ABSTRACT
Starches isolated from five corn cultivars namely C4, C5, C6, C8, and C15 were investigated for their physicochemical and structural properties. The amylose content of corn starches varied from 24.74 to 30.32 g/100 g among the cultivars. The starch granules showed polyhedral granule shapes and differences in their mean granule size ranged from 2.3 to 19.5 μm. The starch samples showed A-type diffraction pattern with strong reflection at 15.25, 18.11, and 23.33°. Pasting profile, textural, and thermal properties of corn starch showed the cultivar differences. Raman spectroscopy showed the major band intensities at 1340, 1082, 940, 865, 523, 485, 440, and 412 cm−1. Syneresis and turbidity of gelatinized pastes of starches varied among the cultivars and increased progressively with the increase in storage period. The present study can be used for identifying differences between corn genotypes for starch quality and could provide guidance to possible industries for their end use.
Introduction
Corn starch dominates nearly 80% of the global starch market.[Citation1] Starch consists of two main components amylose and amylopectin, which greatly influences the physicochemical and functional properties. Corn starch is a valuable ingredient to the food industry due to their desirable physicochemical and techno-functional properties and being widely used as a thickener, stabilizer, textural modifiers, gelling, bulking, and water retention agent in food formulations.[Citation2,Citation3] It occupies an important place either as raw material or as a food additive in the preparation of puddings, pies, confections, and salad dressings.[Citation4]
The physicochemical properties of corn starch change with the growing environment conditions.[Citation5] Diversity of corn cultivars has impact on different properties of starches as reported by several researchers.[Citation6–Citation8] Several factors, including variety, rainfall, temperature, soil type, and growing conditions affecting the starch properties and may sometimes exhibit considerable influence than genotypic difference.[Citation9,Citation10] Many factors, including composition, amylose/amylopectin ratio and granular size affect the properties of starch. Several techniques like X-ray diffractometry,[Citation11] scanning electronic microscopy,[Citation12] Raman spectroscopy,[Citation13] and properties like rapid visco analysis (RVA),[Citation10] gel textural,[Citation14] swelling power and solubility,[Citation11,Citation15] syneresis,[Citation8] and thermal properties[Citation10] have been used to study the physicochemical and techno-functional properties of starches. The diverse industrial applications have spurred toward investigating the properties of starches from different genotypes. Therefore, the objective of the present study is to compare the physicochemical and structural properties of starch isolated from different corn cultivars grown in temperate region of the Indian Himalayas.
Materials and methods
Materials
Corn cultivars were obtained from Karewa Damodar Research Centre, Sher-e-Kashmir University of Agriculture Science and Technology of Kashmir, India. Five corn cultivars namely C4, C5, C6, C8, and C15 were used during the current study, which are the prevalent cultivars in the temperate region of the Indian Himalayas. In this region July is the warmest month having an average temperature of 26.5ºC and January is the coldest month by a mean temperature of 3ºC. The average rainfall of this region is 710 mm per year. The grains were dried and cleaned manually to remove foreign matters.
Starch isolation
Starch was isolated from the corn cultivars by the method of Jiranuntakul et al.[Citation16] with slight modifications. Briefly, 250 g of corn grains were steeped to 200 mL of 0.16% sodium metabisulphite solution for 24 h. The steeped water was drained off, and then grains were washed with distilled water and ground with a blender (Crompton Greaves, CG-BX, Mumbai, India). The ground slurry was screened through a 100 mesh. The cloudy supernatant was removed, and the sediment was re-slurried in 0.2% sodium hydroxide solution. Then the supernatant was discarded, and the starch cake was re-suspended in water. The starch slurry was then passed through 325 mesh. The starch was given repeated washings with water until the pH of the starch slurry reached 7. The supernatant was then discarded and settled starch layer was re-suspended in distilled water and centrifuged at 3000 × g for 10 min. Afterward, the upper non-white layer was removed and the white layer was then collected and dried in an oven at 40ºC.
