ABSTRACT
The macaque (Macaca fascicularis) monkeys are the third largest primate population in tropical forests. Being the potential carrier of Simian Immunodeficiency and Ebola and Corona viruses, as well as being a religiously-protected and wildlife-protected species could not save the macaques from being over-hunted and consumed. Despite having needs, methods to detect monkey species in foods are rarely documented. To fill this gap, this article describes a monkey-specific polymerase chain reaction assay targeting a short-site (120 bp) of mitochondrial d-loop gene because the short-length targets are thermodynamically more stable than the longer ones under degrading states. Specificity was tested against 17 terrestrial and aquatic meat and fish species and no cross-species amplification was detected under raw, processed, and admixed states. The sensitivity of the assay was 0.0001 ng DNA under pure states and 0.1% monkey meats in binary meat mixtures. Finally, the assay was validated by digesting the polymerase chain reaction products with AluI and CViKI-1 and distinctive restriction fingerprints for macaque species were demonstrated.
Introduction
Fraud labeling of meat and meat products is prevalent in most countries. For instance, pig, horse, and buffalo meats are frequently frauded as beef and chevon.[Citation1] However, the adulteration reports of some uncommon and detrimental species such as rat, dog, and cat species in common meats are alarming and, indeed, have raised widespread concern.[Citation2] Recently, the illegal trades of certain endangered populations including primates, carnivores, ungulates, and wild fowl have been spotted.[Citation3] Although humans have hunted and eaten wild meat for millennia for health benefits and exotic taste, consumption over recent years has dramatically increased.[Citation4] The recent trend of meat preference shows a higher interest in wild meat over red meat due to nutritional facts, such as lower content total dietary fat and saturated fat.[Citation5] Wild meat has been a great appeal for exotic meat lovers because of the exquisite tastes and healthier attributes in terms of lower fat (<3%) and high protein contents (16–55%).[Citation6] In many parts of Africa, bush meat has been consumed, and the total consumption has been estimated to be 3.8 million tonnes of primate meat. For intance, it has been estimated that the exploitation of primates in the Tai region of the Ivory Coast represents a market value of $124,031–$136,688 per annum.[Citation7] Meanwhile in Southeast Asia, Malaysia is one of the most intense hunting countries, where approximately 108 million tonnes of bushmeat are killed for consumption annually.[Citation8,Citation9]
Despite being the common meal by the rural people in Africa and Southeast Asia, the possibility of primate meat to be replaced in food products is relatively high. This is due to the huge population of primate species, such as long-tailed monkey, Macaca fascicularis. It is the third largest primate species broadly distributed across the mainland and islands of Southeast Asia.[Citation10] In 2010, an adulteration case of long-tailed monkey meat has been reported in Indonesia, where the meat was replaced for beef in meatball soup.[Citation11] Even though there was a lack of offical documentation on this issue, we believe the monkey species are certainly a potential candidate to be adulterated in foods in certain areas where its population density is very high and could be easily hunted, but there is no offered price in the legal markets.
On the other hand, bushmeats are often carriers of many emerging zoonotic diseases.[Citation12] For intance, the Ebola virus is linked with the consumption of bushmeat, mainly chimpanzees, that were found dead in western Africa.[Citation13] Morever, trichinellosis has long been associated with consumption of undercooked meat from wild animals such as bears. Furthermore, the recent emergence of severe hepatitis E in Japanese hunters is linked to the consumption of uncooked meat from deer and wild boar.[Citation14] These exotic foods are definitely carriers of several zoonotic pathogens or parasites, such as protozoa (Toxoplasma), trematodes (Fasciola sp., Paragonimus spp.), cestodes (Taenia spp., Diphyllobothrium sp.), and nematodes (Trichinella spp., Anisakis sp., Parastrongylus spp.).[Citation14] Therefore, the need to develop a reliable and convenient assay for M. fascicularis identification is highly desirable.
Enzyme-linked immunosorbent assay (ELISA),[Citation15] liquid chromatography,[Citation16] species-specific polymerase chain reaction (PCR),[Citation17] multiplex PCR,[Citation18] randomly amplified polymorphic DNA (RAPD),[Citation19] PCR restriction fragment length polymorphism (RFLP), real-time PCR,[Citation20] and nanoparticle biosensors[Citation21,Citation22] have been proposed for the identification of meat species. Among all these detection techniques, DNA-based PCR methods are the method of choice because of the universal presence of DNA in all cells, tissues, and organs, as well as the possiblity of amplifying a single copy DNA in specimens into sufficient quanties using PCR techniques.Therefore, we developed a very short-amplicon-length (120 bp) PCR assay targeting the mitochondrial d-loop gene which is present in multiple copies. We further validated the assay results by RFLP analysis for the authentic detection of macaque monkeys in raw, processed, and admixed matrices. Selection of a shorter target is advantageous since it can survive under compromised states (natural decomposition or forceful breakdown of DNA).[Citation23]
Materials and Methods
Samples Collections
Fresh commercial meat, fish, and other samples were purchased in triplicate from various markets across Malaysia (). All samples were chopped into the smallest possible pieces using surgical blades and were stored in a freezer at –20°C until further use to prevent the enzymatic degradation of DNA.
Table 1. Description of meat, fish, and other samples used in the study.
