocolate Rheology. In Loders Croklaan Articles and Lectures Booklet; Loders Croklaan: Wormerveer, The Netherlands, 2006; 26–35.
- Sharoba, A.M.; Ramadan, M.F. Impact of Frying on Fatty Acid Profile and Rheological Behavior of Some Vegetable Oils. Journal Food Processing and Technology 2012, 3 (7), 161–169.
- Dimick, P.S.; Influence de la Composition Sur la Cristallisation Du Beurre de Cacao [Influence of the Composition on the Crystallization of Cocoa Butter]. In La Cristallization, 3ème Colluge; Paris, 1994; 33–46.
- Timms, R.E. Phase Behavior of Fats and Their Mixtures. Progress in Lipid Research 1984, 4, 23–38.
- Ziegler, G. Product Design and Shelf Life Issues: Oil Migration and Fat Bloom. In Science and Technology of Enrobed and Filled Chocolate, Confectionery, and Bakery Products; Talbot, G.; Ed.; Woodhead Publishing Limited: Cambridge, United Kingdom, 2009; 185–210.
- Windhab, E.J. Tempering. In Industrial Chocolate Manufacture and Use, 4th Ed; Beckett, S.T.; Ed.; Blackwell Publishing Ltd.: Oxford, United Kingdom, 2009; 276–319.
- Haylock, S.J.; Dodds, T.M. Ingredients from Milk. In Industrial Chocolate Manufacture and Use, 3rd Ed; Beckett, S.T.; Ed.; Blackwell Science: Oxford, United Kingdom, 1999; 137–152.
- Himawan, C.; Starov, V.M.; Stapley, A.G.F. Thermodynamic and Kinetic Aspects of Fat Crystallization. Advances in Colloid and Interface Science 2006, 122, 3–33.
- Takeuchi, M.; Ueno, S.; Sato, K. Synchrotron Radiation SAXS/WAXS Study of Polymorph-Dependent Phase Behavior of Binary Mixtures of Saturated Monoacid Triacylglycerols. Crystal Growth and Design 2003, 3, 369–374.
- Kempf, N.W.; Downey, P.J. Finished Chocolate Product. US Patent 2,760,867, August 28, 1956.
- O’Rourke, J.J. Chocolate Product and Process. US Patent 2,904,438 , September 15, 1959.
- Schenk, H.; Peschar, R. Understanding the Structure of Chocolate. Radiation Physics and Chemistry 2004, 71, 829–835.
- Foubert, I.; Dewettinck, K., Van de Walle, D.; Dijkstra, A.J.; Quinn, P.J. Physical Properties: Structural and Physical Characteristics. In The Lipid Handbook with CD-ROM, 3rd Ed; Gunstone, F.D.; Harwood, J.L.; Dijkstra, A.J.; Eds.; CRC Press: Boca Raton, FL, 2007; 471–534.
- Young, F.V.K.; Poot, C.; Biernoth, E.; Krog, N.; Davidson, N.G.J.; Gunstone, F.D. Processing of Fats and Oils. In The Lipid Handbook, 2nd Ed; Gunstone, F.D.; Harwood, J.L.; Padley, F.B., Eds.; Chapman and Hall: Cambridge, United Kingdom, 1994; 249–318.
- Przybylski, R. Canola/Rapeseed Oil. In Vegetable Oils in Food Technology: Composition, Properties, and Uses, 2nd Ed; Gunstone, F.D.; Ed.; Blackwell Publishing Ltd.: Oxford, United Kingdom, 2011; 107–136.
- McFarlane, I. Instrumentation. In Industrial Chocolate Manufacture and Use, 3rd Ed; Beckett, S.T.; Ed.; Blackwell Science: Oxford, United Kingdom, 1999; 347–376.
- Loser, U. Instrumentation. In Industrial Chocolate Manufacture and Use, 4th Ed; Beckett, S.T.; Ed.; Wiley-Blackwell Publishing Ltd.: Oxford, United Kingdom, 2009; 493–529.
- Sakamoto, M.; Ohba, A.; Kuriyama, J.; Maruo, K.; Ueno, S.; Sato, K. Influences of Fatty Acid Moiety and Esterification of Polyglycerol Fatty Acid Esters on the Crystallization of Palm Mid Fraction in Oil-in-Water Emulsion. Colloids and Surfaces B: Biointerfaces 2004, 37, 27–33.
- Awad, T.S.; Sato, K. Fat Crystallisation in O/W Emulsions Controlled by Hydrophobic Emulsifier Additives. In Physical Properties of Lipids, Marangoni, A.; Narine, S.S.; Eds.; Marcel Dekker: New York, NY, 2002; 3–62.
- Sakamoto, M.; Maruo, K.; Kuriyama, J.; Kouno, M.; Sato, K. Effects of Adding Polyglycerol Behenic Acid Esters on the Crystallization of Palm Oil. Journal of Oleo Science 2003, 52, 639–645.
- Sato, K.; Ueno, S. Polymorphism in Fats and Oils. In Bailey’s Industrial Oil and Fat Products, 6th Ed.; Shahidi, F., Ed.; Wiley-Interscience: New York, NY, 2005; 77–120.
- Afoakwa, E.; Paterson, A.; Fowler, M.; Vieira, J. Characterization of Melting Properties of Dark Chocolates from Varying Particle Size Distribution and Composition Using Differential Scanning Calorimetry. Food Research International 2008, 41, 751–757.
- Svanberg, L.; Ahrne, L.; Loren, N.; Windhab, E. Effect of Sugar, Cocoa Particles, and Lecithin on Cocoa Butter Crystallisation in Seeded and Non-Seeded Chocolate Model Systems. Journal of Food Engineering 2011, 104, 70–80.
- Long, K.; Jamari, M.A.; Ishak, A.; Yeok, L.J.; Latif, R.A.; Lai, O.M. Physico‐Chemical Properties of Palm Olein Fractions as a Function of Diglyceride Content in the Starting Material. European Journal of Lipid Science and Technology 2005, 107 (10), 754–761.
- Fredrick, E.; Foubert, I.; Van de Sype, J. Dewettinck, K. Influence of Monoglycerides on the Crystallization Behavior of Palm Oil. Crystal Growth and Design 2008, 8, 1833–1839.
- Lonchampt, P.; Hartel, R.W. Fat Bloom in Chocolate and Compound Coatings. European Journal of Lipid Science and Technology 2004, 106, 241–274.
- Ribeiro, A.; Masuchi, M.; Miyasaki, E.; Domingues, M.; Stroppa, V.; de Oliveira, G.; Kieckbusch, T. Crystallization Modifiers in Lipid Systems. Journal of Food Science and Technology 2015, 52, 3925–3946.
- Norberg, S. Chocolate and Confectionery Fats. In Modifying Lipids for Use in Food, Gunstone, F.; Ed; Woodhead Publishing Ltd.: Cambridge, United Kingdom, 2006; 488–516.