ABSTRACT
The purpose of this research is the evaluation of a flaxseed oil-in-water emulsion, stabilized by a multi-layer structure consisting of sodium caseinate (Na-caseinate) and pectin to provide a basis for the combination of these materials for future studies. In the first step, the o/w emulsion (10 g oil, 90 g aqueous phase, and a pH 6.8) with varying concentration of Na-caseinate was investigated. Second, the pectin solution (0.05–1.5 g/100 g solution) was added to the primary emulsions and the pH was adjusted to 3.0. The emulsions were characterized by mean particle size (dynamic light scattering and static light scattering techniques), ζ-potential, turbidity value, creaming index, and the visualization of the microstructure. A clear separation of the oil phase at low protein contents and destabilizing by depletion flocculation at high protein content were observed. Extensive droplet flocculation and coalescence were determined until the pectin concentration reached 0.5 g/100 g solution for the secondary emulsion. After 7 days of storage, a 1.5 g/100 g solution pectin content had good stability with a relatively small size distribution, high turbidity value, and no cream phase separation.
Introduction
Emulsion based food products play an important role in the food industry by representing a considerable number of products such as cream, soups, sauces, mayonnaise, salad dressings, ice cream, coffee butter, margarine, dessert, etc. Moreover, the emulsion-based delivery systems are a very promising procedure for many practical applications concerning different functional food ingredients.[Citation1–Citation3] Over the past decade, an important new tendency has emerged to improve the functionality of the traditional emulsion systems. The need for additional progress in the stabilization of emulsion droplets against environmental stress factors, such as pH change, salt concentration, thermal treatments (heating, freezing, chilling, and dehydration), high mineral concentration, and current limitations in the type of emulsifiers and production methods are the main driving forces for novel approaches in this field.[Citation4–Citation7] A multi-layer oil-in-water (o/w) emulsion is an alternative strategy for improving interfacial limitations of traditional emulsion systems and contains oil droplets being surrounded by multiple layers constituted with the layer-by-layer (LBL) electrostatic deposition technique.[Citation1,Citation8–Citation12] The stabilizing mechanism of emulsion systems is based on the characteristics of the interfacial layer between the surface of the droplets and the surrounding continuous phase. The LBL technique aims to manipulate the structural properties (chemical composition, net electrical charge, thickness, permeability, and rheology) and reorganize the colloidal interactions for changing the environmental responsiveness of emulsions.[Citation13] For the first step of the LBL method, a charged surface is formed by an ionic emulsifier around the lipid droplets through homogenization. This primary emulsion is used as the basic layer for the oppositely charged polyelectrolyte which is added to the system and adsorbed onto the droplet surfaces. Thus, the secondary emulsion structure containing oil droplets coated with a two-layer interface is produced. Multi-layer interfacial structures can be obtained by using this step repetitively for three or more layers.
In this study, the influence of protein and the biopolymer contents on the stability of multi-layer o/w emulsions was investigated. The primary emulsion, prepared with Na-caseinate and citrus pectin, was used for the multi-layer structure using the LBL technique. The multi-layer emulsion theory assumes that the primary emulsion is stable to aggregation before the addition of the polyelectrolyte.[Citation13] For this reason, first step of the research was the determination of the optimum emulsifier content for stabilizing the primary structure. The second step was the determination for the amount of biopolymer which would be sufficient to saturate all the particle surfaces completely and form a stable multi-layer structure.
Materials and methods
Materials
The high methoxyl pectin (HMP; 71% degree of esterification) was obtained from Yantai Andre Pectin (China). The powdered sodium caseinate was obtained from BBA Lactalis Industrie (88% protein content, 8% moisture, 1.2 ± 0.25% sodium, and 0.06–0.12% calcium; France). The flaxseed oil was purchased from a local producer as a cold press production. The sodium phosphate monobasic dihydrate was purchased from Sigma-Aldrich and the di-sodium hydrogen phosphate dodecahydrate was purchased from Carlo Erba. The analytical grade hydrochloric acid (HCl) was purchased from Merck and the sodium hydroxide (NaOH) was purchased from Honeywell Riedel-de Haen®. Deionized water was used for the preparation of all solutions.
Stock Solution Preparation
A Na-caseinate stock solution (2 g/100 g solution) was prepared by dispersing weighed amounts of Na-caseinate into a 10 mM sodium phosphate buffer solution (pH 6.8), then stirring for 7 h for complete hydration and kept at +4°C until utilization. The pectin stock solution (3 g/100 g solution) was prepared in a similar way with a Na-caseinate solution. But, a pH adjustment (pH 6.8) was necessary with 1 M NaOH for this solution.