Binary Admixed Preparation
Two types of binary mixtures, namely (1) beef-monkey and (2) goat-mixtures were made into a 100 g specimen by spiking monkey meat into beef and goat meat at a proportion of 10, 5, 1, and 0.1%. All meat mixtures were first minced and then homogenised with a blender. To avoid contamination, each mixture was prepared separately using freshly cleaned blender containers on three different days.
DNA Extraction
Total DNA extraction using 30 mg of each raw and admixed sample was performed using DNA extraction kit for animal tissues (Yeastern Biotech Co. Ltd, Taipei, Malaysia) as per manufacturer’s instruction provided with the kit. The DNA concentration and purity were checked by an ultraviolet-visible (UV-VIS) spectrophotometer (Biochrom Libra S70, Biochrom Ltd., UK) taking absorbance at 260–280 nm. The purified DNA was kept at –20°C until further use.
Monkey-Specific Primer Design
A pair of M. fascicularis primers were designed targeting mt-d-loop gene (FJ906803.1) available in NCBI database using Primer3Plus (http://www.bioinformatics.nl/cgi-bin/primer3plus/primer3plus.cgi) software. The specificity of primers was ensured though mismatch, phylogenetic tree, 3D plot, and Basic Local Alignment Search Tool (BLAST) analyses.[Citation24] The primers (forward primer: 5’ TGAAATCAATATCCCGCACA3’and reverse primer: 5’ CTGGTTGTTATGGCCC TGAG 3’) were supplied by the First Base Laboratories Sdn. Bhd., Selangor, Malaysia.
PCR Amplification
We prepared a PCR reaction mixture in a 250 µL PCR tubes in a total reaction volume of 20 µL containing 4 µL of 5× Green GoTaq Flexi Buffer, 2.2 µL of 25 mM of MgCl2, 0.4 µL of 0.2 mM of each DNTP, 0.4 µM of each primers and 0.5 unit Taq polymerase and 10 ng of total DNA extracted from each sample. For assay validation, we prepared another type of reaction that consisted of 0.4 µM of Eukaryotic 18S rRNA as endogenous control (Forward primer: 5’-GGT AGT GAC GAA AAATAA CAA TAC AGG AC-3’ and reverse primer: 5’-ATA CGC TAT TGG AGC TGG AAT TAC C - 3’; ).[Citation25,Citation26] We purchased all PCR reagents from Promega Corporation (Madison, USA) and performed PCR reaction in Veriti 96-Well Thermal Cycler (Applied Biosystems Inc., CA, USA), following initial denaturation at 94°C for three min followed by 30 cycles of denaturation at 94°C for 30 s, annealing at 58°C for 30 s, extension at 72°C for one min, and the final extension at 72°C for five min. We stored PCR products at –20°C for further analysis and set a negative control for the PCR reaction with nuclease-free water instead of template DNA to eliminate contamination. Amplified PCR products were analysed by gel electrophoresis at 120 V for 90 min in 2% agarose gel with Fluorosafe DNA Stain (First Base Laboratories, Selangor, Malaysia). The gel was visualized in a gel-imager (AlphaImager HP; Alpha Innotech Corp., Santa Clara, CA, USA).
Figure 1. Specificity analysis of macaque-specific primer pair against various primates and non-primates species. Shown are gel image of 18 meat species A: phylogenetic tree of 13 Macaca (Genera Cercopithecinae; 1–13), 20 Colobines species (Genera Presbytis, Trachypithecus, Semnopithecus, Rhinopithecus, Nasalis, Pygathrix, Colobus, and Procolobus; 14–33), and Homo sapiens (34) and 3D plot showing the discrimination of long-tailed Macaque facicularis target in the primer binding sites of 51 species (c). In A: Lane L: 100 bp Ladder; Lane 1–18: 120 bp PCR products from 10 ng DNA template extracted from monkey, chicken, beef, chevon, lamb, buffalo, venison, duck, pork, quail, pigeon, salmon, carp, cod, turtle, dog, cat, and rat meats, respectively. Lane 19: negative control.
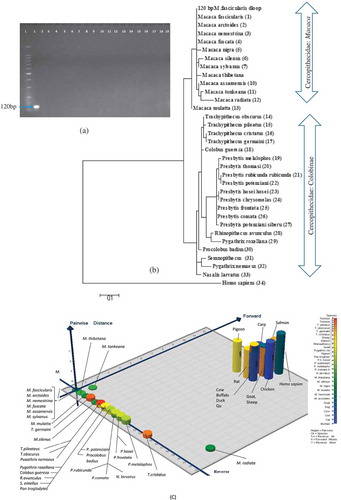
Figure 2. Sensitivity test under A: pure and B: monkey-beef, and C: monkey-goat binary admixtures under raw states.
