ABSTRACT
The mortiño fruit (Vaccinium meridionale Swartz) has been recognized as an excellent source of antioxidants, phenolic compounds, and anthocyanins. Drying conditions, particularly temperature, often lead to food quality degradation. The present study investigated the influence of drying temperature (40, 50, and 60°C) on antioxidant activity, anthocyanin, and phenol content of mortiño. Four different thin layer drying models of drying kinetics (Modified page, Newton, Henderson & Pabis and, two-terms) were fitted to the experimental data. For antioxidant capacity determination, oxygen radical absorbance capacity and ferric ion reducing antioxidant power assays were used. The results showed that antioxidant capacity, and phenolic and anthocyanin content all decreased with increasing temperature and drying time. It was observed that phenols and anthocyanins were conserved in greater amounts at 60°C with 34% (5.85 mg gallic acid/g dm) and 32% (2.36 mg Cyanidin-3-glucoside/g dm) preservation of initial content, respectively. Drying kinetics models were compared based on their R2 and root mean square error values between the experimental and predicted moisture ratios. The two-terms model was found to satisfactorily describe the drying curves for all temperatures evaluated, with a determination coefficient (R2) above 0.9987 and root mean square error lower than 0.0201.
Introduction
Mortiño (Vaccinium meridionale Swartz) is a berry of 5–10 mm in diameter, dark reddish in color, with a sour and tart flavor.[Citation1] The fruit is globose, fleshy, dark purple-to-black when ripe, and has numerous small seeds.[Citation2] It is a fruit with high potential for commercialization due to its high antioxidant capacity, phenolic compounds, anthocyanins, flavonoids, and health benefits.[Citation3–Citation7] Its antioxidant properties are attributed primarily to its content of Cyanidin 3-galactoside and chlorogenic acid, providing similar or better antioxidant properties than other species of Vaccinium.[Citation1,Citation8]
The fruit became widely known to herbalists in the 16th century, when it was used for treating bladder stones, biliary disorders, scurvy, coughs, and lung tuberculosis.[Citation1] It has been shown to have the potential to prevent oxidative damage caused by reactive oxygen species.[Citation9] Polyphenolic compounds inhibit several oxidative and inflammatory enzymes and have been shown to have antiallergenic, antiviral, antibacterial, antifungal, antitumor, atheroprotective and hypoglycemic properties, and antihemorrhagic activity.[Citation9,Citation10] The antioxidative activity of food-based polyphenolics is based upon the cumulative effects of chemical and enzymatic processes that occur during processing and/or storage.[Citation11]
Several researchers have experimentally studied the effects of drying and concluded that increases in drying temperature diminish nutraceutical properties.[Citation12–Citation15] Related studies have also investigated the effects of various drying technologies (e.g., vacuum drying, freeze drying, hot air drying, principally) on the antioxidant capacity of fruits.[Citation16,Citation17] Despite several researchers having reported that drying is generally considered to be unfavorable due to the possibility of inducing oxidative decomposition, either enzymatically or by thermal degradation of polyphenols, high antioxidant potentials of dried fruits were reported by Kamiloglu and Capanoglu.[Citation18] Additionally, increases in phenolic and flavonoid content, as well as antioxidant activity, due to drying have even been reported.[Citation19,Citation20] In this sense, water plays an important role in biological reactions, including physical, chemical, microbiological, and enzyme reactions.[Citation21]
Due to the limited information currently available about the dehydration of mortiño, the aim of this study was to investigate changes in its antioxidant activity as a function of drying temperature. The effects of drying temperature (40, 50, and 60ºC) on the antioxidant capacity and content of phenols and anthocyanins during the drying process were analyzed. Characteristic curves of drying and the drying rate of mortiño fruit were then determined, and Newton, Modified page, Henderson & Pabis, and two-terms models were evaluated for correlation to the dehydration kinetics.
Materials and Methods
Raw Materials
The mortiño fruits were obtained on the Guijas farm, located in the municipality of El Retiro, Antioquia, Colombia in good physical condition with an adequate degree of ripeness, and an average moisture content of 82.81 ± 0.025% (w/w). The fruits were treated with a chlorine solution (100 ppm) to remove dirt and were then stored at –5 ± 0.5°C prior to undergoing the dehydration process. The mortiño fruits were cut into halves, and the samples were then placed into a dehydration chamber.
Drying Equipment and Method
Drying treatments were performed in a food dehydrator (LEM trademark, Ohio, USA) in a batch process. The drying chamber had a volume of 0.15 mCitation3, air was circulated inside by a 0.15 kW axial fan at a constant velocity (0.5 ms–Citation1 ± 0.1), and heat was generated by electrical resistance (1.5 kW). Mortiño samples were cut into halves and distributed on stainless steel trays within the drying chamber. The temperature was adjusted manually by a thermostat, with a range of 40–60 ± 1°C, which was verified using a PT1000 resistance temperature sensor (Swagelok, Texas, USA). Moisture loss was periodically measured by taking samples out and weighing them on a digital balance with a precision of 0.01 g (Ohaus Adventurer Pro, New Jersey, USA). The initial moisture content was measured by a moisture analyzer (Ohaus MB45, New Jersey, USA). Three replicates of each experiment were performed and the average of these results was calculated.