Primary emulsion preparation
The preparation of a primary emulsion that was stable against droplet aggregation was the main purpose of the preliminary experiment. The determination of the optimum Na-caseinate amount for the o/w emulsion was performed by preparing a diluted Na-caseinate stock solution with a phosphate buffer solution (10 mM, pH 6.8) in various concentrations (0.1–1.5 g/100 g solution in the final o/w emulsion). Then, the pH values of all the diluted solutions were adjusted to a pH 6.8 with a 0.1 M NaOH solution. The flaxseed oil (10 wt%) was mixed with the diluted Na-caseinate solutions with gentle stirring at room temperature. The solution (10 wt% oil, and 90 wt% aqueous emulsifier solution) was pre-homogenized with a high speed blender (7011S, Waring Commercial blender, USA) under 22,000 rpm for 3 min, followed by five passes at 10,000 psi through a high-pressure microfluidizer (M-110L, Microfluidics, USA). The Na-caseinate stabilized emulsions were stored at +4°C for 24 h before being analyzed. The optimum Na-caseinate concentration that was necessary for covering all the oil particles in the continuous phase and stabilized droplets against aggregation was established as 0.7 g/100 g solution. This protein amount was used to prepare the primary emulsions in the following experiments.
Secondary emulsion preparation
A primary emulsion (10 wt% flaxseed oil, 0.7 wt% Na-caseinate, and pH 6.8) was prepared by pre-homogenizing with a high-speed blender (7011S, Waring Commercial blender, USA) under 22,000 rpm for 3 min, followed by five passes at 10,000 psi through a high-pressure microfluidizer (M-110L, Microfluidics, USA). This emulsion was diluted with gently stirring with a pectin stock solution (pH 6.8) and phosphate buffer solution (pH 6.8) to produce a series of secondary emulsions with different pectin concentrations (5 wt% flaxseed oil, 0.35 wt% Na-caseinate, and 0.05–1.5 g/100 g solution pectin). After adding the pectin stock solution to the primary emulsions, the pH was adjusted to 3.0 with a 1 M HCl solution and the emulsions were stirred for 30 minutes using a magnetic stirrer at ambient temperature. The multi-layer emulsions were stored at +4°C for 24 h before being analyzed. The mixing of oil droplets and pectin at pH values where they had similar charges (pH 6.8) provides more dispersed pectin molecules throughout the aqueous phase before adsorption. Then adjusting the pH to a value where they had opposite charges leads to a further stable secondary emulsion to flocculation.[Citation14]
Particle size determination
The mean particle size was measured using a dynamic light scattering (DLS) technique, with a Zetasizer Nano-ZS (Malvern Instruments, Worcestershire, UK) and a static light scattering (SLS) technique using a Mastersizer 2000 (Malvern Instruments, Worcestershire, UK) because of the limits of their particle size ranges. The primary emulsions were diluted 1/100 (v/v) using a phosphate buffer solution with a pH 6.8 to avoid multiple scattering effects in the application of DLS. The DLS measurement is based on the Brownian motion of particles in a suspension which causes laser light to be scattered at different intensities. The size of the particles is calculated with the Stokes-Einstein equation. The results were obtained as z-average diameter and polydispersity index (the width of the distribution).
The secondary emulsions were diluted at approximately 1/1000 (v/v) using distilled water at a pH 3.0 in the application of SLS. The SLS technique utilizes the relationship between the intensity of light scattered by a molecule and its molecular weight and size to measure absolute molecular weight. The particle size measurement are reported as the area-volume mean diameter (d[Citation3,Citation2] = ∑nidi3 /∑nidi2) and the volume fraction-length mean diameter (d[Citation4,Citation3] = ∑nidi4 /∑nidi3), where ni is the number of particles and di is the diameter of particles. The refractive index for the oil phase and continuous phase were 1.48 and 1.333, respectively. The measurements were carried out at the ambient temperature. The average and standard deviation of measurements for the particle size analysis were calculated twice for each of the two freshly prepared samples, with three readings made per sample.
ζ-Potential measurements
The analysis was performed with a Zetasizer Nano-ZS, Malvern Instrument, after 24 h of storage at +4°C. The primary and secondary emulsions were diluted (1/100 v/v) using a phosphate buffer solution prior to analysis (at the same pH as the samples) to avoid multiple scattering effects. The ζ-potential provides the electrical potential at the shear plane of the particles. The magnitude and sign of the electrical charge of particle surfaces plays an important role in emulsion stability.[Citation15] The ζ-potential measurement was determined from the average of three readings taken for each samples.