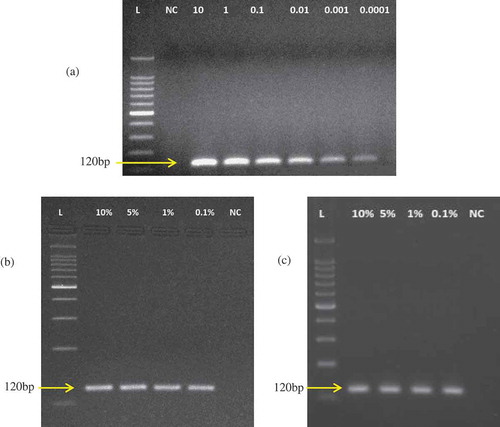
Figure 3. Marker DNA stability test under A: boiling, B: microwave cooking, and C: autoclaving treatments. In (A), lanes 1–6, 7–12, and 13–18 represent boiling at 60, 80, and 100°C, respectively, for 30 min. In (B), lanes 1–6, 7–12, and 13–18 represent microwave cooking at 300, 500, and 700 W, respectively, for 30 min. In (C), lanes 1–6, 7–12, and 13–18 represent autoclaving at 121°C for 30, 90, and 150 min, respectively. The six set of lanes in every treatment demonstrate PCR products from 10, 1, 0.1, 0.01, 0.001, and 0.0001 ng template DNA extracted from post-treated meats.
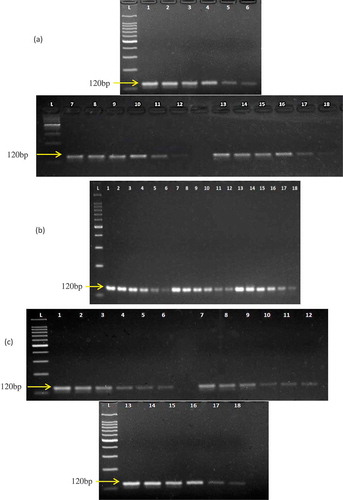
Figure 4. Restriction pattern analysis of monkey-target (120 bp) and endogenous control (141 bp) before (Lanes 1–3) and after AluI (Lane 4–6) and CViKI-I digestion. Lanes 1, 4, and 7: monkey; Lanes 2, 5, and 8: cow; and Lanes 3, 6, and 9: goat. Endogenous target (141 bp) was amplified from all species, reflecting good quality templates in all tubes and eliminating any false negative detection.
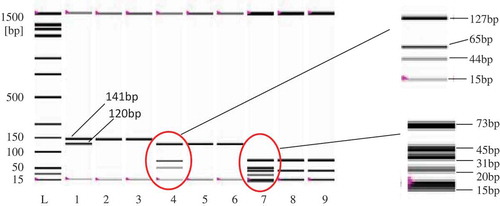
Specificity and Sensitivity Test
We tested the specificity of the primer pair via a cross-amplifying reaction with DNA of 17 non-target species (chicken, beef, chevon, lamb, buffalo, venison, duck, pork, quail, pigeon, salmon, carp, cod, turtle, dog, cat, and turtle). The sensitivity was determined by dilution of DNA extracted from pure and mixed meat (10, 5, 1, and 0.1% monkey meats mixed with adjusted amount of beef and goat). The limit of detection (LOD) was determined by amplification of 10-fold serially diluted (10, 1, 0.1, 0.01, 0.001, and 0.0001 ng DNA) monkey DNA.
Stability Test
Collected raw monkey meat samples were randomly chopped into the smallest possible pieces using a surgical blade. Three types of food processing and cooking treatments were applied to the chopped meat samples to evaluate the stability of d-loop gene target under processing conditions. To mimic the normal way of cooking, approximately 50 g of chopped monkey meat was boiled at 60, 80, and 100°C for 30 min and microwave cooked at 300, 500, and 700 Watts for 30 min. To simulate the steaming and canning process, meat samples were autoclaved at 121°C under 14.5 psi for 30, 60, and 150 min. After completing the heat treatments, total genomic DNA was extracted separately from 100 mg of treated samples using Yeastern Genomic DNA Mini Kit (Yeastern Biotech Co., Ltd. Taipei, Taiwan) as per the protocol provided by the manufacturer. Finally, concentration and purity of DNA were checked by UV-VIS spectrophotometer (Biochrom Libra S70, Biochrom Ltd., UK) based on absorbance value of 1.7–2.0 at A260/280 nm. More DNA was yielded from heat treated samples but only 10 ng was used for PCR amplification. The purified DNA was kept at –20°C until used.
RFLP Aanalysis
PCR products were digested with AluI and CViKI-1 restriction endonucleases (New England Biolabs, UK) in 25 µL reaction mixture in separate tubes containing 1 µg of unpurified PCR product, 5 U of enzyme, 1× digestion buffer and adjusted amount of sterilized distilled water. Digestion was carried out at 37°C in a shaking water bath for 45 min. After 45 min, AluI digestion was stopped by heating the mixture at 65°C for 20 min. However, no enzymatic inactivation steps were required for the CViKI-1 enzyme. For RFLP analysis, 1 µL of digested product was applied to a microfluidic-lab-on-a-chip using 1 k DNA kit and was separated by Experion Automated Electrophoresis System (Bio-Rad, CA, US).