Composition
Moisture, protein, fat, and ash content of starch samples were determined according to the standard methods of AACC.[Citation17] The amylose content was determined by the method of William et al.[Citation18]
Swelling power and solubility
Swelling power and solubility of the samples were determined according to the method of Wang et al.[Citation19] with slight modification. Starch samples (500 mg) were heated with 50 mL of distilled water at a temperature of 90°C for 30 min. The samples were then cooled to the room temperature and centrifuged at 2500 × g for 15 min. The supernatant was decanted, and the residue was weighed for the estimation of swelling power. The supernatant was poured into a Petri dish and dried at 105°C and weighed. The swelling power and solubility were calculated as follows:
Color
Color was determined by International Commission on Illumination (Commission internationale de l’éclairage) (CIE) color scales L*, a*, and b* using Hunter Lab digital colorimeter (Model CX2748, Hunter Associates Laboratory, Reston, USA). L* indicates the degree of lightness or darkness of the sample extended from 0 (black) to 100 (white). a* indicates degree of redness (+a) to greenness (–a), whereas b* indicates the degree of yellowness (+b) to blueness (–b), respectively.
Scanning electron microscopy
Morphology of the starch samples was analyzed by scanning electronic microscopy (Hitachi, S-3400N, Tokyo, Japan). The samples were mounted on aluminium stubs using double-sided adhesive tape to which the samples were fixed and afterward were coated with a thin layer of gold. An acceleration potential of 15 KV was used during micrography.
X-ray diffraction
X-ray diffraction pattern was performed using an X-ray diffractometer (Shimadzu, XRD 7000) operated at a tube current (30 mA) and target voltage (40 kV) with Cu Kα radiation wavelength of 1.540. The scanning range for 2θ values was set to 10 to 60° to cover all significant diffraction peaks of sample crystallites with a scan speed of 2°/min.
Raman spectroscopy
The Raman spectra were recorded with a Raman spectrometer (InVia, Renishaw, Gloucestershire, United Kingdom), working in confocal mode. The dry samples were placed on an aluminium holder and were excited with 785 nm laser line of high-performance near infrared (HP-NIR) diode laser Renishaw (UK). The laser power was kept low enough to ensure that it did not damage the sample. Measurements were performed with the microscope and spectra were taken from the same spot of each sample in the range of 1900 to 400 cm−1.
Syneresis
Syneresis of starch samples was measured by the method of Sandhu et al.[Citation20] Starch suspensions of each cultivar (2%, w/w) were heated at 90°C for 30 min in a thermostatic water bath with constant stirring. The starch samples in separate tubes were stored for 1, 2, 3, 4, and 5 days at 4°C. Syneresis was measured as percentage amount of water released after centrifugation at 3000 × g for 10 min.
Turbidity
Turbidity of starch samples from different corn cultivars were measured by the method of Wang et al.[Citation19] with slight modification. Aqueous suspension (2%) of starch each cultivar was heated in a boiling water bath for 1 h with constant stirring. The suspension was cooled to room temperature, and then stored for 7 days at 4°C in a refrigerator. Turbidity was determined every 24 h by measuring absorbance at 640 nm against a water blank with a ultraviolet-visible (UV-Vis) Spectrophotometer (UV-1800, Shimadzu, Japan).
Pasting properties
Pasting properties of samples were determined using the RVA (Starch Master 2, Newport Scientific Pty. Ltd., Warriewood, Australia). Three grams of starch sample was added to 25 mL of distilled water in RVA aluminium canisters and stirred initially at 960 rpm for 10 s and finally at 160 rpm for the remaining test. The temperature profile was started from 50ºC for 1 min followed by raising the temperature to 95ºC for 3 min and 48 s, holding for 2 min and 30 s, cooling the system to 50ºC in 3 min and 58 s, and ending the process in 13 min. Pasting properties including peak viscosity, hold viscosity, final viscosity, breakdown viscosity, setback viscosity, and pasting temperature were recorded.
Gel textural properties
The textural properties of RVA gels of starch were determined by texture profile analysis (TA-XT2i Stable Micro Systems, Surrey, UK). The starch gels prepared during RVA were sealed in aluminium canisters and stored at 4°C for 24 h. Then each gel sample inside the tube was penetrated with a cylindrical probe (5 mm in diameter). Force-time curves were obtained at a crosshead speed of 1.5 mm s−1 during two penetration cycles. From the texture profile curve, hardness, cohesiveness, adhesiveness, gumminess, springiness, and chewiness were determined.