Turbidity measurement
The turbidity measurement was carried out using a Cary 50 Scan UV-Vis spectrophotometer (Varian Inc., California, USA). The primary and secondary o/w emulsions were stored at ambient temperature for 7 days and then, diluted using a phosphate buffer solution (1:500 v/v) at the same pH as the samples. The turbidity values were determined by the absorbance at 660 nm.[Citation16] The turbidity of the emulsions depends principally on the size and concentration of the particle, hence, high turbidity values can represent less coagulation or coalescence in the structure of the emulsions.[Citation17]
Creaming stability measurement
After the primary Na-caseinate emulsions and secondary Na-caseinate+pectin emulsions were prepared, 10 ml of each sample were transferred to glass cylinder tubes immediately and tightly sealed with a plastic cap. After 7 days of storage at an ambient temperature, separated layers were observed. A clear oil phase separation in the case of the primary emulsions of the lower Na-caseinate concentration was determined. Also, an optically opaque cream layer at the top and a transparent, or turbid serum layer, at the bottom were observed in the case of the secondary emulsion samples. The creaming stability index was calculated using the following equation:
HS represents the height of the serum layer (the sum of the turbid and transparent layers at the bottom of the samples) and the HT represents the height of the total emulsion that was directly measured with a ruler.[Citation18] The creaming index is an indicator for gravitational separation arising from droplet aggregation. An increase in the creaming index provides indirect information about the emulsion instability.
Optical microscopy
After 7 days of storage, the structure of the selected primary and secondary o/w emulsion samples were observed using an optical microscope (Olympus-CX31, Olympus Inc., Japan). The emulsions were gently agitated in glass tubes before analysis.
Statistical analysis
Experiments were performed twice using two freshly prepared samples. The averages and standard deviations were calculated from these measurements using SPSS (IBM, SPSS Statistics, Version 20).
Results
Determination of NA-caseinate concentration for primary emulsion
Our first objective was to determine the appropriate Na-caseinate concentration to obtain a stable and homogenous primary emulsion structure (10 wt% flaxseed oil, 90 wt% aqueous phase, and a pH 6.8). indicates that while the Na-caseinate concentrations were 0.1 wt% and 0.3 wt%, the z-average diameter values were 0.71 ± 0.27 µm and 0.30 ± 0.053 µm, respectively. Also, the polydispersity index was measured at 0.62 ± 0.15 and 0.42 ± 0.12 at a pH 6.8. At the higher Na-caseinate concentrations (0.4– 1.5 wt %), the particle size of the droplets decreased from around 0.3 µm to 0.2 µm and their stabilities were independent of the protein content. It has been reported that the protein stabilized emulsions, which have insufficient protein concentration to cover oil droplet surfaces, are prone to droplet association and particle aggregation. The size of the emulsion droplets is determined by the limited protein at this phase and larger particles may be formed as a result of coalescence or bridging flocculation. Further increasing the amount of protein leads to saturated of oil droplet surfaces and also, a decrease in particle size distribution is observed. In this phase, the particle size distribution is governed primarily by the homogenization pressure.[Citation19,Citation20] The ζ-potential of the Na-caseinate coated droplets increased from –43.4 to –47.6 mV at a pH 6.8 parallel to the increase in the Na-caseinate concentration (0.1–0.5 wt%). After 1 wt% concentration, the ζ-potential values remained stable (data not shown). The electrical charge of the oil droplets normally originates from the adsorption of the emulsifier molecules on the droplet surfaces. The isoelectric point (pI) of the Na-caseinate is nearly a pH 4.6 and above this pH, the oil particles which are covered with emulsifier had a negative value which was expected. After a certain concentration, the ζ-potential remained relatively constant based on the particle layer saturation with the emulsifier. Hu et al.[Citation21] reported that the o/w emulsion prepared with corn oil and Na-caseinate had a decreasing particle size with an increasing protein concentration (0.2–1.5 wt%) and a monomodal emulsion structure was obtained with 1.5 wt% Na-caseinate. Also, after a 0.5 wt% Na-caseinate concentration, the ζ-potential remained stable with an increasing protein concentration. This result showed that the droplet surfaces were saturated with protein even at the lowest protein concentration used. Day et al.[Citation19] found that the protein content from 0.5 to 1.0 wt% had no effect on the alteration of the size distribution of the fish o/w emulsion.