Result and Discussion
DNA Extraction
DNA was extracted from raw, boiled, microwaved, and autoclaved meat samples. Heat treated samples provided a better yield of DNA (150–587 ng/µL for boiled and 600–700 ng/µL for autoclaved and microwaved samples) than the raw meat samples (100–200 ng/µL). The higher DNA yield upon thermal processing of meat samples might be due to the dehydration process, which results in an increased number of cells per unit weight of the sample.[Citation27]
Specificity Test
Species-specific PCR are often conclusive and have been widely used for the detection of beef,[Citation28] chicken,[Citation29] pork, and dog[Citation2,Citation25] in singleplex and cattle, pig, chicken, sheep, goat, and horse[Citation18] in multiplex PCR systems. Although the latter allows for amplifying and detecting multiple targets at a time, reducing analytical cost and time, its optimization is tedious and it is often difficult to achieve optimum PCR conditions with multiple target-species with uniform sensitivity. In contrast, singleplex PCR is easy, robust, accurate, and highly sensitive to detect a single target.[Citation25]
In-silico analyses of M. facsicularis (FJ906803.1) d-loop gene target (120 bp) against 17 non-target species revealed perfect matching (100%) only with M. fascicularis and 10–13 nt mismatching with the other species, demonstrating sufficient fingerprints for the target species ().[Citation25] Pairwise distance () and phylogenetic tree () among the closely related species were analysed as described elsewhere.[Citation24,Citation30] The lowest distance was observed between monkey and buffalo, carp, dog, cat, rat, and pig (1.43–1.44), and the highest was found between monkey and chicken (1.86), indicating a high genetic distance and unlikelihood of cross-species amplification in a PCR run. The mismatched-bases in the primer binding sites of the studied species were between 50–70% which made the cross-species detection improbable.[Citation22,Citation25,Citation31,Citation32] The dendrogram () constructed by a neighbour-joining method[Citation2] clearly reflected high discrimination of M. facicularis from other animal species. Finally, the PCR run amplified only the M. fascicularis species (), confirming the theoretical findings.
Table 2. The mismatch comparison of the long-tailed macaque specific forward and reverse primers against 27 species including 9 other macaque species.
Table 3. Pairwise distances of the Macaca fascicularis specific primer sites of D-loop gene against corresponding sites of 32 different tested species and most closely related species.
The literature report reflected four monkey-specific PCR assays were previously used for the phylogenetic studies of monkey species. However, the target amplicons of the documented assays were very large (cytochrome c, 850 bp),[Citation33] (cytochrome b, 383 bp),[Citation34] (mt-whole genome, 896bp),[Citation35] and (d-loop, 590 bp)[Citation36] easily break down under food processing treatments and thus are not suitable for meat-species detection in foods.[Citation2,Citation25] Thus, a very short-amplicon-length (120-bp) PCR assay targeting mt-d-loop gene was reported for the first time for M. fascicularis detection in processed meat.
Sensitivity Test
PCR sensitivity for monkey detection was tested for the first time using two different approaches. First, serially diluted (10, 1, 0.1, 0.01, 0.001, and 0.0001 ng) DNA extracted from pure raw meat was used in triplicate (three independent analysts) on three different days to determine the sensitivity under raw and pure states. Previously this method was used by Che Man et al.[Citation37] and Karabasanavar et al.[Citation38] to their assay sensitivity for porcine at 0.001 ng/µL level. In the current study, 120 bp products was clearly observed from 0.0001 ng macaque DNA template, and therefore, the LOD for this assay was defined as 0.0001 ng macaque DNA under raw and pure states (). However, the LOD was 0.001 ng DNA extracted from boiled, microwaved, and autoclaved macaque DNA (). This higher LOD in processed meat was referred to as the breakdown of DNA under processing treatments. Second, to simulate the real process of meat adulteration, base adulterated meat mixture (BAM) was used.[Citation25] shows PCR products from both monkey-beef and monkey-goat binary admixture and demonstrates that macaque-specific PCR assay developed in this study was highly sensitive since it identified as low as 0.1% (w/w) monkey meat under mixed background. The intensity of the PCR product obtained from 0.1% monkey admixed suggested that the assay could detect much lower than 0.1% level of adulteration. Contamination as low as 1% is routinely detected and any amounts less than 0.1% is considered unprofitable in commercial settings weighing the risk of good will damage.[Citation39] Ali et al.[Citation25] and Hazim et al.[Citation40] detected up to 0.01 and 0.1% pork in the meat mixture, respectively. However, the intentional adulteration is considered above 5% and inadvertent contamination is categorized if it is in the range of 0.1 to 1%.[Citation41] Therefore, the sensitivity of this assay was appropriate for detection of both profit making adulteration and unintentional contamination of monkey species in meat products.
Target DNA Stability
demonstrates that DNA extracted from all the heat-treated samples was successfully amplified by PCR. Boiling of meat samples at 60, 80, and 100°C for 30 min did not affect the amplification of species-specific biomarkers from M. fascicularis (). In standard domestic practice, microwave cooking is performed at 500 Watts (W) for 15–30 min. The meat was cooked at three different conditions; low (300 W), medium (500 W), and extreme (700 W) microwaving and PCR products were from 10–0.001 ng template DNA (). Meat cooked above 700 W for 30 min appeared to be dried out, burnt, and thus not suitable for consumption (data not shown). Arslan et al.[Citation19] pan fried beef at 190°C for 80 min and found no PCR product at this regime when cooking was performed under non-aqueous conditions. However, the DNA extracted in the present assay was sufficiently amplified up to 700 W, microwaving for 30 min (), reflecting the target-stability under extreme conditions.
