ABSTRACT
In order to use commercial starch for flavor encapsulation, three starches (mungbean, rice, and tapioca) were selected to determine their ability to form inclusion complex with menthone. Mungbean starch had the highest amylose content, followed by rice starch and tapioca starch, respectively. After complexation, appearance and microstructure changes were observed for all starches. Mungbean and tapioca complexes with well-organized V7 crystalline pattern gave similar menthone entrapment (around 4%) which was higher than that of the rice sample (<1%). Molecular size distribution of the complexes revealed the role of amylose fractions for both mungbean and tapioca complexes. According to differential scanning calorimetric analysis, menthone complexes formation was confirmed by a thermoreversible event. Regarding the high yield of starch precipitate and menthone entrapment, mungbean starch is considered a more suitable material for menthone encapsulation rather than tapioca and rice starches.
Introduction
Flavor encapsulation is an advantageous technique which improves food flavor perception and numerous developments have been established in order to give several favorable properties of the encapsulated flavors.[Citation1,Citation2] Inclusion complexes between flavors and starches are of interest because of their retention and controlled release. Starch might be used as a flavor encapsulation material since the formation of a helical arrangement of amylose plays an important role in inclusion complex formation.[Citation3–Citation6] Guest molecules of suitable size, shape and hydrophobicity are able to localize in the amylose cavity to form stable complexes which are insoluble at room temperature.[Citation7] A variety of flavor compounds has been reported for their ability to form starch inclusion complex with the flavor loading of 2–10%.[Citation3,Citation5,Citation6]
Different starches display different complexing ability as influenced by the content and the structure of their amylose. Starch with high amylose content tends to include greater amount of flavor compounds.[Citation6] Furthermore, the binding capacity is also depending on the chain length of the amylose or the degree of polymerization (DP).[Citation3] In South East Asia, mungbean, rice, and tapioca starches are available as low cost food ingredients compared with the high amylose starch or pure amylose. However, no reports have been found on inclusion complexes of mungbean while rice starch was known to form complex with many ligands,[Citation8] and tapioca starch was reported to form inclusion complexes with many flavor compounds especially alcohols.[Citation4] Thus, different composition among starch sources should attribute to deviation in complexation ability with flavor compounds, especially amylose. Mungbean starch has higher amylose content (30%) compared to potato starch which commonly used as complexing material with many flavors, while rice and tapioca starches has the similar range (17–20%).[Citation9] The advantage of using starches as encapsulation material is not only their lower price but also the synergistic effect of native lipid on the flavor loading capacity.[Citation5] For complex preparation process, it has been reported that the formation of starch flavor complex can be induced by heating an aqueous starch dispersion at temperature of 120–140°C with various periods of time.[Citation3,Citation4,Citation10]
Menthone, the mint-like aroma compound, has been reported to form an inclusion complex with some starches, such as rice, potato, high amylose maize, and corn.[Citation5,Citation6,Citation8,Citation11] The interaction between tapioca starch and menthone dispersions were investigated.[Citation12] However, the entrapment of menthone and the ability to form inclusion complex with commercial starches are limited. The aim of this study was, therefore, to investigate the ability to form inclusion complexes of three types of commercial starch with menthone in order to compare the possibility to use food ingredient as encapsulant materials.
Materials and methods
Materials
Commercial mungbean starch (Pine Brand, Sithinan Co., Ltd., Thailand) and tapioca starch (Jade Leaf Brand, Bangkok Interfood Co., Ltd., Thailand) were purchased from a local supermarket. Commercial rice starch was obtained from Siam flour company (Bangkok, Thailand). Menthone (purity 90%, Aldrich) was used as an aroma compound and amylose from potato was purchased from Sigma–Aldrich (St. Louis, MO, USA). All other chemicals were of analytical grade and solvents were of high-performance liquid chromatography (HPLC) grade.