Figure 1. A) The effect of different Na-caseinate concentrations (wt%) on Z-average diameter (μm) and polydispersity index of primary Na-caseinate stabilized emulsion (10 wt% flaxseed oil, 90 wt% aqueous phase) at pH 6.8. Data represents mean (n = 4) ± standard derivations. Some error bars lay within data points B) Optical microscope photographs from a series of 10% flaxseed oil at different Na-caseinate concentrations; a) 0.1 wt% b) 0.4 wt% c) 0.7 wt% d) 1.5 wt% after 7 days storage at the ambient temperature.
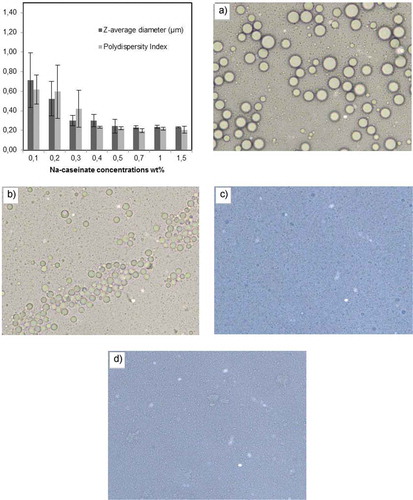
Light scattering techniques assumes that all emulsion particles are isolated and homogenous spheres. Therefore, it is not possible to differentiate the larger particles that may be formed as a result of coalescence or bridging flocculation. Also, only diluted samples can be used in this technology for avoiding multiple scattering effects and diluting may cause appreciable alterations in particle size distribution through the breaking of the flocculated droplets.[Citation15] Therefore, imaging with optical microscopy is a necessary next step for understanding the structure of the Na-caseinate stabilized emulsions. shows the primary emulsions after 7 days storage at ambient temperature. Emulsions containing low concentration of Na-caseinate have a broad size distribution and flocculated regions because of insufficient emulsifier and particle surface cover. When the emulsifier concentration was increased to a medium level, it was observed that the oil particle size was getting smaller with a homogeneous particle size distribution. However, a higher amount of Na-caseinate partly caused a depletion flocculation in the emulsion structure.[Citation22] Moreover, after 7 days of storage, the turbidity values and creaming index were determined for the o/w emulsions containing different Na-caseinate concentrations. The turbidity value was measured as 0.38 ± 0.0045 and 0.54 ± 0.007 at low protein concentrations (0.1–0.3 wt%) while it increased to 0.66 ± 0.001 at a medium level of protein (0.7 wt%). This increase was indicative of a structure containing small and distributed particles throughout the sample cell with a sufficient amount of emulsifier (). If the Na-caseinate concentration continued to increase (1.0–1.5 wt%), an important decline was observed (0.44 ± 0.01 and 0.4 ± 0.002, respectively) and it was indicative of a structure losing its stability and containing large particles that slowly moved upward.[Citation23] In addition, the results of the creaming index showed a regular decline with the increase in the Na-caseinate concentration ().
Figure 2. shows a) turbidity value (data represents mean (n = 4) ± standard derivations. Some error bars lay within data points) b) creaming index of a series of 10% flaxseed oil at different Na-caseinate concentrations (0.1–1.5 wt%) after 7 days storage at the ambient temperature.
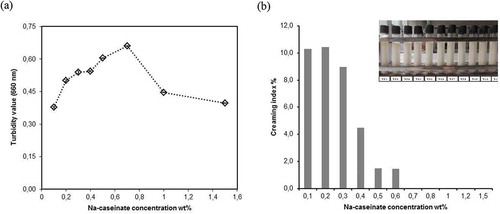
Creaming is one of the gravitational separation forms which is an upward movement of droplets having lower density than the continuous phase. Creaming can cause a contact between the droplets for an extended period of time and lead to an enhanced coalescence that forms an oiling off structure as an oil layer on top of the emulsion later on in the storage period. At the lower concentration of Na-caseinate (0.1–0.4 wt%), a clear oiling off layer was observed in the o/w primary emulsions (). All measurements indicated that the o/w emulsion containing 10% flaxseed oil and an addition of a medium level of Na-caseinate (0.7 wt%) provided a higher emulsion stability and resistance against creaming at a pH 6.8. After this concentration, the system may contain instability indicators such as depletion flocculation () and larger particle formation () after 7 days of storage. Rasnani et al.[Citation16] selected a 0.5 wt% Na-caseinate concentration for preparing a stable multi-layer emulsion with 10% canola oil at various pH levels. Surh et al.[Citation18] utilized 1 wt% Na-caseinate for the primary emulsion (10 wt % corn oil with a 90 wt% aqueous Na-caseinate solution) at a pH 7.0. Srinivasan, Singh, and Munro[Citation24] evaluated the creaming stability of the o/w emulsion (30 wt% oil) prepared with a sodium and calcium caseinate. According to the obtained results, low concentrations of Na-caseinate (0.5% and 1.0%) could stabilize the emulsion with no flocculation. Day et al.[Citation19] also reported that excessive Na-caseinate such as 1 wt% in an o/w emulsion (25 wt% fish oil) caused the aggregation of oil droplets through depletion flocculation and a lower Na-caseinate concentration such as 0.1 wt% caused bridging flocculation but showed good stability to creaming. Our research results obtained are in line with these previous studies.