Previously, Haunshi et al.,[Citation29] Karabasanavar et al.,[Citation27] and Mane et al.[Citation43] studied the effect of autoclaving on DNA degradation by treating various types of domestic meat at 121°C for 15–30 min and they found their samples were stable and were not degraded at this condition. Here, we autoclaved monkey meat at 120°C for 30, 90, and 150 min (extensive treatment) and found PCR products under all conditions. However, faded bands were obtained from 0.01 and 0.001 ng template under extreme autoclaving (Lanes 17 and 18 in ), reflecting some degree of target breakdown under extreme treatments. However, it did not affect the identification. This finding was in line with the established fact that shorter DNA targets are extremely stable under extreme processing treatments.[Citation2]
PCR-RFLP Analysis
Occasionally, the end-point PCR assay could be unconvincing since it only shows the virtual data with a lack of sequence information. This limitation, however, could be overcome by other complementing analytical techniques, such as probe hybridisation, DNA sequencing, and restriction analysis (RFLP).[Citation44] In this work, we validated our assay by using PCR-RFLP analysis, since the amplicon size was small with adequate restriction sites but was short for DNA sequencing, which requires larger sequences. RFLP also needs a simple instrument and could be done in ordinary lab settings. We digested the 120 bp monkey-specific PCR products by two different enzymes, AluI and CViKI-1 since in silico analysis showed available restriction sites for these enzymes with suitable fragment-lengths (New England Biolabs, http://nc2.neb.com/NEBcutter2/). Two-sites for AluI and 4 sites for CViKI-1 were found within the amplified sequence (120 bp). Lane 4 in demonstrates two fragments at the length of 65 and 44 bp which resulted by following the AluI digestion of the monkey-specific PCR product (120bp). However, a 11 bp fragment, which was below the resolution capacity of the instrument (15 bp), could not be detected. Meanwhile, lane 7 of the same figure presented the CViKI-1 digestion product (three fragments of length 45, 31, and 20 bp). The other fragments (13 and 11 bp) were below the lower end resolution and hence, remained undetected. On the other hand, endogenous control (141 bp) produced two AluI (127 and 14 bp) and four CViKI-1 fragments (73, 39, 15, and 14 bp). However, only 127 bp (Lane 4) and 73 and 39 bp (Lane7) fragments were detected. The use of endogenous control further eliminated the chances of any false negative detection. Since conventional agarose-gel electrophoresis cannot resolute fragments of smaller differences in lengths, we used an automated lab-on-a-chip-based electrophoretic system incorporated in Experion bionalyzer.[Citation45]
In Silico Analysis of Available Monkey Species
The Old World monkey genus Macaca (Cercopithecidae: Papionini) represents one of the largest and most diverse primate families, since there are about 20 to 22 species of macaques.[Citation46] While the rest of them originated from the Africa region, there are total 13 Asian macaque species that fall into four subgroups, namely; the silenus-sylvanus group, fascicularis group, sinica group, and arctoides group.[Citation46,Citation47] Due to the unavailability of a sample procurement system of macaque species in local markets as well as strong government’s legislation, we did not get a sufficient number of macaque species in commercial pet shops and meat and fish markets. We applied to the Department of Wildlife and National Park Malaysia (PERHILITAN) for permission to use all Macaca species, including other macaque species. However, permission was given only for the Macaca fascicularis species.
We performed in silico analysis of 11 Macaca species to measure the possibility of detection with the designed primers. The d-loop gene sequence was retrieved from NCBI for all leaf-eating monkeys (Colobines) as well as commercially important meat species for theoretical analysis. Pairwise distance and phylogenetic studies revealed minimum distance within the Macaca species itself. M. fascicularis, M. nemestrina, M. arctoides, and M. fuscata were the closest species (Supplementary ). Primer mismatch analysis (Supplementary ) predicted that at least four macaque species (M. nemestrina, M. arctoides, M.sylvanus, and M. thibetana) could be amplified, since there is only one mismatch opposite of the primer. According to Wu et al.,[Citation48] a mismatch at the 3′ end of a primer-binding region may hinder the successful PCR amplification. As for the rest of macaque species, the number of mismatched nucleotides was between one to four bases. Therefore, the designated assay/primers, could hopefully be used to detect not only fradulence in the food industry, but also to trace the trafficking of any macaque species. However, this requires a practical PCR test, which we could not perform in the present study due to the lack of samples from other macaques to further confirm the universal detection.
Conclusion
A species-specific PCR assay targeting a short-fragment (120 bp) of multicopy mitochondrial d-loop gene for macaque M. fascicularis detection in raw and processed meats was successfully developed. The specificity and stability of the assay were studied against 17 meat species and under boiling, microwaving, and autoclaving treatments both under pure and mixed matrices. The assay was sensitive enough to detect as low as 0.0001 ng macaque DNA under pure states and 0.1% (w/w) macaque meat in binary mixtures. The assay was validated by RFLP analysis in presence of an endogenous control (141 bp), which eliminated the chances of any false negative detection. Distinctive restriction patterns for AluI and CViKI-1 were obtained for both targets (120 and 141 bp). We believe the assay would find application for M. fascicularis detection by regulatory bodies for the halal food industry and wildlife conservation.