Mathematical Modeling
The study of drying characteristics is important to be able to model the drying behavior effectively. The data obtained experimentally for the three different temperatures studied were plotted as the moisture ratio (MR) versus time. The MR of samples during drying is generally calculated by the following equation:
where W is the moisture content at the time of measurement, We is the equilibrium moisture content, and W0 is the initial moisture content, all expressed in terms of dry basis (g water/g dry matter). Experimental drying curves were modeled using four separate empirical equations: Newton, Henderson-Pabis, Modified page, and two-terms ().[Citation22–Citation24]
Determination of Phenolic Compound Content and Antioxidant Activity
For laboratory analysis, samples were taken during the drying process at 15 min intervals during the first hour and then at 60 min intervals up to a total of 300 min. The extracts were obtained using the method described by Kuskoski et al.[Citation25] Each sample was weighed, and 5.0 g of the sample was centrifuged at 4000 rpm for 20 min. The supernatant was then recovered and stored at –20°C for the analysis. Sequential extractions were performed with 25-mL tri-distilled water at room temperature for 1 h.
Determination of total anthocyanin content
The pH differential method, as described by Gaviria et al.,[Citation26] allows an estimate of total anthocyanin content. Briefly, absorbance of cyanidin-3-glucoside with a molar extinction coefficient (ε) of 26,900 L mol–Citation1 cm–Citation1 and a molar mass (MM) of 449.2 g mol–Citation1 was measured at 530 and 700 nm in buffers at pH 1.0 (0.025 mol·L–Citation1 potassium chloride buffer) and 4.5 (0.4 mol·L–Citation1 sodium acetate buffer; Sigma-Aldrich, St. Louis, MO, USA) using a spectrophotometer (Thermo Scientific Multiskan, Finland). Anthocyanin content was then calculated using Eq. (2).[Citation27]
Results were expressed as milligrams cyanidin-3-glucoside/g dry matter.
Oxygen radical absorbance capacity (ORAC) assay
ORAC is a hydrogen atom transfer (HAT)-based assay that measures the capability of antioxidants to quench peroxyl radicals by hydrogen donation.[Citation28] The method described by Prior et al.[Citation29] and Romero et al.[Citation30] was used with minor modifications. The solutions (30 μL) were added to 21 μL of fluorescein (0.01 M) prepared in 2.899 μL of 75 mM sodium phosphate buffer (PBS; pH 7.4) and 50 μL 2,2′-Azobis (2-methylpropionamidine) dihydrochloride, AAPH (Sigma-Aldrich, St. Louis, MO, USA; 0.6 M) in PBS, with the temperature adjusted to 37°C and the pH to 7.4. The fluorescence of the above reaction was monitored. Values were taken at an excitation wavelength of 493 nm with an excitation slit of 10 nm, an emission wavelength of 515 nm with an emission slit of 15 nm, and an attenuator of 1%. The protective effect of the antioxidant was calculated using the differences in the areas under the decay curves of fluorescein in the absence and in the presence of the sample and compared against a standard curve, using Trolox as a primary reference. The results were expressed as Trolox equivalents (μmol Trolox equivalents/g dm) according to the following equation:
where AUC is the area under the sample curve, AUC° is the area under the control curve (absence of sample), AUC Trolox is the area under the Trolox reference curve, f is the dilution factor of the extracts (i.e., the ratio between the total volume of the working solution [target molecule plus AAPH plus berry extract] and the added berry extract volume) and [Trolox] is the Trolox molar concentration.