Chemical composition analysis of starches
Before complexing with menthone, the moisture, protein and lipid contents of all starch samples were determined according to the official methods of the Association of Official Analytical Chemists.[Citation13] The apparent amylose content was determined by colorimetric method[Citation14] and high-performance size exclusion chromatography with refractive index detector (HPSEC-RI) according to the method of Kim et al.[Citation15] Non-granular starch was prepared before analysis. The HPSEC system consisting of a pump (HPLC pump model 600, Waters, US), two analytical columns (Shodex OHpak SB-806 and SB 400 HQ, Shodex, Japan) in a 55°C column oven, and a differential refractometer (Model 410, Waters, US) was performed at 40ºC. Deionized water was used as an eluent, and the flow rate was 0.6 mL/min. The relative ratio of starch fraction was determined using the same extraction method as reference. The relationship between retention time and molecular weight of standard pullulans (molecular weight 6.2–805 kDa) was used for molecular weight distribution analysis.
Preparation of starch menthone inclusion complex
The complex of starch and menthone was prepared by dispersing an aqueous solution of starch (0.8% w/v) in a retort at 140ºC for 1 h. The starch solution was subsequently cooled to 80ºC for 15 min in a water bath before addition of menthone (0.1% v/v) and maintained at this temperature for 1 h. The starch menthone mixture was allowed to cool to room temperature overnight. One drop of dispersion was placed on microscope glass slide and covered with glass cover before examining under light microscope (Axiolab.re, Carl Zeiss, Germany). The appearance and microstructure changes of the freshly prepared samples were then evaluated. Finally, the precipitates were collected by centrifugation at 12,000 × g for 20 min at 20°C and then freeze dried. The control sample was prepared in the similar method as above without menthone added.
Examination of starch-menthone inclusion complexes
Yield of starch precipitates
Since only a small amount of starch precipitate was obtained, an indirect method was used to determine the amount of starch precipitated from the starch menthone complex mixture. After centrifugation of the starch menthone complex mixture, the amount of starch left in the supernatant was determined by the phenol-sulfuric method.[Citation16]
Determination of the entrapped menthone
Before quantitative analysis, the excess non-complexed menthone and lipid compounds on the surfaces of freeze dried starch-menthone complexes were removed by washing with hexane.[Citation17] Dried complexes (10 mg) were washed once with 4 mL hexane for 20 min at room temperature. After washing, the samples were dried in a fume hood for 10 min and then in a desiccator overnight. The samples were dispersed in alkaline solution and the entrapped menthone were extracted by hexane and quantitated by gas chromatography (GC). An internal standard, 2-decanone, was added to the solvent at a concentration of 100 ppm. The GC (HP-6890; Agilent Technologies, U.S.) was equipped with an HP-5 column (30 m × 0.32 mm × 0.25 µm) and a flame ionization detector. The oven temperature regime was: hold at 40°C for 2 min, heat to 180°C at the rate of 5°C/min, heat to 250°C in 2 min, and hold at 250°C for 3 min.
X-ray diffraction measurement
The freeze dried starch-menthone complexes and their control samples were examined by a wide angle x-ray diffractometer (WAXD) to characterize their crystalline properties. Analysis was conducted using an x-ray diffractometer (JEOL, JDX-3530, 2kW, Japan) with Cu kα radiation source and accelerating at 30 kV and 40 mA. Each dried powder was measured in the 2θ angle range between 4º and 30º at a scan rate of 2.0 s/step and a step size of 0.02º. Jade software was used for data analysis.
HPSEC
To characterize the molecular structure of the complexes the freeze dried starch–menthone samples were dispersed in deionized water before boiling for 60 min. The filtered samples were then analyzed using HPSEC-RI system under the same condition used for starch analysis.
Thermal analysis
Since the structure of the complexes might change by annealing from heat during drying,[Citation18] the freshly prepared starch–menthone precipitate was investigated by differential scanning calorimetry (DSC) without drying. Approximately 80 mg of the blotted precipitate was weighed into a stainless steel DSC pan. Since the blotted sample did not gain from fresh rice precipitates. Freeze-dried sample before DSC analysis has been reported to have no alteration on DSC results which obtained from dried-rehydrated sample of lipid amylose complexes.[Citation18] Therefore, freeze-dried sample was used and deionized water was added with similar moisture content as those of mungbean and tapioca inclusion samples. DSC analysis was carried out with Mettler-Toledo DSC1 differential scanning calorimeter (Mettler-Toledo, Italy). The pan was held at 0ºC for 1 min, then heated to 180ºC at 10ºC/min, cooled from 180 to 0ºC at 10ºC/min and reheated from 0 to 180ºC at 10ºC/min. For control sample, the deionized water was added into the freeze-dried starch suspension to give the similar moisture content as the blotted sample. The sample pan was allowed to equilibrate overnight before measurement. The moisture content of each sample was determined after DSC analysis according to the method of Tapanapunnitikul et al.[Citation5]
Statistical analysis
The data were expressed as the mean ± standard deviation of triplicate determinations except for WAXD and DSC data which were analyzed in duplicate. Duncan’s multiple range test was used to differentiate mean values, with significance defined at p < 0.05. Statistical analysis was performed using the software SPSS 12.0 (SPSS Inc., Armonk, NY, USA)
Results and discussion
Chemical composition of starches
Moisture, protein, lipid, and amylose contents of the starches are presented in . Rice had the highest protein and lipid contents compared with those of mungbean and tapioca starches (p < 0.05). For amylose content, both colorimetry and HPSEC showed that mungbean starch had the highest amylose content (p < 0.05) followed by rice and tapioca starches, respectively. Similar result was reported by Jane et al.,[Citation9] mungbean starch contains the higher amount of amylose than rice and tapioca starches.