Supplementary_Document.docx
Download MS Word (96.9 KB)Acknowledgment
The authors are thankful to the Department of Wildlife and National Park Malaysia for the donation of macaque meat-tissue samples for conducting this study.
Funding
This research was supported by the University of Malaya fund PPP fund no. PG0004-2013B and GC001A-14SBS to M. E. Ali.
Additional information
Funding
Notes on contributors
Md. Eaqub Ali
All authors declare no conflict of interest in publishing this article. Ethical clearance of ref. No: NANOCAT /17/07/3013/NRAR (R) was obtained from the Institutional Animal Care and Use Committee, University of Malaya (UM IACUC) and all experiments were conducted following the national and institutional guideline while handling animal meats used in this study.
Nur Raifana Abdul Rashid
All authors declare no conflict of interest in publishing this article. Ethical clearance of ref. No: NANOCAT /17/07/3013/NRAR (R) was obtained from the Institutional Animal Care and Use Committee, University of Malaya (UM IACUC) and all experiments were conducted following the national and institutional guideline while handling animal meats used in this study.
Sharifah Bee Abd Hamid
All authors declare no conflict of interest in publishing this article. Ethical clearance of ref. No: NANOCAT /17/07/3013/NRAR (R) was obtained from the Institutional Animal Care and Use Committee, University of Malaya (UM IACUC) and all experiments were conducted following the national and institutional guideline while handling animal meats used in this study.
S. M. Azad Hossain
All authors declare no conflict of interest in publishing this article. Ethical clearance of ref. No: NANOCAT /17/07/3013/NRAR (R) was obtained from the Institutional Animal Care and Use Committee, University of Malaya (UM IACUC) and all experiments were conducted following the national and institutional guideline while handling animal meats used in this study.
Asing Asing
All authors declare no conflict of interest in publishing this article. Ethical clearance of ref. No: NANOCAT /17/07/3013/NRAR (R) was obtained from the Institutional Animal Care and Use Committee, University of Malaya (UM IACUC) and all experiments were conducted following the national and institutional guideline while handling animal meats used in this study.
M. A. Motalib Hossain
All authors declare no conflict of interest in publishing this article. Ethical clearance of ref. No: NANOCAT /17/07/3013/NRAR (R) was obtained from the Institutional Animal Care and Use Committee, University of Malaya (UM IACUC) and all experiments were conducted following the national and institutional guideline while handling animal meats used in this study.
I. S. M. Zaidul
All authors declare no conflict of interest in publishing this article. Ethical clearance of ref. No: NANOCAT /17/07/3013/NRAR (R) was obtained from the Institutional Animal Care and Use Committee, University of Malaya (UM IACUC) and all experiments were conducted following the national and institutional guideline while handling animal meats used in this study.
References
- Karabasanavar, N.S.; Singh, S.P.; Umapathi, V.; Girish, P.S.; Shebannavar, S.N.; Kumar, D. Authentication of Carabeef (Water Buffalo, Bubalus Bubalis) Using Highly Specific Polymerase Chain Reaction. European Food Research and Technology 2011, 233, 985–989.
- Ali, M.E.; Rahman, M.M.; Hamid, S.B.A.; Mustafa, S.; Bhassu, S.; Hashim, U. Canine-Specific PCR Assay Targeting Cytochrome B Gene for the Detection of Dog Meat Adulteration in Commercial Frankfurters. Food Analytical Methods 2014, 7, 234–241.
- Fajardo, V.; González, I.; Martín, I.; Rojas, M.; Hernández, P.E.; García, T.; Martín, R. Differentiation of European Wild Boar (Sus Scrofa Scrofa) and Domestic Swine (Sus Scrofa Domestica) Meats by PCR Analysis Targeting the Mitochondrial D-Loop and the Nuclear Melanocortin Receptor 1 (MC1R) Genes. Meat Science 2008, 78, 314–322.
- Milner-Gulland, E.J.; Bennett E.L. Wild Meat: The Bigger Picture. Trends in Ecology & Evolution 2003, 18, 351–357.
- Hoffman, L.C.; Wiklund, E. Game and Venison—Meat for the Modern Consumer. Meat Science 2006, 74, 197–208.
- Hoffman, L.; Cawthorn, D. What is the Role and Contribution of Meat from Wildlife in Providing High Quality Protein for Consumption. Animal Frontiers 2012, 2, 40–53.
- Estrada, A. Human and Non-Human Primate Co-Existence in the Neotropics: A Preliminary View of Some Agricultural Practices as a Complement for Primate Conservation. Ecological and Environmental Anthropology 2006, 2, 2.
- Bennett, E.L. Is There a Link Between Wild Meat and Food Security? Ecological and Environmental Anthropology 2002, 16, 590–592.
- Bennett, E.L.; Robinson, J.G. Hunting of Wildlife in Tropical Forests: Implications for Biodiversity and Forest Peoples. Biodiversity Series—Impact Studies, Environment Department. The World Bank 2000, 76, 1–56.
- Eudey, A.A. The Crab-Eating Macaque (Macaca Fascicularis): Widespread and Rapidly Declining. Primate Conservation 2008, 23, 129–132.