Thermal properties
Thermal properties of starch from each cultivar were determined by differential scanning calorimeter (DSC-2010, TA instruments, New Castle, DE, USA) using the method described by Martinez et al.[Citation21] Thermal transitions of samples for gelatinization were characterised by To (onset temperature), Tp (peak temperature), Tc (conclusion temperature) and ΔHgel (enthalpy of gelatinization).
Statistical analysis
The data were analyzed statistically using package SPSS 18.0 (SPSS Inc., Chicago, USA) and the means were separated using the Duncan’s multiple range test (p < 0.05). The data reported as averages of triplicate observations. All the data are presented as the mean with the standard deviation.
Results and discussion
Composition
The chemical composition of corn starches is shown in . The protein content of starches ranged from 0.31 to 0.54 g/100 g, fat content from 0.44 to 0.60 g/100 g, and ash content from 0.16 to 0.23 g/100 g. These results were in accordance with the earlier studies.[Citation8] Pukkahuta et al.[Citation22] reported the protein content of 0.25 g/100 g, fat 0.07 g/100 g, and ash 0.06 g/100 g for corn starches. The amylose content of corn starches varied from 24.74 to 30.32 g/100 g with the highest in C6 cultivar, followed by C5 and C15, whereas lowest in C4 cultivar. Corn starches with high amylose content have health benefits due to rich source of resistant starch. High amylose starch is presently of interest because it can lower glycaemia responses and reduce the risk for developing type II diabetes, obesity, and cardiovascular disease.[Citation3] In addition, high amylose starch is also potentially of special value for industrial utilization. The high amylose starches are characterized by their high gelling strength suggested their usefulness in the preparation of pasta, sweets, bread and in the coating fried products.[Citation23] Li et al.[Citation12] found the amylose content of 29.08 to 33.19 g/100 g for corn starches. Amylose content from 16.9 to 21.3 g/100 g was observed for starches isolated from sub-tropical corn cultivars.[Citation6] This difference in chemical composition might be due to the variation in genetic makeup, environmental and soil conditions.
Table 1. Physicochemical properties of starches isolated from corn cultivars grown in temperate region of the Indian Himalayas.
Swelling power and solubility
Significant difference is observed in the swelling power of starch (). The swelling power of corn starches varied from 10.79 to 13.55 g/g. The highest swelling power is observed in C4, whereas lowest in C6 cultivar. Uarrota et al.[Citation7] reported the swelling power in the range of 8.07 to 10.52 g/g of Brazilian maize starches. The difference in swelling power may be related to the variation in amylose content and the presence of other components of starch. The swelling power of starches has been found dependent on the water binding capacity of starch by hydrogen bonding.[Citation24] The amylopectin is likely to swell due to the weakness of the intra- and inter-molecular coherence in starch, while as, amylose acts as an inhibitor of swelling.[Citation25]
The solubility values of corn starch varied from 9.54 to 10.42%. The highest solubility values were observed in C4 followed by C5 and C8, whereas lowest in the C15 cultivar. The difference of solubility in corn starches may be due to the variation in amylose and amylopectin content, composition, viscosity patterns, and morphological difference of starch granules. Uarrota et al.[Citation7] reported the solubility index less than the present study in Brazilian maize starches. The heating leads to the swelling of insoluble starch by penetrating water and weakened the infrastructure of hydrogen bonds, and correspondingly some fragments of the starch are solubilized. The hydrogen bonds can be easily weakened in the starch granules with high amylopectin content and leading to the high solubility values.[Citation13]
Color
The color values of corn starches varied among the cultivars (). The highest L* value were observed in C5 (95.65), whereas lowest in the C15 cultivar (94.37). The a* value also varied with the highest in C6 (1.56) followed by C15 (0.44) and C8 (0.38) cultivar, whereas, b* value varied among the cultivars from 1.85 to 8.55 with the lowest in C5 and highest in C6 cultivar. The difference in color parameters (L*, a*, and b*) of starch is due to the presence of pigments in the corn kernel like carotene and phenolic compounds which considerably impact on starch quality. The difference in color parameters of corn starches were also reported in literature.[Citation8]
Morphology of starch
The granule morphology of starch from various corn cultivars viewed by scanning electronic microscopy is depicted in . The corn starch showed the presence of mainly oval to polygonal granules with size ranging from 2.3 to 19.5 μm. C4 cultivar starch showed the presence of largest size granules (4.6–19.5 μm), whereas, C15 (2.3–15.4 μm) had the smallest sizes. The average size of 4.80 to 18.80 μm for starch granules were observed for various Brazilian maize landraces Uarrota el al.[Citation7] Amaya-Llano et al.[Citation26] reported the granule size of 3.77 to15.90 μm for corn starches. The difference in granular size of starch is due to the variation in genetic makeup and geographical conditions.