Table 1. Chemical composition of mungbean, rice, and tapioca starches.
Appearance and microstructure of starch menthone complexes
After a 24 h incubation, the suspensions precipitated as a result of inclusion complex formation as shown in for mungbean and tapioca starch samples. These may be explained by the lower water solubility due to the small configurational entropy of the complexes compared to the random coil conformation of the native starch molecules.[Citation19] In contrast, the menthone inclusion complex of the rice starch sample did not settle at the bottom of the tube but the suspension became more turbid compared to the clear suspension of the control sample.
Figure 1. Appearance changes of different starches when complexing with menthone. Comparing between control starch samples (C) and menthone complexes (M).
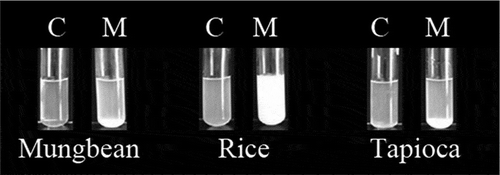
In micrometer range, light micrographs revealed differences in size between menthone rice starch complex and the others, mungbean and tapioca complexes (). The predominant of complexes had an average diameter of 13–18 μm (69 and 78% for mungbean and tapioca complexes, respectively); while rice complex structure could not be seen under light microscope. The complexes with smaller diameter (8–12 μm) were also observed (9.7 and 7.0% for mungbean and tapioca complexes, respectively). About 21.7 and 14.7% of larger complexes (19–29 μm) were found for mungbean and tapioca complexes, respectively. Birefringence was not detected when examined under polarized light and none of the starch granules were observed which indicated that all starch granules were disrupted prior to complex formation and new supramolecular structures were formed upon complexing with menthone.
Yield of starch precipitates and percentage of menthone entrapment
The influence of different starches on the yield of starch precipitate and flavor entrapment after menthone complexation are presented in . Determination via an indirect method showed that rice starch gave the highest yield of starch precipitates (65.45 ± 8.77%), followed by mungbean (24.59 ± 6.43%), and tapioca starches (17.76 ± 6.47%), respectively (p < 0.05). However, quantitative analysis of the entrapped menthone by GC revealed maximum loading of menthone at 4% by mungbean and tapioca starches while less than 1% was found for the rice starch. Different results have also been reported. Higher entrapment (10%) exhibited by high amylomaize starch[Citation5] might be related to its higher amylose content (70%). However, the complexation method might also contribute to the different flavor entrapment since lower menthone loading (2%) of high amylomaize starch was obtained with the acidification method.[Citation6]
Table 2. Yield of starch precipitation, menthone entrapment and stoichiometrya of starch menthone complexes.
Interestingly, the amount of entrapped menthone was lowest in rice starch, whereas the yield of precipitate was highest (p < 0.05). Since a soft gel was obtained after centrifugation of the rice starch menthone complex suspension as a result of the different complexation behavior between rice starch and menthone, therefore, the amount of menthone entrapment of rice starch might not directly correspond to the yield of the starch precipitate. According to stoichiometry of starch menthone complexes (), rice menthone complex also had the higher mole ratio of starch to menthone than those of mungbean and tapioca complexes. Mungbean and tapioca starches had higher affinity to form inclusion complex with menthone since only 23 mole of anhydroglucose unit (AGU) was required to form complex with mole of menthone. Lipid has been reported as one of the factors that influence on starch complexation. Both of competitive effect of monostearate on menthone starch binding in a model system[Citation20] and the synergistic effect of lipid on menthone complexation with high amylomaize starch have been proposed.[Citation5] In the present study, the highest lipid content of rice starch did not increase the ability of menthone complexation although its content was in the same range of high amylomaize starch. Therefore, possible affecting factors on menthone complexation might be origin of starches or difference of amylose sources.