Ferric ion reducing antioxidant power (FRAP) assay
To assess the antioxidant potential of bioactive compounds, the antioxidant capacity was determined by the FRAP method using the procedure reported by Benzie and Strain,[Citation31] with some modifications. This method is based on the increase in absorbance due to the formation of 2,4,6-tripyridil-s-triazine (TPTZ)-Fe (II; Sigma-Aldrich, St. Louis, MO, USA) in the presence of reducing agents. The FRAP reagent contains 2.5 mL of TPTZ (10 μM) in 40 mM HCl, 2.5 mL of FeCl3 (20 μM), and 25 mL acetate buffer at pH 3.6 (0.3 μM). A volume of 50 μL of extract was mixed with 950 μL FRAP reagent (Sigma-Aldrich, St. Louis, MO, USA). The change in absorbance (590 nm) was measured and the reducing capacity was calculated using the absorbance difference between the sample and blank and the reference FeCitation2+ standard solution. The FRAP values were expressed as ascorbic acid equivalent antioxidant capacity (AEAC; mg ascorbic acid per g dm) using the ascorbic acid standard curve of Rojano et al.[Citation32]
Total phenolic content (Folin–Ciocalteu assay)
The Folin–Ciocalteu reagent method[Citation33] was used with some modifications to determine the total phenolic content. Fruit extracts (50 μL) were mixed with 125 μL of Folin–Ciocalteu reagent (Merck Millipore, Darmstadt, Germany) and 400 μL of sodium carbonate (7.1% w/v), and the resulting solution was brought to a final volume of 1000 μL. The mixture was stirred and stored at room temperature, in the dark, for 30 min. The absorbance was measured in triplicate at 760 nm against a blank. Aqueous solutions of gallic acid (Sigma-Aldrich, St. Louis, MO, USA) were used for the calibration curve, and the results are expressed as mg of gallic acid equivalents (GAE)/g dm
Hydroxycinnamic acids/high-performance liquid chromatography (HPLC)
Hydroxycinnamic acids were determined by HPLC analysis. The aqueous extract was filtered (0.45 μm pore size filter) prior to injection. The chromatograph was equipped with a SIL-20A auto injector/HT, a CBM-20A communication module and a SPD-M20A photodiode array detector (PDA) calibrated at 320 nm. Quantification was performed on a Merck RP18 column with a 5 μm particle size, 250 mm length, and 4.0 mm diameter. The mobile phase was comprised of methanol and acidified water with 0.1% formic acid. The flow rate of the mobile phase was 0.6 mL/min, and the other conditions were 50°C and gradient elution. The ultraviolet (UV)-visible spectrum from 200 to 600 nm was measured for all peaks; identification and quantification of the compounds were performed using calibration curves for each of the phenolic acids using the method of Naranjo et al.[Citation34] with some modifications.
Statistical Analysis
The determination coefficient (R2) and root mean square error (RMSE) were used as the fit criteria for the data. High values of R2 together with low values of the RMSE corresponded to a high goodness of fit.[Citation35,Citation36]
Results and Discussion
Drying Experiments
Experimental drying results are shown in . shows the drying kinetics obtained at three different temperatures (40, 50, and 60°C). The effect of temperature on drying time was observed, as increasing the air temperature decreases the amount of drying time needed to reach the equilibrium moisture content. The initial moisture content was 4.81 ± 0.8 g H2O/g dm. At the end of the drying process, the moisture contents were 0.22, 0.932, and 2.2135 g H2O/g dm at 60, 50, and 40°C, respectively.
As expected, increasing the drying temperature accelerated the evaporation of water and led to an increase in mass transfer. Similar results were obtained by Doymaz[Citation23] and Mohapatra and Srinivasa[Citation37] working with other species of fruits and vegetables. The drying rate curves for the three temperatures are shown in . There was no constant drying rate in any of the drying conditions because halves of mortiño could not provide a constant supply of moisture during the drying process. The observed results were similar to the reported drying characteristics of many agricultural products.[Citation38,Citation39]
When surface moisture is insufficient, the drying speed is dominated by the diffusion of moisture from the interior to the surface,[Citation40] i.e., the drying rate reduces with time and reduction of moisture content. Temperature is the primary driving force of drying, and the time required to complete the process depends on the energy supplied. Experimental drying curves were modeled with four empirical equations (), which are described above. The results are shown in .
Table 1. Kinetic models from literature.
Table 2. Values of the drying constants and coefficients of different models determined through regression method.
Figure 2. Comparison of experimental data with the predictive moisture ratio data from the two-terms model.
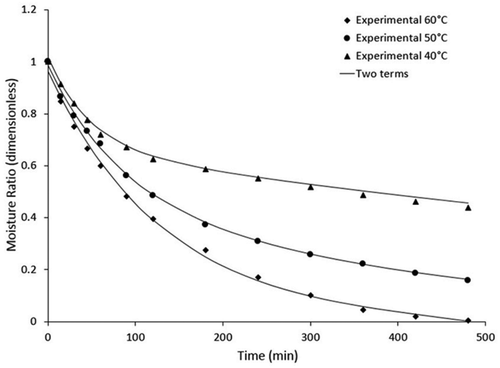
An exponential trend is observed for the drying kinetics, validating the use of models proposed a priori to simulate the whole drying process. Although all models fit the experimental almost perfectly, the two-terms model showed the best statistical fit, i.e., lower values of RMSE (0.0065, 0.0113, 0.0201 for 40, 50, and 60°C, respectively) and R2 values close to unit (0.9989, 0.9987, 0.9973 for 40, 50, and 60°C, respectively). As seen in , the value of the constant b for the two-terms model increased with temperature, while the values of a and k5 each decreased with temperature.