Formation of multi-layer emulsion structure using NA-caseinate stabilized emulsion and pectin
The aim of these experiments was to determine the optimum concentration of pectin which is necessary to stabilize a secondary emulsion. Addition of different pectin concentrations (0.05–1.5 wt%) to the primary emulsion (10 wt% flaxseed oil, 0.7 wt% Na-caseinate, and 10 mM phosphate buffer) was carried out at a pH 6.8 and then the pH was adjusted to 3.0 with continuously stirring. The d(4,3) and d(3,2) values were measured against the pectin concentrations after 24 h are shown in and . When the pectin concentration was increased from 0.05 to 0.15 wt%, the d(4,3) values were 16.2 ± 0.9 and 57.4 ± 1.9 µm, and the d(3,2) values were 3.9 ± 0.6 and 22.6 ± 1.2 µm, respectively. The net charge on the droplets was nearly zero by reason of charge neutralization which occurred around 0.15 wt% pectin (). Insufficient repulsive forces between oil droplets could cause a strong droplet aggregation and the dilution effect was not enough to break it at this range. At relatively low polymer concentrations, bridging flocculation is also common in protein stabilized emulsion containing biopolymers such as pectin, chitosan, and carrageenan.[Citation10,Citation12,Citation25] If the pectin concentration continued to increase to 0.5 wt%, the d(4,3) and d(3,2) values were observed as 10.65 ± 2.92 and 2.0 ± 1.2 µm and after this concentration both the d(4,3) and d(3,2) values remained close to these values. Nevertheless, the ζ-potential of the droplets changed from positive to negative as the pectin concentration in the emulsions was increased at a pH 3.0 (). These measurements indicated that the negatively charged pectin molecules were adsorbed onto the surface of the positively charged Na-caseinate covered droplets and it was responsible for the net charge alteration. This kind of charge reversal which results from the adsorption of a charged polyelectrolyte to the surface of the oppositely charged colloidal particles was found in previous studies.[Citation4,Citation26–Citation28] In the absence of pectin, the ζ-potential was measured as 26.5 ± 6.6 mV because of the positively charged Na-caseinate molecules at a pH 3.0. When the pectin concentration reached 0.5 wt%, the ζ-potential reached – 11.2 ± 1.2 mV as a consequence of adsorption.
Figure 3. shows a) d(4,3) b) d(3,2) and c) ζ-potential values of the secondary emulsion (5 wt% flaxseed oil, 0.35 wt% Na-kazeinate, 0–1.5 wt% pectin, pH 3.0) aganist different pectin concentration. Data represents mean (n = 4) ± standard derivations. Some error bars lay within data points.
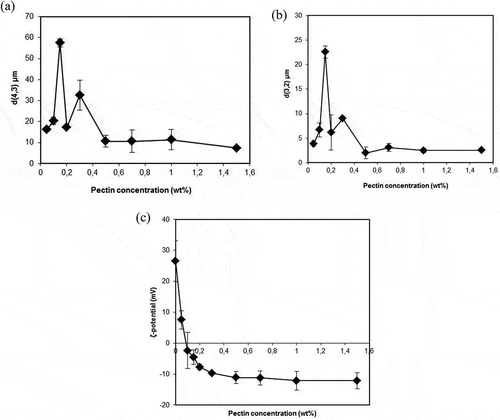
After 7 days of storage at ambient temperature, and show the turbidity value and creaming index of the secondary emulsions of the different pectin concentrations. The turbidity value of the secondary emulsions were 0.11±0.002, 0.28±0.002 and 0.63±0.001 with an increasing pectin concentration (0.05, 0.5, and 1.5 wt%) and the highest value was measured at a 1.5 wt% pectin content. These results were in close agreement with the creaming index measurements. In addition, a clear separation of the cream layer at the top and a transparent (or turbid) serum layer at the bottom were observed between 0.05–0.5 wt% pectin concentrations () while the primary emulsion with no pectin was preserving its stability until the end of storage. The cream layer separation because of extensive aggregation is based on the charge neutralization and bridging effect concerning an insufficient biopolymer content which leads to a decrease in absorbance.[Citation17]
Figure 4. shows a) turbidity value (data represents mean (n = 4) ± standard derivations. Some error bars lay within data points) b) creaming index of the secondary emulsion (5 wt% flaxseed oil, 0.35 wt% Na-kazeinate, 0-1.5 wt% pectin, pH 3.0) aganist different pectin concentration.