X-Ray diffraction
The X-ray diffractographs of corn starch samples are shown in . All the starch samples showed typical A-type crystalline pattern with high intensity peaks at Bragg’s angle (2θ) about 15.25°, 18.11°, and 23.33°. The diffraction pattern of corn starches was similar to the other cereal starches indicating its semi-crystalline nature.[Citation27] Previous studies also reported that X-ray diffraction of corn starch showed the typical A-type pattern and mostly strong reflections at 2θ about 15°, 18°, and 23°.[Citation28]
Raman spectroscopy
Raman spectroscopy is a suitable method for structural characterization of the starch samples. Raman spectra of the starch samples in the region of 400–1900 cm−1 are shown in . The band intensity at 1460 cm−1 is a characteristic of (CH2) twisting and CH bending, whereas 1340 cm−1 to (CH2) and C–OH bending. The vibrational modes at CCH and COH are observed in the region between 1380 and 1400 cm−1, whereas vibrational bands such as CO and CC stretching and CCH, COH, and CCH deformations are observed in the region between 1200 and 1340 cm−1 bands. The Raman band at 1381 cm−1, usually assigned to δ (C–OH) and δ (C–H) modes in polymers.[Citation29] The band region between 1200 and 800 cm−1 is an associated with the CO and CC stretching and COC deformation modes, referring to the glycosidic bond. The vibrational bands at 942 cm−1 is sensitive to the ratio of linear/branched residues.[Citation30,Citation31]
Figure 3. Raman spectrum of starches isolated from corn cultivars grown in temperate region of the Indian Himalayas.
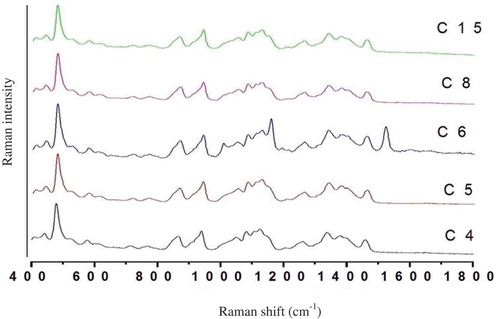
In C6 cultivar high highest peak band intensity is observed at 1510, 1490, and 1150 cm−1. The peak differentiation at 1490 cm−1 is a property of CH2 twisting and CH bending. The Raman band region between 1200 and 800 cm−1 is an associated with the CO and CC stretching and COC deformation modes, referring to the glycosidic bond. This region is also known as the fingerprint or anomeric region.