Effect of Temperature on Phenolic Content and Antioxidant Activity
Anthocyanins
shows that anthocyanin content decreased during drying. Total anthocyanin content preservations of 24% at 40°C, 13% at 50°C, and 18% at 60°C (1.79, 1.21, and 2.36 mg cyanidin-3-glucoside/g dm, respectively) were observed. This observation is comparable to blueberries, with a content of 3.18 mg cyanidin-3-glucoside/g dm with a temperature of 70°C for 10 h. The results were compared to other drying methods such as osmotic dehydration and ultrasound (1.95 and 5.83 mg cyanidin-3-glucoside/g dm, respectively).[Citation41] According to Vashisth et al.,[Citation16] this behavior can be attributed to the polyphenolic and anthocyanin content that is reduced as a consequence of long exposure time and high temperatures. De Ancos, Ibañez, Reglero, and Cano[Citation42] suggested that anthocyanin and other polyphenolic compounds may degrade depending upon many factors, e.g., the activity of polyphenol oxidase, organic acid content, sugar concentration, and pH. In addition, during long thermal treatments, polyphenol oxidase can lead to a marked reduction in the phenolic constituents.
Total phenol content (TPC)
The TPC was determined using the Folin-Ciocalteu assay. As shown in , TPC decreased with time. These results show that phenols were preserved in greater proportion at 60°C. At the end of the drying process, 25, 29, and 34% of the phenolic content was preserved (3.87, 6.50, and 5.85 mg GAE/g dm at 40, 50, and 60°C, respectively) in relation to the initial phenolic content. These results are similar to those reported by Bennett et al.[Citation28] of fruits such as Zante currant (7.5 mg GAe/g dm) and prune (5.69 GAE/g dm) dried by hot air, as well as the results reported by Bhouri et al.[Citation27] on Tunisian raisin varieties (4.47–6.54 GAE/g dm). According to Gümüşay et al.,[Citation11] activation of oxidative enzymes such as polyphenoloxidase and peroxidase during the drying process may have led to the loss of phenolic complexes. Vashisth et al.,[Citation16] suggested that polyphenols are rendered thermolabile by prolonged heat treatment, leading to the destruction of flavonoids and tannins during drying. Additional reasons for the loss of phenolic content may include binding of phenols to proteins, changes in chemical structures, or inadequate extraction capacities of the methods presently available.[Citation11] The disruption of cells may also result in a release of oxidative and hydrolytic enzymes, which are capable of oxidizing endogenous polyphenolics. However, exposure to high temperatures for even a short time can inactivate these enzymes and protect the polyphenolics from further decomposition. Swati and Poonam[Citation43] have reported phenolic compounds decreased with the increased temperature and storage time, which may be due to oxidation and polymerization of phenolic compounds.
This behavior may be related to the drying process at low temperatures, which leads to long drying times that can promote a reduction in the antioxidant capacity.[Citation44] Temperature usually has a negative effect on antioxidants, which can be attributed to irreversible oxidative processes during drying. Vashisth et al.[Citation16] suggested that because phenolic acids are mainly bonded to carbohydrate and proteinaceous moieties, their release during thermal processing might occur due to a breakdown of cellular constituents and covalent bonds. According to Chong et al.,[Citation45] phytochemical compounds can oxidize during hot-air drying because the food material has greater contact with oxygen. Direct exposure to hot air also destroys the color, vitamins, and flavor of fruits.
Measuring antioxidant capacity using the ORAC assay
shows that ORAC values were considerably higher during the first hour of the drying process and that these values decreased with time at all temperatures utilized in this study. It was observed that the ORAC values at 50°C were lower than those at 40 and 60°C; therefore, no direct correlation between drying temperature and antioxidant capacity was observed. The temperature with the highest retention of antioxidant capacity was 40°C (54%), followed by 60°C (47%) and finally 50°C (20%) with observed values of 7,424, 538.41, and 221.16 μmol Trolox/g dm, respectively. All mortiño extracts suppressed the consumption of fluorescein throughout the induction time. Induction times were generated by reactive phenols present in the extracts of mortiño.[Citation46]
FRAP assay
As shown in , antioxidant capacities of 13.7, 12.61, and 32.2% (4.57, 5.70, and 8.05 mg ascorbic acid/g dm) of initial values were observed at 40, 50, and 60°C, respectively. This indicates that mortiño fruits dried at 60°C had an antioxidant capacity 20% higher than those dried at lower temperatures. Temperature is generally recognized to have a negative effect on antioxidant capacity, which can be attributed to irreversible oxidative processes during drying. According to Sun, Shen, Liu, and Ye[Citation47] and Mejia-Meza et al.,[Citation48] free phenolic acids can become liberated from the bound form of phenolic acids at increased temperatures. Phenolic acids might also be susceptible to destruction at increased temperatures. Furthermore, the antioxidant capacity of foods can be affected by long drying times.