Crystalline pattern
WAXD was performed in order to identify the polymorph of the complexes. illustrates the crystalline pattern obtained for menthone complexes of mungbean and tapioca starches with peaks at 6.8, 12.9, and 18.0° typical of V7 crystalline structure as exhibited by starch–menthone inclusion complexes.[Citation21] The results also matched closely the pattern of amylose and high amylose starch when complexing with menthone.[Citation5,Citation6] However, all control starch samples were identified as amorphous materials which revealed that the retrogradation did not occur by this method of complexation. From these data could be concluded that the crystalline structure of the complexes were formed by complexing with menthone.
Figure 3. WAXD pattern for menthone inclusion complexes and control samples: (a) mungbean (b) rice and (c) tapioca starch.

The contribution of amylose in the V-form peak has been discussed; the sharper V-form peaks are increased as the amylose content increased.[Citation6] In the present study, however, mungbean and tapioca starches could form menthone inclusion complexes with similar relative crystallinity even though the amylose content of mungbeans is much higher. Furthermore, a broadening of the two major reflection peaks (12.9 and 18.0°) indicative of amylose inclusion complex with less crystallinity was observed for rice samples (p < 0.05). These data were in agreement with the light micrographs of menthone starch complexes (). The smaller size of rice menthone complexes may be the result of an aggregation of the smaller helical materials, making them less crystalline than mungbean and tapioca menthone complexes. This loose pack crystalline also collaborates to the lowest menthone entrapment capacity of the rice starch.
HPSEC profiles
Molecular size distribution of starches and menthone complexation samples are shown in . All starches exhibited the profile which the sharp peak of amylopectin was eluted before the broad amylose fraction at different ratios. Tapioca starch had the largest amylose molecule (MW 11.43 ± 2.55 × 105 g/mol), followed by mungbean starch (MW 4.76 ± 2.13 × 105 g/mol), and rice starch (MW 1.29 ± 0.13 × 105 g/mol), respectively (p < 0.05). After complexing with menthone, the narrow peaks of small molecules were found in mungbean (MW 5.98 ± 1.11 × 104 g/mol) and tapioca (MW 7.19 ± 0.99 × 104 g/mol) samples which indicated the role of amylose in the formation of the inclusion complex. In addition, a small broad amylopectin fraction was presented in both mungbean and tapioca samples unlike the molecular profile of the rice menthone complex which revealed a distinct amylopectin peak and a broader, much less prominent fraction of small molecules (MW 9.48 ± 0.67 × 104 g/mol).
The shortest amylose of rice starch (DPw 798 ± 80) exhibited the lowest entrapment of menthone. However, the longest tapioca amylose (DPw 7060 ± 1565) showed the similar menthone entrapment to mungbean amylose (DPw 2935 ± 1312). This indicated that the amylose chain length influenced the complexing ability between these starches and menthone. Source of amylose was also reported as the major effect on complexing with hexanal by Wulff et al.[Citation3] and there was less effect on the DP of enzymatically prepared amyloses above 290.
The smaller amylose of rice starch may result in the lower complexing ability since the lower DP could reversibly solubilize into the medium. Furthermore, a large fraction of amylopectin was also found in the rice menthone complex which might result in low solubility in water due to its high molecular weight. These data might also explain the conceivably highest starch precipitate yield of rice starch as a result of the connection between the initial inclusion complex which was smaller in size and some of the amorphous segments. This might be related to the formation of soft gel for rice menthone complexes. In addition, rice amylopectin has the highest molecular weight and gyration radius among the three starches studied[Citation22] which might impede the formation of rice inclusion complex in spite of the high amylose content of the rice starch. The highest precipitate yield of rice complex might not be caused by a true menthone entrapment which indicating by its lowest menthone entrapment and the presence of amylopectin fraction in the complex. Therefore, rice starch was not a good material for menthone encapsulation.