- Creagh, S. Two Arrested Two over Monkey Meatballs. Reuters 2010. http://www.reuters.com/article/2010/04/28/us-monkey-meatballs-idUSTRE63R35M20100428 (accessed Jan. 11, 2016)
- Brown, C. Emerging Zoonoses and Pathogens of Public Health Significance—An Overview. Revue Scientifique et Technique-Office International des Epizooties 2004, 23, 435–442.
- Georges-Courbot, M.C.; Sanchez, A.; Lu, C.Y.; Baize, S.; Leroy, E.; Lansout-Soukate, J. Isolation and Phylogenetic Characterization of Ebola Viruses Causing Different Outbreaks in Gabon. Emerging Infectious Diseases 1997, 3, 59–62.
- Chomel, B.B.; Belotto, A.; Meslin, F.X. Wildlife, Exotic Pets, and Emerging Zoonoses. Emerging Infectious Diseases 2007, 13, 6–11.
- Ayaz, Y.; Ayaz, N.; Erol, I. Detection of Species in Meat and Meat Products Using Enzyme‐Linked Immunosorbent Assay. Journal of Muscle Foods 2006, 17, 214–220.
- Chou, C.-C.; Lin, S.-P.; Lee, K.-M.; Hsu, C.-T.; Vickroy, T.W.; Zen, J.-M. Fast Differentiation of Meats from Fifteen Animal Species by Liquid Chromatography with Electrochemical Detection Using Copper Nanoparticle Plated Electrodes. Journal of Chromatography B 2007, 846, 230–239.
- Kesmen, Z.; Sahin, F.; Yetim, H. PCR Assay for the Identification of Animal Species in Cooked Sausages. Meat Science 2007, 77, 649–653.
- Matsunaga, T.; Chikuni, K.; Tanabe, R.; Muroya, S.; Shibata, K.; Yamada, J.; Shinmura, Y. A Quick and Simple Method for the Identification of Meat Species and Meat Products by PCR Assay. Meat Science 1999, 51, 143–148.
- Arslan, A.; Ilhak, O.I.; Calicioglu, M. Effect of Method of Cooking on Identification of Heat Processed Beef Using Polymerase Chain Reaction (PCR) Technique. Meat Science 2006, 72, 326–330.
- Dooley, J.J.; Paine, K.E.; Garrett, S.D.; Brown, H.M. Detection of Meat Species Using Taqman Real-Time PCR Assays. Meat Science 2004, 68, 431–438.
- Ali, M.E.; Hashim, U.; Mustafa, S.; Che Man, Y.B.; Yusop, M.H.M.;. Bari, M.F; Islam, Kh.N.; Hasan, M.F. Nanoparticle Sensor for Label Free Detection of Swine DNA in Mixed Biological Samples. Nanotechnology 2011, 22, 195503.
- Ali, M.E.; Hashim, U.; Mustafa, S.; Che Man, Y.B.; Islam, Kh.N. Gold Nanoparticle Sensor for the Visual Detection of Pork Adulteration in Meatball Formulation. Journal of Nanomaterials 2012, 2012, 103607.
- Smith, S.; Vigilant, L.; Morin, P.A. The Effects of Sequence Length and Oligonucleotide Mismatches on 5′ Exonuclease Assay Efficiency. Nucleic Acids Research 2002, 30, e111.
- Rojas, M.; González, I.; Pavón, M.Á.; Pegels, N.; Lago, A.; Hernández, P.E.; Garcia, T.; Martín, R. Novel TaqMan Real-Time Polymerase Chain Reaction Assay for Verifying the Authenticity of Meat and Commercial Meat Products from Game Birds. Food Additives and Contaminants 2010, 27, 749–763.
- Karabasanavar, N.S.; Singh, S.P.; Umapathi, V.; Kumar, D.; Shebannavar, S.N. Identification of Goat Meat Using Highly Species‐Specific Polymerase Chain Reaction. Journal of Food Quality 2011, 34, 142–149.
- Calvo, J.H.; Rodellar, C.; Zaragoza, P.; Osta, R. Beef- and Bovine-Derived Material Identification in Processed and Unprocessed Food and Feed by PCR Amplification. Journal of Agricultural and Food Chemistry 2002, 50, 5262–5264.
- Haunshi, S.; Basumatary, R.; Girish, P.; Doley, S.; Bardoloi, R.; Kumar, A. Identification of Chicken, Duck, Pigeon, and Pig Meat by Species-Specific Markers of Mitochondrial Origin. Meat Science 2009, 83, 454–459.
- Ali, M.E.; Rahman, M.M.; Hamid, S.B.A.; Mustafa, S.; Bhassu, S.; Hashim, U. Canine-Specific PCR Assay Targeting Cytochrome B Gene for the Detection of Dog Meat Adulteration in Commercial Frankfurters. Food Anal. Methods 2014, 7, 234–241.
- Ali, M.E.; Hashim, U.; Mustafa, S.; Che Man, Y.B. Swine-Specific PCR-RFLP Assay Targeting Mitochondrial Cytochrome B Gene for Semiquantitative Detection of Pork in Commercial Meat Products. Food Analytical Methods 2012, 5, 613–623.
- Rahman, M.M.; Ali, M.E.; Hamid, S.B.A.; Mustafa, S.; Hashim, U.; Hanapi, U.K. Polymerase Chain Reaction Assay Targeting Cytochrome B Gene for the Detection of Dog Meat Adulteration in Meatball Formulation. Meat Science 2014, 97, 404–409.