Chlorogenic acid
Leusink et al.[Citation49] have shown that berries have high antioxidant activity; several compounds contributing to this characteristic, including chlorogenic acid, can vary depending on the variety and maturity of the fruit. shows the content of chlorogenic acid present in the mortiño at the end of the drying process relative to fresh berries. Chlorogenic acid in mortiño is labile to oxidation by the effects of the drying temperature, and a considerable decrease in relation to fresh berries (5.36 mg chlorogenic acid/g dm) was likewise observed. However, it was also observed that when dried at 60°C, the content of chlorogenic acid is more stable than when dried at lower temperatures (1.26, 1.38, and 2.02 mg chlorogenic acid/g dm at 40, 50, and 60°C, respectively). This result is comparable to those obtained by Kamiloglu and Capanoglu,[Citation18] as they found 1.1 mg chlorogenic acid/g dm in purple figs after sun drying.
Ferulic acid
Phenolic acids (e.g., caffeic acid, chlorogenic acid, p-coumaric, and ferulic acid) are secondary metabolites that are found in berries and contribute to their antioxidant capacity. shows that mortiño fruits were sensitive to heat treatment. Antioxidant content was found to decrease with increasing temperature, as 77.95, 69.92, and 70.23% (0.88, 0.79, and 0.80 mg Ferulic acid/g dm) of initial values were observed following drying at 40, 50, and 60°C, respectively. According to Arrieta-Baez et al.,[Citation50] the high reactivity of ferulic acid to decarboxylation and the rapid dimerization of 4-vinylguaiacol under high temperature conditions could lead to an increase in the antioxidant capacity of the final product. However, they also noted an increase in the production of 4-vinylguaiacol at 30 min followed by decay of the compound minutes later. They note that their reported results were for samples subjected to the drying process for 300 min. Chong et al.[Citation45] suggested that the reduced levels of polyphenolics and other active compounds found in dried fruits were related to antioxidant activity and indicated that decreases in antioxidant activity resulted from the degradation of biologically active compounds at high temperatures due to chemical, enzymatic, or thermal decomposition. The total antioxidant activity of a given food item is the result of the activities of each antioxidant component of that food item. Furthermore, the antioxidant components of a food item can interact among themselves to produce synergistic or inhibitory effects.[Citation51]
Conclusions
The results are in agreement with the other studies reported in the literature. However, differences in thermal stability are attributable to the various phenolic compounds mortiño and especially the presence of anthocyanin as cyanidin and delphinidin glucosides. The present study represents the data describing the changes in phenolic compounds and antioxidant capacity of Mortiño fruit as a result of dried at 40, 50, and 60°C under forced convection (0.5 m/s). The results demonstrate how the phenolic compounds and antioxidant activity are modified upon fruit processing and the changes to fruit quality after the drying. Drying curves indicated a decreasing drying rate over time; no periods of constant drying rate were observed. It was observed that temperature had a considerable effect on the variation of MRs with respect to drying time. The initial moisture content of the samples was found to be 4.81 g H2O/g dm. Following drying at 40, 50, and 60°C, moisture contents of 0.9304, 0.4387, and 0.2270 g H2O/g dm, respectively, were observed. The two-terms model provided the best fit with the experimental data of MRs versus drying time for all drying conditions (R2 values of 0.9989, 0.9987, and 0.9973 at 40, 50, and 60°C, respectively). The characteristic drying curve of the mortiño fruit was established.
In relation to preservation of the antioxidant capacity of dehydrated fruits, a negative correlation between ascorbic acid and antioxidant activity was observed. The effect of drying methods on antioxidant retention depends on the temperature and length of the drying process. Temperature had a negative effect on the antioxidant capacity, which can be attributed to irreversible oxidative processes occurring during drying. Although the effect was negative at all temperatures studied, higher percentages of antioxidant activity and total phenolic content preservation were observed at 60°C. Their release during thermal processing might occur due to the breakdown of cellular constituents and covalent bonds.
Phytochemical compounds can be oxidized when food material has greater contact with oxygen. Direct exposure to hot air also destroys antioxidant and phenolic compounds. Total phenolic content showed the lowest amount of destruction when fruits were dried at 60°C, which was probably due to the formation of new compounds with antioxidant properties at high temperatures. It was observed that large changes in the antioxidant capacity of mortiño fruit occurred during the first hour of drying; therefore, it is important to carefully monitor drying temperatures during this time period. Our results indicated that the observed decrease in antioxidant activity resulted from degradation of biologically active compounds during drying, leading to chemical, enzymatic, or thermal decomposition. According to the results the total phenolic content as unique methodology to study the drying of fruits rich in sensitive compounds such as anthocyanins, is not recommended. Thus for future work mortiño drying is recommended to study presence of phenolic compounds including anthocyanins and phenolic acids as main components, by HPLC techniques to understand the changes in antioxidant activity during the drying process. Further improvements on optimization of the process (varying the temperature and time drying, pretreatment, other drying methods, texturing) could improve the quality of final product of nutraceutical foods with value-added.