The vibrations originating from α-1,4 glycosidic linkages can be observed as strong Raman bands in the 920–960 cm−1 region, and thus the band observed at 940 cm−1 was assigned to the amylose α-1,4 glycosidic linkage. The C-O-C stretching vibration of the α-glucose ring rises at 1085 and 855 cm−1. The vibrations observed in 400 to 800 cm−1 region of starch were due to CCO and CCC deformations, and this region has a strong coupling due to the glycosidic ring skeletal deformations. The Raman band at 715 cm−1 is attributed due to the C-C stretching vibrations.[Citation32,Citation33] Mir and Bosco[Citation15] also observed the major Raman peaks at 412, 440, 485, 523, 688, 770, 940, 1082, and 1381 cm−1 in rice starches. In all the spectra of corn starch, the highest intensity showed a band at 478 cm−1 due to skeletal mode, involving (C-O-C) ring mode and (C-C-O) of α-glucose ring. The band of 865 cm−1 is assigned to symmetric C (1)-O-C (5) stretching of the α-D-glucose ring.[Citation34]
Pasting properties
Perusal of data presented in revealed the pasting properties of corn starch. Significant difference (p < 0.05) were observed among the pasting properties of starch samples of different corn cultivars. Peak viscosity of corn starch samples ranged from 3096 to 4867 cP. The highest peak viscosity was shown by C4, whereas the lowest by C15 cultivar. Pasting properties of starch are affected by the amylose content, amylose/amylopectin ratio and branched chain length.[Citation7] The increase of peak viscosity in C5 cultivar is observed due to the low protein and lipid content. The less protein and lipid content from the starch granule might increase the swelling of starch granules during pasting, which in turn increase the viscosity.[Citation15] The results are in par with Sandhu and Singh,[Citation6] who reported the peak viscosity values from 804 to 1252 cP for sub-tropical corn starches. Ali et al.[Citation8] reported the lower peak viscosity values for corn starch as compared to present study. Hold viscosity and final viscosity in the starch samples ranged from 1565 to 2240 cP and 3010 to 4271 cP, respectively. Breakdown viscosity, measure of the starch paste resistance to heat and shear, ranged from 1532 to 2898 cP with the highest in C6 cultivar (2898 cP) followed by C4 (2705 cP) and C5 (2327 cP) and lowest in C15 cultivar (1532 cP). Setback viscosity showed the tendency of starch pastes to retrograde ranged from 1444 (C15) to 2193 cP (C5). Pasting temperature ranged from 65.22 to 74.20°C with the highest value observed for C5 and lowest for C4 cultivar. The pasting properties of starches are affected by the amylose, lipid, protein contents, and swelling power.[Citation35] The differences in pasting properties of corn varieties are also reported in literature.[Citation6,Citation26,Citation36]
Table 2. Pasting and gel properties of starches isolated from corn cultivars grown in temperate region of the Indian Himalayas.
Textural properties of starch gel
The textural values of starch gels determined by textural analyzer differ significantly (p < 0.05) among the various corn cultivars (). The highest gel hardness was observed in C6 starch (64.86 g), whereas, the lowest value in C4 starch (31.33 g). The gel hardness is mainly caused by starch gel retrogradation, associated with crystallization of amylose and amylopectin.[Citation6] High amylose cultivars tend to show high gel firmness. The extent of amylose gel network and deformability of swollen granules are reported as the main factors contributing to gel strength.[Citation37] The highest adhesiveness (115.12) and springiness (0.76) was observed in C6, cohesiveness (0.50), gumminess (31.23), and resilience (0.48) in C15, whereas chewiness in C5 cultivar (19.70). These findings are consistent with the studies of Sun et al.[Citation13] The gel formation of starch mainly depends on swollen starch granules that hold water in the network within the granule. The mechanical strength of starch gels is mainly affected by composition, properties of the granules, and molecular interaction with water.[Citation15]
Syneresis
Significant difference is observed in syneresis of starch gels among the corn cultivars for 5 days storage period (). The syneresis of gels prepared from starches was measured as amount of water release from the gels during storage. C6 starch paste showed the highest syneresis value (78.5%) while C15 showed the lowest value (75%) on the zero day storage. The syneresis of corn starches increased progressively with increase in storage days. The increase in percentage syneresis during storage has been attributed due to the interaction between leached amylose and amylopectin chains, which leads to the development of functional zones and release of water.[Citation38] Previous studies reported the increase in syneresis with increased in storage period of starches.[Citation39] The syneresis of starch gels are indirectly influenced by the structural arrangement of starch chains within the amorphous and crystalline regions of ungelatinized granule, which in turn influence the extent of granule breakdown during gelatinization and the interaction that occurs between starch chains during gel storage.[Citation20] Literature also showed the differences in syneresis due to the variation in amylose, starch granule morphology, and the presence of other components in the starch.[Citation39]