Thermal properties
The thermoproperties of the three controls (moisture content 81–83%) are shown in . During the initial heating from 0 to 180ºC at 10ºC/min, the endothermic peak was observed with melting temperature in the range of 130–140°C. The broad endotherm might result from reorganization of the starch structure after cooling of the heated starch dispersion without menthone added. Subsequent cooling and reheating the control samples at the same rate did not show any endothermic or exothermic peak which might be due to the condition in the sample pan was less preferable for starch polymer rearrangement,[Citation23] especially holding time which greatly influenced on starch retrogradation.[Citation24]
In contrast to the control samples, initial heating showed that the starch menthone complexes needed the energy to melt the crystalline region as shown in . Peak temperature (Tp) of mungbean and tapioca complexes (around 113°C) were similar to that of the amylomaize starch menthone complex (Tp 119°C) reported by Tapanapunnitikul et al.[Citation5] While rice complex had a lower Tp of 110°C. These findings might be explained by the lower energy required to uncoil the helices of the less compact structure of rice menthone complex. A lower Tp of rice sample was in agreement with a lower crystallinity and smaller size from WAXD studies when compared to mungbean and tapioca complexes. However, the largest endotherm of rice sample was observed with the lowest crystallinity. This might be explained by the smaller size of rice complex. The decreasing of transition enthalpy has been reported which resulted from the larger and more perfect crystallites of narrow size distribution of crystals of starch-glycerol monosterate complex which developed after isothermal annealing.[Citation18] Furthermore, large size of mungbean and tapioca complexes which observed under light microscope were also supported their low transition enthalpy.
The results showed that subsequent cooling could induce the formation of menthone-amylose complex with the resultant exothermic peak. This suggested that starch menthone complex could be formed at 42.9–47.6°C. After reheating, a melting endotherm was also displayed in the similar range of the initial heating (Tp 110–113°C) with lower enthalpy. This might indicate that less organized crystalline structure was formed after melting.
Conclusions
This study showed that different starches affected the ability to form menthone inclusion complexes at different levels. The impact factor on the formation of menthone inclusion complex with these three commercial starches was not only the content of their amylose but also the size. The shortest amylose of rice starch gave the lowest menthone complexing affinity. However, the longest amylose of tapioca starch did not give the higher menthone complexing affinity when compared with mungbean amylose. The higher precipitation yield of mungbean starch was more practical for application. Thus, using mungbean starch as carrier for flavor encapsulation would be advantageous.
Funding
A part of this research was funded by Center of Advanced Studies for Agriculture and Food, Institute for Advanced Studies, Kasetsart University, Bangkok 10900, Thailand (CASAF, NRU-KU, Thailand) and Kasetsart University Research and Development Institute (KURDI).
Additional information
Funding
References
- Madene, A.; Jacquot, M.; Scher, J.; Desobry, S. Flavour Encapsulation and Controlled Release – A Review. International Journal of Food Science and Technology 2006, 41(1), 1–21.
- Yuliani, S.; Torley P.J.; Bhandari B. Physical and Processing Characteristics of Extrudates Made from Starch and d-Limonene Mixtures. International Journal of Food Properties 2009, 12(3), 482–495.
- Wulff, G.; Avgenaki, G.; Guzmann, M.S.P. Molecular Encapsulation of Flavours as Helical Inclusion Complexes of Amylose. Journal of Cereal Science 2005, 41(3), 239–249.
- Itthisoponkul, T.; Mitchell, J.R.; Taylor, A.J.; Farhat, I.A. Inclusion Complexes of Tapioca Starch with Flavour Compounds. Carbohydrate Polymers 2007, 69(1), 106–115.
- Tapanapunnitikul, O.; Chaiseri, S.; Peterson, D.G.; Thompson, D.B. Water Solubility of Flavor Compounds Influences Formation of Flavor Inclusion Complexes from Dispersed High-Amylose Maize Starch. Journal of Agricultural and Food Chemistry 2008, 56(1), 220–226.
- Ades, H.; Kesselman, E.; Ungar, Y.; Shimoni, E. Complexation with Starch for Encapsulation and Controlled Release of Menthone and Menthol. LWT–Food Science and Technology 2012, 45(2), 277–288.