Funding
We would thank the “Benito Juárez” Autonomous University of Oaxaca for financial support that made transportation to the Medellín campus of the National University of Colombia School of Mines possible.
Additional information
Funding
References
- Garzón, G.A.; Narváez, C.E.; Riedl, K.M.; Schwartz, S.J. Chemical Composition, Anthocyanins, Non-Anthocyanin Phenolics, and Antioxidant Activity of Wild Bilberry (Vaccinium Meridionale Swartz) from Colombia. Food Chemistry 2010, 122, 980–986.
- Luteyn, J.L. Diversity, Adaptation, and Endemism in Neotropical Ericaceae: Biogeographical Patterns in the Vaccinieae. Botanical Reviews 2002, 68(1), 55–87.
- Kalt, W.; McDonald, J.E.; Donner, H. Anthocyanins, Phenolics, and Antioxidant Capacity of Processed Lowbush Blueberry Product. Journal of Food Science 2000, 65, 390–393.
- Prior, R.L.; eCao, G.; Martin, A.; Sofic, E.; McEwen, J.; O’Brien, C. Antioxidant Capacity as Influenced by Total Phenolic and Anthocyanin Content, Maturity, and Variety of Vaccinium Species. Journal of Agricultural and Food Chemistry 1998, 46(7), 2686–2693.
- Yuan, W.; Zhou, L.; Dengee, G.; Wang, P.; Creech, D.; Li, S. Anthocyanins, Phenolics, and Antioxidant Capacity of Vaccinium L. in Texas; USA Pharm. C. 2011, 2, 11–13.
- Castrejón, A.; Eichholz, I.; Rohn, S.; Kroh, L.; Huyskens-Keil, S. Phenolic Profile and Antioxidant Activity of Highbush Blueberry (Vaccinium Corymbosum L.) During Fruit Maturation and Ripening. Food Chemistry 2008, 109(3), 564–572.
- Veggi, C.P.; Meireles, A.A. Anthocyanin Extraction from Jabuticaba (Myrciaria Cauliflora) Skins by Different Techniques: Economic Evaluation. Procedia Food Science 2011, 1, 1725–1731.
- Rojano, B.; Ochoa, C.; Sánchez, N.; Medina, C.; Lobo, M.; Galeano, P.; Mosquera, A.; Tamayo, A.; Lopera, Y.; Gaviria, C. Propiedades Antioxidantes de los Frutos de Agraz o Mortiño (Vaccinium Meridionale Swartz). In Perspectivas de Cultivo de Agraz o Mortiño (Vaccinium Meridionale Swartz) en la Zona Altoandina de Colombia, Ligarreto, G.; Ed.; Universidad Nacional de Colombia: Bogotá, 2009; 95–112.
- Prencipe, F.; Bruni, R.; Guerrini, A.; Rossi, D.; Benvenuti, S.; Pellati, F. Metabolite Profiling of Polyphenols in Vaccinium Berries and Determination of Their Chemopreventive Properties. Journal of Pharmaceutical and Biomedical Analysis 2014, 89, 257–267.
- Cavalcanti, R.N.; Santos Diego, T.; Meireles, M.A. Non-Thermal Stabilization Mechanisms of Anthocyanins in Model and Food Systems—An Overview. Food Research International 2011, 44(2011), 499–509.
- Gümüşay, Ö.A.; Borazan, A.A.; Ercal, N.; Demirkol, O. Drying Effects on the Antioxidant Properties of Tomatoes and Ginger. Food Chemistry 2015, 173, 156–162.
- Demarchi, S.M.; Quintero Ruiz, N.A.; Concellón, A.; Ginera, S.A. Effect of Temperature on Hot-Air Drying Rate and on Retention of Antioxidant Capacity in Apple Leathers. Food and Bioproducts Processing 2010, 91(4), 310–318.
- Miranda, M.; Maureira, H.; Rodríguez, K.; Vega-Gálvez, A. Influence of Temperature on the Drying Kinetics, Physicochemical Properties, and Antioxidant Capacity of Aloe Vera (Aloe Barbadensis Miller) Gel. Journal of Food Engineering 2009, 91, 297–304.
- Kuljarachanan, T.; Devahastin, S.; Chiewchan, N. Evolution of Antioxidant Compounds in Lime Residues During Drying. Food Chemistry 2009, 113, 944–949.
- Katsube, T.; Tsurunaga, Y.; Sugiyama, M.; Furuno, T.; Yamasaki, Y. Effect of Air-Drying Temperature on Antioxidant Capacity and Stability of Polyphenolic Compounds in Mulberry (Morus Alba L.) Leaves. Food Chemistry 2009, 113, 964–969.