Turbidity
Turbidity values of gelatinized starch suspension varied among the corn cultivars as depicted in . The differences in turbidity values have been affected by factors like granule swelling, swollen granule ruminants, leached amylose and amylopectin, amylose, and amylopectin chain lengths.[Citation40] The turbidity values of C4 and C8 starch suspension were significantly lower than the turbidity values of other corn cultivars. The lowest turbidity values of C4 and C8 starch suspension may be due to their low amylose content. The turbidity values of starch suspensions progressively increased with increase in storage period. The increase in turbidity during the storage period may be due to the leached amylose and amylopectin chains and the aggregation and crystallization of amylose that leads to the development of the functional zone which scatter a significant amount of light.[Citation41]
Thermal properties
The transition temperatures (onset temperature, To; peak temperature, Tp; endset temperature, Tc) and enthalpy of gelatinization (ΔHgel) of starches from corn cultivars differ significantly (). Among the starches from different corn cultivars, the highest To in observed in C5 (69.63°C), Tp in C15 (75.23°C), whereas Tc in C6 cultivar (82.24°C). The variation in thermal properties in starches from different cultivars might be due to the differences in amylose and amylopectin ratio. These values are in agreement with the reports on corn starches reported by Amaya-Llano et al.[Citation26] and Sandhu and Singh.[Citation6] However, these values are higher than corn starches from Chinese cultivars.[Citation10] The higher gelatinization temperature is found in C4 and C5 starch, which indicates that more energy is required to initiate starch gelatinization in these cultivars. The variation in gelatinization temperatures might be due to the difference in amounts of long chains of amylopectin, starch composition, and granular architecture. Longer chains require higher temperature to dissociate completely than that required for short double helices.[Citation42]
Table 3. Thermal properties of starches isolated from corn cultivars grown in temperate region of the Indian Himalayas.
Conclusion
Starches isolated from corn cultivars of Indian Himalayas showed differences in physicochemical and morphological characteristics. High amylose corn starches were observed in this region during the present study. Significant difference is observed in the pasting, textural, and thermal properties of starch. High hardness gels were formed which seemed to be caused by the high amylose content. The starch showed the varied granule size ranged from 2.3 to 19.5 μm. X-ray diffraction of starch showed the A-type diffraction pattern. Raman spectroscopy of starch showed the band intensities of C-H vibrational modes. Syneresis and turbidity of gelatinized pastes of starches varied among the cultivars and increased progressively with increase in storage period. These parameters as well as other functional properties would provide relevant information for useful applications of the starches in food industries. The data of this work would increase both domestic and industrial utilization of the starches and thereby enhance the production of corn cultivars.
References
- Jobling, S. Improving Starch for Food and Industrial Applications. Plant Biology 2004, 7, 210–218.
- Yousif, E.I.; Gadallah, M.G.E.; Sorour, A.M. Physico-Chemical and Rheological Properties of Modified Corn Starches and Its Effect on Noodle Quality. Annals of Agricultural Science 2012, 57, 19–27.
- Lin, J.H.; Singh, H.; Chen, F.B.; Chang, Y.H. Changes in Swelling and Rheological Properties of Corn Starches After Acid-Methanol Degradation. Food Hydrocolloids 2015, 45, 361–368.
- Arocas, A.; Sanz, T.; Fiszman, S.M. Influence of Corn Starch Type in the Rheological Properties of a White Sauce After Heating and Freezing. Food Hydrocolloids 2009, 23, 901–907.
- Lenihan, E.; Pollak, L.; White, P. Thermal Properties of Starch from Exotic-by-Adapted Corn (Zea Mays L.) Lines Grown in Four Environments. Cereal Chemistry 2005, 82, 683–689.
- Sandhu, K.S.; Singh, N. Some Properties of Corn Starches II: Physicochemical, Gelatinization, Retrogradation, Pasting and Gel Textural Properties. Food Chemistry 2007, 101, 1499–1507.
- Uarrota, V.G.; Amante, E.R.; Demiate, I.M.; Vieira, I.D.; Maraschin, M. Physicochemical, Thermal, and Pasting Properties of Flours and Starches of Eight Brazilian Maize Landraces (Zea Mays L.). Food Hydrocolloids 2013, 30, 614–624.
- Ali, A.; Wani, T.A.; Wani, I.A.; Masoodi, F.A. Comparative Study of the Physico-Chemical Properties of Rice and Corn Starches Grown in Indian Temperate Climate. Journal of the Saudi Society of Agricultural Sciences 2014. DOI:10.1016/j.jssas.2014.04.002
- Beckles, D. M.; Thitisaksakul, M. How Environmental Stress Affects Starch Composition and Functionality in Cereal Endosperm. Starch/Stärke 2014, 66, 58–71.