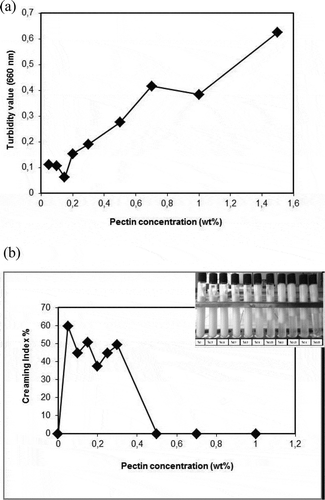
The optical microscope photographs illustrated that there was a strong bridging flocculation and coacervation at these concentrations (). When the pectin concentration reached 1.5 wt% it was observed that most of the flocculations were broken and that a relatively stable and uniform particle size distribution was achieved.
Figure 5. Optical microscope photographs from a series of secondary emulsion with varying pectin concentrations; a)0.1 wt% b)0.3 wt% c)0.5 wt% d)1.0 wt% e)1.5 wt% after 7 days storage at the ambient temperature.

Katsuda et al.[Citation29] reported that a stable multi-layer emulsion (0.1% Na-caseinate, 1% fish oil, and different amounts of citrus pectin) could be obtained between 0.1–0.4 wt% citrus pectin concentrations. Moreau et al.[Citation30] studied the emulsions stabilized by β-lactoglobulin and pectin layers at a pH 3.0. According to their results, the net charge of the droplets reached a constant negative value when the pectin concentration exceeded 0.1 wt%. However, when the pectin concentration increased (0.08–0.2 wt%), that a large increase was observed in the mean particle diameter d(3,2) because of the adsorption of pectin molecules to the surface of more than one droplet. According to Mao et al.[Citation31] a multi-layer o/w emulsion (10 wt% oil) with whey protein isolate (WPI) and pectin showed stability when the pectin concentration reached 0.8 wt% at a pH 5, 4, and 3.
According to our research results, the optimum pectin concentration determined for a stable multi-layer structure (1.5 wt%) is relatively high compared with previous studies. One of the reason may be the relatively smaller droplet size of the primary emulsion (nearly 0.23 µm) obtained in our study and the increased surface area of the droplets. The oil-emulsifier ratio, type of pectin (citrus, apple, or sugar beet), and the degree of esterification of pectin are other possible reasons which may affect the biopolymer amount.[Citation18,Citation32]
Conclusions
The purpose of this study was the determination of the polyelectrolyte concentrations for preparing a multi-layer emulsion structure stabilized by Na-caseinate and pectin. In the first step, a primary o/w emulsion was produced through the homogenization of flaxseed oil and Na-caseinate at a pH 6.8. It was a necessary step to avoid an excessive or modest protein content which affects the stability of the multi-layer structure before the addition of a biopolymer. In the next step, a secondary emulsion was produced by mixing a varying content of pectin solutions with the primary emulsion at a pH 6.8 and then adjusting the pH to 3.0. The adsorption through electrostatic attraction occurred between the positively charged droplets and negatively charged pectin at this pH. The primary emulsion which was prepared with 0.7 wt% protein showed a small particle size distribution (0.23 ± 0.01 µm) and a good stability to creaming after 7 days of storage. Below this concentration a clear flocculation, coalescence, and finally the oil-off structure were observed. Moreover, a depletion flocculation was detected in the microscopic examination of higher concentrations (1 and 1.5 wt%). The secondary emulsion exhibited a small particle size distribution (7.3 ± 0.76 µm for d[Citation4,Citation3] and 2.6 ± 0.37µm for d [Citation3,Citation2]) and a high ζ-potential value at 1.5 wt% pectin concentration. A strong bridging flocculation was observed in the optical microscope photographs until the pectin concentration reached 1.5 wt%. The oil droplets that had a partial surface coverage contributed to the particle aggregation and showed a cream phase separation after 7 days. This study showed that a stable multi-layer emulsion can be prepared using flaxseed oil, Na-caseinate, and pectin at a pH 3.0. But further studies are necessary to understand the interfacial characteristics of the multi-layer membrane under changing environmental stress factors and its protective effect against oxidative deterioration of the flaxseed oil for this protein and polymer combination.