- Ali, M.E.; Hashim, U.; Mustafa, S.; Che Man, Y.B.; Dhahi, T.S.; Kashif, M.; Uddin, M.K.; Hamid, S.B.A. Analysis of Pork Adulteration in Commercial Meatballs Targeting Porcine-Specific Mitochondrial Cytochrome B Gene by Taqman Probe Real-Time Polymerase Chain Reaction. Meat Science 2012, 91, 454–459.
- Ali, M.E.; Kashif, M.; Uddin, K.; Hashim, U.; Mustafa, S.; Che Man, Y.B. Species Authentication Methods in Foods and Feeds: The Present, Past, and Future of Halal Forensics. Food Analytical Methods 2012, 5, 935–955.
- Md-Zain, B.; Mohamad, M.; Ernie-Muneerah, M.; Ampeng, A.; Jasmi, A.; Lakim, M.; Mahani, M. Phylogenetic Relationships of Malaysian Monkeys, Cercopithecidae, Based on Mitochondrial Cytochrome C Sequences. Genet. Mol. Res 2010, 9, 1987–1996.
- Abdul-Latiff, M.A.B.; Ruslin, F.; Fui, V.V.; Abu, M., Ryan, J.J.R.; Abdul-Patah, P.; Lakim, M.; Roos, C.; Yaakop, S.; Md-Zain, B.M. Phylogenetic Relationships of Malaysia’s Long-Tailed Macaques, Macaca Fascicularis, Based on Cytochrome B Sequences. ZooKeys 2014, 407, 121–139.
- Hayasaka, K.; Fujii, K.; Horai, S. Molecular Phylogeny of Macaques: Implications of Nucleotide Sequences from an 896-Base Pair Region of Mitochondrial DNA. Molecular Biology and Evolution 1996, 13, 1044–1053.
- Blancher, A.; Bonhomme, M.; Crouau-Roy, B.; Terao, K.; Kitano, T.; Saitou, N. Mitochondrial DNA Sequence Phylogeny of 4 Populations of the Widely Distributed Cynomolgus Macaque (Macaca Fascicularis Fascicularis). Journal of Heredity 2008, 99, 254–264.
- Che Man, Y.B.; Mustafa, S.; Mokhtar, K.; Fadhilah, N.; Nordin, R.; Sazili, A.Q. Porcine-Specific Polymerase Chain Reaction Assay Based on Mitochondrial D-Loop Gene for Identification of Pork in Raw Meat. International Journal of Food Properties 2012, 15, 134–144.
- Karabasanavar, N.S.; Singh, S.P.; Kumar, D.; Shebannavar, S.N. Detection of Pork Adulteration by Highly-Specific PCR Assay of Mitochondrial D-Loop. Food Chemistry 2014, 145, 530–534.
- Lockley, A.; Bardsley, R. DNA-Based Methods for Food Authentication. Trends in Food Science Technology 2000, 11, 67–77.
- Hazim, M.M.Y.; Mustafa, S.; Che Man, Y.B.; Omar, A.R,; Mokhtar, N.F.K. Detection of Raw Pork Targeting Porcine-Specific Mitochondrial Cytochrome B Gene by Molecular Beacon Probe Real-Time Polymerase Chain Reaction. Food Analytical Methods 2012, 5, 422–429.
- Meyer, R.; Candrian, U. PCR-Based DNA Analysis for the Identification and Characterization of Food Components. LWT–Food Science Technology 1996, 29, 1–9.
- Ilhak, O.I.; Arslan, A. Identification of Meat Species by Polymerase Chain Reaction (PCR). Turkish Journal of Veterinary & Animal Sciences 2007, 31, 159–163.
- Mane, B.; Mendiratta, S.; Tiwari, A. Beef Specific Polymerase Chain Reaction Assay for Authentication of Meat and Meat Products. Food Control 2012, 28, 246–249.
- Maede, D. A Strategy for Molecular Species Detection in Meat and Meat Products by PCR-RFLP and DNA Sequencing Using Mitochondrial and Chromosomal Genetic Sequences. European Food Research and Technology 2006, 224, 209–217.
- Rahman, M.M.; Ali, M.E.; Hamid, S.B.A.; Bhassu, S.; Mustafa, S.; Al Amin, M.; Razzak, M.A. Lab-on-a-Chip PCR-RFLP Assay for the Detection of Canine DNA in Burger Formulations. Food Analytical Methods 2015, 8(6), 1598–1606.
- Li, J.; Han, K.; Xing, J.; Kim, H.S.; Rogers, J.; Ryder, O.A.; Disotell, T.; Batzer, M.A. Phylogeny of the Macaques (Cercopithecidae: Macaca) Based on Alu Elements. Gene 2009, 448, 242–249.
- Fooden, J. Provisional Classifications and Key to Living Species of Macaques (Primates: Macaca). Folia Primatol. (Basel) 1976, 25, 225–236.
- Wu, J.-H.; Hong, P.-Y.; Liu, W.-T. Quantitative Effects of Position and Type of Single Mismatch on Single Base Primer Extension. Journal of Microbiological Methods 2009, 77, 267–275.