- Lu, D.; Cai, X.; Shi, Y.; Zhao, J.; Lu, W. Effects of Waterlogging After Pollination on the Physicochemical Properties of Starch from Waxy Maize. Food Chemistry 2015, 179, 232–238.
- Sui, Z.; Yao, T.; Zhao, Y.; Ye, X.; Kong, X.; Ai, L. Effects of Heat–Moisture Treatment Reaction Conditions on the Physicochemical and Structural Properties of Maize Starch: Moisture and Length of Heating. Food Chemistry 2015, 173, 1125–1132.
- Li, X.; Wang, C.; Lu, F.; Zhang, L.; Yang, Q.; Mu, J.; Li, X. Physicochemical Properties of Corn Starch Isolated by Acid Liquid and L-Cysteine. Food Hydrocolloids 2015, 44, 353–359.
- Mir, S.A.; Bosco, S.J.D. Cultivar Difference in Physicochemical Properties of Starches and Flours from Temperate Rice of Indian Himalayas. Food Chemistry 2014, 157, 448–456.
- Sun, Q.; Zhu, X.; Si, F.; Xiong, L. Effect of Acid Hydrolysis Combined with Heat Moisture Treatment on Structure and Physicochemical Properties of Corn Starch. Journal of Food Science and Technology 2015, 52, 375–382.
- Nadiha, M.Z.N.; Fazilah, A.; Bhat, R.; Karim, A.A. Comparative Susceptibilities of Sago, Potato and Corn Starches to Alkali Treatment. Food Chemistry 2010, 121, 1053–1059.
- Jiranuntakul, W.; Puttanlek, C.; Rungsardthong, V.; Puncha-arnon, S.; Uttapap, D. Microstructural and Physicochemical Properties of Heat-Moisture Treated Waxy and Normal Starches. Journal of Food Engineering 2011, 104, 246–258.
- AACC. Approved Methods of the AACC, 8th Ed; American Association of Cereal Chemists: St Paul, MN, 2000.
- Williams, P.C.; Kuzina, F.D.; Hlynka, I. A Rapid Colorimeteric Procedure for Estimating the Amylose Content of Starches and Flours. Cereal Chemistry 1970, 47, 411–420.
- Wang, L.; Xie, B.; Shi, J.; Xue, S.; Deng, Q.; Wei, Y.; Tian, B. Physicochemical Properties and Structure of Starches from Chinese Rice Cultivars. Food Hydrocolloids 2010, 24, 208–216.
- Sandhu, K.S.; Singh, N.; Kaur, M. Characteristics of the Different Corn Types and Their Grains Fractions: Physicochemical, Thermal, Morphological, and Rheological Properties of Starch. Journal of Food Engineering 2004, 64, 119–127.
- Martínez, M.M.; Calviño, A.; Rosell, C.M.; Gómez, M. Effect of Different Extrusion Treatments and Particle Size Distribution on the Physico-Chemical Properties of Rice Flour. Food Bioprocess Technology 2014, 7, 2657–2665.
- Pukkahuta, C.; Suwannawat, B.; Shobsngob, S.; Varavinit, S. Comparative Study of Pasting and Thermal Transition Characteristics of Osmotic Pressure and Heat-Moisture Treated Corn Starch. Carbohydrate Polymers 2008, 72, 527–536.
- Vignaux, N.; Doehlert, D.C.; Elias, E.M.; McMullen, M.S.; Grant, L.; Kianian, S.F. Quality of Spaghetti Made from Full and Partial Waxy Durum Wheat. Cereal Chemistry 2005, 82, 93–100.
- Adebooye, O.C.; Singh, V. Physico-Chemical Properties of the Flours and Starches of Two Cowpea Varieties (Vigna Unguiculata (L.) Walp). Innovative Food Science and Emerging Technologies 2008, 9, 92–100.
- Tester, R.F.; Morrison, W.R. Swelling and Gelatinization of Cereal Starches. I. Effects of Amylopectin, Amylose and Lipids. Cereal Chemistry 1990, 67, 551–557.