Acknowledgment
We thank the Izmir Institute of Technology (IYTE), Biotechnology and Bioengineering Application and Research Center (BIOMER) for the optical microscopy analysis.
References
- Grigoriev, D.O.; Miller, R. Mono and Multilayer Covered Drops as Carriers. Current Opinion in Colloid & Interface Science 2009, 14(1), 48–59.
- McClements, D.J.; Decker, E.A.; Weiss, J. Emulsion-Based Delivery Systems for Lipophilic Bioactive Components. Journal of Food Science 2007, 72(8), 109–124.
- Preetz, C.; Rübe, A.; Reiche, I.; Hause, G.; Mäder, K. Preparation and Characterization of Biocompatible Oil-Loaded Polyelectrolyte Nanocapsules. Nanomedicine: Nanotechnology, Biology, and Medicine 2008, 4, 106–114.
- Aoki, T.; Decker, E.A.; McClements, D.J. Influence Of Environmental Stresses on Stability of O/W Emulsions Containing Droplets Stabilized by Multilayered Membranes Produced by a Layer-by-Layer Electrostatic Deposition Technique. Food Hydrocolloids 2005, 19, 209–220.
- Guzey, D.; McClements, D.J. Formation, Stability and Properties of Multilayer Emulsions for Application in the Food Industry. Advances in Colloid and Interface Science 2006, 128–130, 227–248.
- McClements, D.J.; Decker, E.A.; Park, Y.; Weiss, J. Structural Design Principles for Delivery of Bioactive Components in Nutraceuticals and Functional Foods. Critical Reviews in Food Science and Nutrition 2009, 49, 577–606.
- Ogawa, S.; Decker, E.A.; McClements, D.J. Production and Characterization of O/W Emulsions Containing Cationic Droplets Stabilized by Lecithin-Chitosan Membranes. Journal of Agricultural and Food Chemistry 2003, 51, 2806–2812.
- Gu, Y.S.; Decker, E.A.; McClements, D.J. Influence of pH and I-Carrageenan Concentration on Physicochemical Properties and Stability of β-Lactoglobulin-Stabilized Oil-in-Water Emulsions. Journal of Agricultural and Food Chemistry 2004, 52, 3626–3632.
- Hu, M.; Li, Y.; Decker, E.A.; Xiao, H.; McClements, D.J. Impact of Layer Structure on Physical Stability and Lipase Digestibility of Lipid Droplets Coated by Biopolymer Nanolaminated Coatings. Food Biophysics 2011, 6, 37–48.
- Liu, J.; Verespej, E.; Alexander, M.; Corredig, M. Comparison on the Effect of High-Methoxyl Pectin or Soybean-Soluble Polysaccharide on the Stability of Sodium Caseinate-Stabilized Oil/Water Emulsions. Journal of Agricultural and Food Chemistry 2007, 55, 6270–6278.
- Ogawa, S.; Decker, E.A.; McClements, D.J. Influence of Environmental Conditions on the Stability of Oil in Water Emulsions Containing Droplets Stabilized by Lecithin-Chitosan Membranes. Journal of Agricultural and Food Chemistry 2003, 51, 5522–5527.
- Perrechil, F.A.; Cunha, R.L. Stabilization of Multilayered Emulsions by Sodium Caseinate and K-Carrageenan. Food Hydrocolloids 2013, 30, 606–613.
- McClements, D.J. Theoretical Analysis of Factors Affecting the Formation and Stability of Multilayered Colloidal Dispersions. Langmuir 2005, 21, 9777–9785.
- Guzey, D.; Kim H.J.; McClements, D.J. Factors Influencing the Production of O/W Emulsions Stabilized by Beta-Lactoglobulin-Pectin Membranes. Food Hydrocolloids 2004, 18(6), 967–975.
- McClements, D.J. Food Emulsions Principles, Practices, and Techniques, 2nd Ed; CRC Press; Boca Raton, FL, 2004; 632.