- Vashisth, T.; Singh Rakesh, K.; Pegg Ronald, B. Effects of Drying on the Phenolic Content and Antioxidant Activity of Muscadine Pomace. LWT–Food Science and Technology 2011, 44, 1649–1657.
- Methakhup, S.; Chiewchan, N.; Devahastin, S. Effect of Drying Methods and Conditions on Drying Kinetics and Quality of Indian Gooseberry Flake. LWT–Food Science and Technology 2005, 38, 579–587.
- Kamiloglu, S.; Capanoglu, E. Polyphenol Content in Figs (Ficus Carica L.): Effect of Sun-Drying. International Journal of Food Properties 2015, 18, 521–535.
- Piga, A.; DelCaro, A.; Corda, G. From Plums to Prunes: Influence of Drying Parameters on Polyphenols and Antioxidant Activity. Journal of Agricultural and Food Chemistry 2003, 51, 3675–3681.
- López-Vidaña, E.C.; Rojano, B.; Pilatowsky, F.I.; Zapata, K.; Cortés, F. Evaluation of the Sorption Equilibrium and Effect of Drying Temperature on the Antioxidant Capacity of the Jaboticaba (Myrciaria Cauliflora). Chemical Engineering Communications. 2015.
- Fennema, O.R.; Damodaran, S.; Kirk, L.P. Fennema’s Food Chemistry, 4th Ed; CRC Press: New York, NY, 2007.
- Azzouz, S.; Guizani, A.; Jomaa, W.; Belghit, A. Moisture Diffusivity and Drying Kinetic Equation of Convective Drying of Grapes. Journal of Food Engineering 2002, 55, 323–330.
- Doymaz, I. Sun Drying of Figs: An Experimental Study. Journal of Food Engineering 2005, 71, 403–407.
- Kaya, A.; Aydın, O.; Kolaylı, S. Effect of Different Drying Conditions on the Vitamin C (Ascorbic Acid) Content of Hayward Kiwifruits (Actinidia Deliciosa Planch). Food and Bioproducts Processing 2010, 88(2–3), 165–173.
- Kuskoski, M.E.; Asuero, A.G.; Troncoso, A.M.; Manzini-Filho, J.; Fett, R. Aplicación de Diversos Métodos Químicos para Determinar Actividad Antioxidante en Pulpa de Frutos. Food Science and Technology (Campinas), 2005, 25(4), 726–732.
- Gaviria, M.; Carlos, A.; Cifuentes, O.A.; Monsalve, G.; Carlos, E.; Rojano, B.A. Actividad Antioxidante de Extractos Metanólicos de Attalea Butyracea Scientia Et Technica, Vol. XIII, núm. 33, Universidad Tecnológica de Pereira Pereira: Colombia, 2007; 297–298.
- Mnari, A. B.; Harzallah, A.; Amri, Z.; Dhaou Aguir, S.; Hammami, M. Phytochemical Content, Antioxidant Properties, and Phenolic Profile of Tunisian Raisin Varieties (Vitis Vinifera L.). International Journal of Food Properties, 2016; 19(3), 578–590.
- Bennett, L.E.; Jegasothy, H.; Konczak, I.; Frank, D.; Sudharmarajan, S.; Clingeleffer, P.R. Total Polyphenolics and Anti-Oxidant Properties of Selected Dried Fruits and Relationships to Drying Conditions. Journal of Functional Foods 2011, 3(2), 115–124.
- Prior, R.L.; Wu, X.; Schaich, K. Standardized Methods for the Determination of Antioxidant Capacity and Phenolics in Foods and Dietary Supplements. Journal of Agricultural and Food Chemistry 2005, 53, 4290−4302.
- Romero, M.; Rojano, B.; Mella-Raipán, J.; Pessoa-Mahana, C.D.; Lissi, E.; López-Alarcón, C. Antioxidant Capacity of Pure Compounds and Complex Mixtures Evaluated by the ORAC-Pyrogallol Red Assay in the Presence of Triton X-100 Micelles. Molecules 2010, 15(9), 6152–6167.
- Benzie, I.F.F.; Strain, J.J. The Ferric Reducing Ability of Plasma (FRAP) as a Measure of “Antioxidant Power:” The FRAP Assay. Analytical Biochemistry 1996, 76, 70–76.
- Rojano, B.; Saez, J.; Schinella, G.; Quijano, J.; Vélez, E.; Gil, A. Experimental and Theoretical Determination of the Antioxidant Properties of Isoespintanol (2-Isopropyl-3,6-Dimethoxy-5-Methylphenol). Journal Molecular Structures 2008, 877(1–3), 1–6.
- Singleton, V.L.; Rossi, J.A. Colorimetry of Total Phenolics with Phosphomolybdic-Phosphotungstic Acid Reagents. American Journal of Viticulture 1965, 16, 144–158.
- Naranjo, M.; Velez, L.; Rojano, B. Actividad Antioxidantes de Café Colombiano de Diferentes Calidades. Revista Cubana Plantas Medicinales 2011, 16(2), 164–173.