- Amaya-Llano, S.L.; Martínez-Bustos, F.; Alegría, A.L.M.; Zazueta-Morales, J.J. Comparative Studies on Some Physico-Chemical, Thermal, Morphological, and Pasting Properties of Acid-Thinned Jicama and Maize Starches. Food Bioprocess Technology 2011, 4, 48–60.
- Suma. P.F.; Urooj, A. Isolation and Characterization of Starch from Pearl Millet (PennisetumTyphoidium) flours. International Journal of Food Properties 2015, 18, 2675–2687.
- Lopez, O.V.; Zaritzky, N.E.; Garcia, M.A. Physicochemical Characterization of Chemically Modified Corn Starches Related to Rheological Behavior, Retrogradation and Film Forming Capacity. Journal of Food Engineering 2010, 100, 160–168.
- Cael, J.J.; Koenig, J.L.; Blackwell, J. Infrared and Raman Spectroscopy of Carbohydrates, Part III: Raman Spectra of the Polymorphic Forms of Amylose. Carbohydrate Research 1973, 29, 123–134.
- Yang, L.; Zhang, L.M. Chemical Structural and Chain Conformational Characterization of Some Bioactive Polysaccharides Isolated from Natural Sources. Carbohydrate Polymers 2009, 76, 349–361.
- Liu, Y.L.; Himmelsbach, D.S.; Barton, F.E. Two-Dimensional Fourier Transform Raman Correlation Spectroscopy Determination of the Glycosidic Linkages in Amylose and Amylopectin. Applied Spectroscopy 2004, 58, 745–749.
- Passauer, L.; Bender, H.; Fischer, S. Synthesis and Characterisation of Starch Phosphates. Carbohydrate Polymers 2010, 82, 809–814.
- Fan, D.; Ma, W.; Wang, L.; Huang, J.; Zhao, J.; Zhang, H.; Chen, W. Determination of Structural Changes in Microwaved Rice Starch Using Fourier Transform Infrared and Raman Spectroscopy. Starch/Starke 2012, 64, 598–606.
- Flores-Morales, A.; Jiménez-Estrada, M.; Mora-Escobedo, R. Determination of the Structural Changes by FT-IR, Raman, and CP/MAS 13C NMR Spectroscopy on Retrograded Starch of Maize Tortillas. Carbohydrate Polymers 2012, 87, 61–68.
- Li, H.; Liu, G.; Zhang, G.; Hu, B.; Yu, D.; Chen, S. The Latest Progress in Study on Starch-Based Adhesives. Chemical Adhesion 2008, 5, 50–53.
- Ketthaisong, D.; Suriharn, B.; Tangwongchai, R.; Lertrat, K. Changes in Physicochemical Properties of Waxy Corn Starches at Different Stages of Harvesting. Carbohydrate Polymers 2013, 98, 241–248.
- Yu, S.; Ma, Y.; Menager, L.; Sun, D.W. Physicochemical Properties of Starch and Flour from Different Rice Cultivars. Food and Bioprocess Technology 2012, 5, 626–637.
- Perera, C.; Hoover, R. Influence of Hydroxypropylation on Retrogradation Properties of Native, Defatted and Heat-Moisture Treated Potato Starches. Food Chemistry 1999, 64, 361–375.
- Abegunde, O.K.; Mu, T.H.; Chen, J.W.; Deng, F.U. Physicochemical Characterization of Sweet Potato Starches Popularly Used in Chinese Starch Industry. Food Hydrocolloids 2013, 33, 169–177.
- Jacobson, M.R.; Obanni, M.; BeMiller, J.N. Retrogradation of Starches from Different Botanical Sources. Cereal Chemistry 1997, 74, 511–518.
- Sandhu, K.S.; Kaur, M.; Singh, N.; Lim, S.T. A Comparison of Native and Oxidized Normal and Waxy Corn Starches: Physicochemical, Thermal, Morphological and Pasting Properties. LWT–Food Science and Technology 2008, 41, 1000–1010.
- Yamin, F.F.; Lee, M.; Pollak, L.M.; White, P.J. Thermal Properties of Starch in Corn Variants Isolated After Chemical Mutagenesis of Inbred Line B73 1. Cereal Chemistry 1999, 76, 175–181.