- Rasnani, N.M.; Mirhosseini, H.; Sham bin Baharin, B.; Tan, C.P. Effect of pH on Physicochemical Properties and Stability of Sodium Caseinate-Pectin Stabilized Emulsion. Journal of Food, Agriculture and Environment 2011, 9(1), 129–135.
- Anal, A.K.; Tobiassen A.; Flanagan, C.; Singh, H. Preparation and Characterization of Nanoparticles Formed by Chitosan–Caseinate Interactions. Colloids and Surfaces B: Biointerfaces 2008, 64, 104–110.
- Surh, J.; Decker, E.A.; McClements, D.C. Influence of pH and Pectin Type on Properties and Stability of Sodium-Caseinate Stabilized Oil-in-Water Emulsions. Food Hydrocolloids 2006, 20, 607–618.
- Day, L.; Xua, M.; Hoobin, P.; Burgar, I.; Augustin, M.A. Characterization of Fish Oil Emulsions Stabilised by Sodium Caseinate. Food Chemistry 2007, 105, 469–479.
- Dickinson, E.; Golding, M.; Povey, M.J.W. Creaming and Flocculation of Oil-in-Water Emulsions Containing Sodium Caseinate. Journal of Colloid and Interface Science 1997, 185, 515–529.
- Hu, M.; McClements, D.J.; Decker, E.A. Lipid Oxidation in Corn Oil-in-Water Emulsions Stabilized by Casein, Whey Protein Isolate, and Soy Protein Isolate. Journal of Agricultural and Food Chemistry 2003, 51, 1696–1700.
- Dickinson, E. Flocculation of Protein-Stabilized Oil-in-Water Emulsions. Colloids and Surfaces B: Biointerfaces 2010, 81, 130–140.
- Harnsilawat, T.; Pongsawatmanit, R.; McClements, D.J. Influence of pH and Ionic Strength on Formation and Stability of Emulsions Containing Oil Droplets Coated by β-Lactoglobulin-Alginate. Interfaces Biomacromolecules 2006, 7, 2052–2058.
- Srinivasan, M.; Singh, H.; Munro, P.A. Creaming Stability of Oil-in-Water Emulsions Formed with Sodium and Calcium Caseinates. Food Engineering and Physical Properties 2001, 66(3), 441–446.
- Li, Y.; Hub, M.; Xiao, H.; Dua, Y.; Decker, E.A.; McClements, D.J. Controlling the Functional Performance of Emulsion-Based Delivery Systems Using Multi-Component Biopolymer Coatings. European Journal of Pharmaceutics and Biopharmaceutics 2010, 76, 38–47.
- Chodanowski, P.; Stoll, S. Polyelectrolyte Adsorption on Charged Particles: Ionic Concentration and Particle Size Effects: A Monte Carlo Approach. Journal of Chemical Physics 2001, 115, 4951–4960.
- Kong, K.Y.; Muthukumar, M. Monte Carlo Study of Adsorption of a Polyelectrolyte onto Charged Spheres. Journal of Chemical Physics 1998, 109, 1522–1527.
- Zinoviadou, K.G.; Scholten, E.; Moschakis, T.; Biliaderis, C.G. Properties of Emulsions Stabilized by Sodium Caseinate-Chitosan Complexes. International Dairy Journal 2012, 26(1), 94–101.
- Katsuda, M.S.; Miglioranza, L.H.S.; McClements, D.J.; Decker, E.A. Fish Oil Emulsion Stabilized by Multilayer Membrane Composed by Casein and Pectin. CIGR-International Conference of Agricultural Engineering, 2008.
- Moreau, L.; Kim, H.; Decker, E.A.; Mcclements, D.J. Production and Characterization of Oil-in-Water Emulsions Containing Droplets Stabilized by β-Lactoglobulin-Pectin Membranes. Journal of Agricultural and Food Chemistry 2003, 51, 6612–6617.
- Mao, L.; Roos, Y.H.; O’Callaghan, D.J.; Miao, S. Volatile Release from Whey Protein Isolate—Pectin Multilayer Stabilized Emulsions: Effect of pH, Salt, and Artificial Salivas. Journal of Agricultural and Food Chemistry 2013, 61, 6231–6239.
- Katsuda, M.S.; McClements, D.J.; Miglioranza, L.H.S.; Decker, E.A. Physical and Oxidative Stability of Fish Oil-in-Water Emulsions Stabilized with β-Lactoglobulin and Pectin. Journal of Agricultural and Food Chemistry 2008, 56, 5926–5931.