- van Ruth, S.M.; Roozen, J.P. Delivery of Flavours from Food Matrices. In Food Flavor Technology; Taylor, A.J.; Ed.; Sheffield Academic Press: Great Britain, 2002; pp. 167–184.
- Osman-Ismail, F.; Solms, J. The Formation of Inclusion Compounds of Starches with Flavor Substances. Lebensmittel-Wissenschaft und-Technologie 1973, 6(4), 147–150.
- Jane, J.; Chen, Y.Y.; Lee, L.F.; McPherson, A.E.; Wong, K.S.; Radosavljevic, M.; Kasemsuwan, T. Effects of Amylopectin Branch Chain Length and Amylose Content on the Gelatinization and Pasting Properties of Starch. Cereal Chemistry 1999, 76(5), 629–637.
- Heinemann, C.; Conde-Petit, B.; Nuessli, J.; Escher, F. Evidence of Starch Inclusion Complexation with Lactones. Journal of Agricultural and Food Chemistry 2001, 49(3), 1370–1376.
- Rutschmann, M.A.; Heiniger, J.; Pliska, V.; Solms, J. Formation of Inclusion Complexes of Starch with Different Organic Compounds. I. Method of Evaluation of Binding Profiles with Menthone as an Example. Lebensmittel-Wissenschaft und-Technologie 1989, 22(5), 240–244.
- Teitz, M.; Buettner, A.; Conde-Petit, B. Interaction Between Starch and Aroma Compounds as Measured by Proton Transfer Reaction Mass Spectrometry (PTR-MS). Food Chemistry 2008, 108(4), 1192–1199.
- AOAC. Official Methods of Analysis of the Association of Official Analytical Chemists. AOAC: Arlington, VA, 1990.
- Chrastil, J. Improved Colourimetric Determination of Amylose in Starches or Flours. Carbohydrate Research 1987, 159(1), 154–158.
- Kim, S.H.; Lee, B.H.; Baik, M.Y.; Joo, M.H.; Yoo, S.H. Chemical Structure and Physical Properties of Mung Bean Starches Isolated from 5 Domestic Cultivars. Journal of Food Science 2007, 72(9), C471–C477.
- Dubois, M.; Gilles, K.A. Colorimetric Method for Determination of Sugars and Related Substances. Analytical Chemistry 1956, 28(3), 350–356.
- Bhandari, R.; Bruce, B.; D’Acry, R.; Thi Bich, L.L. Lemon Oil to ß-Cyclodextrin Ratio Effect on the Inclusion Efficiency of ß-Cyclodextrin and the Retention of Oil Volatiles in Complexes. Journal of Agricultural and Food Chemistry 1998, 46(4), 1494–1499.
- Biliaderis, C.G.; Seneviratne, H.D. On the Supramolecular Structure and Metastability of Glycerol Monostearate-Amylose Complex. Carbohydrate Polymers 1990, 13(2), 185–206.
- Evans, D.F.; Wennerstrom, H. The Colloidal Domain: Where Physics, Chemistry, Biology and Technology Meet; VHC: New York, NY, 1994; p. 515.
- Rutschman, M.A.; Solms, J. The Formation of Ternary Inclusion Complexes of Starch with Menthone and Monostearate—A Possible Food Model System. Lebensmittel-Wissenschaft und-Technologie 1990, 23(5), 451–456.
- Rutschmann, M.A.; Solms, J. Formation of Inclusion Complexes of Starch with Different Organics Compounds. IV. Ligand Binding and Variability in Helical Conformations of V Amylose Complexes. Lebensmittel-Wissenschaft und-Technologie 1990, 23(1), 84–87.
- Yoo, S.H.; Jane, J.L. Molecular Weights and Gyration Radii of Amylopectins Determined by High-Performance Size-Exclusion Chromatography Equipped with Multi-Angle Laser-Light Scattering and Refractive Index Detectors. Carbohydrate Polymers 2002, 49(3), 307–314.
- Nuessli, J.; Putaux, J.L.; Le Bail, P.; Buleon, A. Crystal Structure of Amylose Complexes with Small Ligands. International Journal of Biological Macromolecules 2003, 33(4–5), 227–234.
- Wang, S.; Li, C.; Copeland, L.; Niu, Q.; Wang, S. Starch Retrogradation: A Comprehensive Review. Comprehensive Reviews in Food Science and Food Safety 2015, 14(5), 568–585.