- Doymaz, I. Convective Drying Kinetics of Strawberry. Chemical Engineering and Processing: Process Intensification 2008, 47(5), 914–919.
- Wang, Z.; Sun, J.; Chen, F.; Liao, X.; Hu, X. Mathematical Modelling on Thin Layer Microwave Drying of Apple Pomace with and Without Hot Air Pre-Drying. Journal of Food Engineering 2007, 80, 536–544.
- Mohapatra, D.; Srinivasa, R. A Thin Layer Drying Model of Parboiled Wheat. Journal of Food Engineering 2005, 66, 513–518.
- Banout, J.; Ehl, P.; Havlik, J.; Lojka, B.; Polesny, Z.; Verner, V. Design and Performance Evaluation of a Double-Pass Solar Drier for Drying of Red Chilli (Capsicum Annum L.). Solar Energy 2011, 85(3), 506–515.
- Lahsasni, S.; Kouhila, M.; Mahrouz, M.; Jaouhari, J.T. Drying Kinetics of Prickly Pear Fruit (Opuntia Ficus Indica). Journal of Food Engineering 2004, 61(2), 173–179.
- Chen, D.; Zheng, Y.; Zhu, X. Determination of Effective Moisture Diffusivity and Drying Kinetics for Poplar Sawdust by Thermogravimetric Analysis Under Isothermal Condition. Bioresource Technology 2012, 107, 451–455.
- Stojanovic, J.; Silva, J. Influence of Osmotic Concentration, Continuous High Frequency Ultrasound and Dehydration on Antioxidants, Colour, and Chemical Properties of Rabbiteye Blueberries. Food Chemistry 2007, 101, 898–906.
- de Ancos, B.; Ibañez, E.; Reglero, G.; Cano, M.P. Frozen Storage Effects on Anthocyanins and Volatile Compounds of Raspberry Fruit. Journal of Agricultural and Food Chemistry 2000, 48, 873–879.
- Swati, K.; Poonam, A. Drying Method Affects Bioactive Compounds and Antioxidant Activity of Carrot. International Journal of Vegetable Science 2015, 21, 467–481.
- Vega-Gálvez, A.; Di Scala, K.; Rodríguez, K.; Lemus-Mondaca, R.; Miranda, M.; López, J.; Perez-Wona, M. Effect of Air-Drying Temperature on Physico-Chemical Properties, Antioxidant Capacity, Colour, and Total Phenolic Content of Red Pepper (Capsicum Annuum, L. Var. Hungarian). Food Chemistry 2009, 117, 647–653.
- Chong, C.H.; Law, C.L.; Figiel, A.; Wojdyło, A.; Oziembłowski, M. Colour, Phenolic Content, and Antioxidant Capacity of Some Fruits Dehydrated by a Combination of Different Methods. Food Chemistry 2013, 141(4), 3889–3896.
- Atala, E.; Vásquez, L.; Speisky, H.; Lissi, E.; López-Alarcón, C. Ascorbic Acid Contribution to ORAC Values in Berry Extracts. An Evaluation by the ORAC-Pyrogallol Red Methodology. Food Chemistry 2009, 113, 331–335.
- Sun, Y.; Shen, Y.; Liu, D.; Ye, X. Effects of Drying Methods on Phytochemical Compounds and Antioxidant Activity of Physiologically Dropped Un-Matured Citrus Fruits. LWT–Food Science and Technology 2015, 60(2), 1269–1275.
- Mejia-Meza, E.I.; Yanez, J.A.; Davies, N.M.; Rasco, B.; Younce, F.; Remsberg, C.M.; Clary, C. Improving Nutritional Value of Dried Blueberries (Vaccinium Corymbosum L.) Combining Microwave-Vacuum, Hot-Air Drying, and Freeze Drying Technologies. International Journal of Food Engineering 2008, 4, 1–6.
- Leusink G.; Kitts D.; Yaghmaee P.; Timdurance. Retention of Antioxidant Capacity of Vacuum MicrowaveDried Cranberry. Journal of Food Science, 2010, Vol. 75, Nr. 3.
- Arrieta-Baez, D.; Dorantes-Alvarez, L.; Martinez-Torres, R.; Zepeda-Vallejo, G.; Jaramillo-Flores, E.; Ortiz-Moreno, A.; Aparicio-Ozores, G. Effect of Thermal Sterilization on Ferulic, Coumaric, and Cinnamic Acids: Dimerization and Antioxidant Activity. Journal of the Science of Food and Agriculture 2012, 92(13), 2715–2720.
- Abreu, W.; Piccolo, B.M.; Barros Vilas Boas, E.; Pablo da Silva, E. Total Antioxidant Activity of Dried Tomatoes Marketed in Brazil. International Journal of Food Properties 2014, 17, 639–649.