ABSTRACT
In this study, the preparation of silver nanoparticles (Ag NPs) using Tribulus longipetalus Viv. leaf extract was evaluated. Additionally, the in vitro antioxidant and antibacterial activities of the extract were evaluated. The antioxidant activity was assessed by two different approaches based on 2,2-diphenyl-1-picryl-hydrazyl and reducing power assay. The antibacterial activity of the extract, on the other hand, was determined against gram positive and gram negative microorganisms. The AgNPs prepared under the optimal conditions were found to be 15 nm in size and were characterized by ultraviolet–visible spectroscopy, dynamic light scattering, X-ray diffraction and transmission electron microscopy.
Introduction
Nanostructures have been in the spotlight in recent years, because their properties mainly depend on their size and shape.[Citation1–Citation5] Nanoparticles of noble metals like silver and gold are widely used in every day products, like soaps, shampoos, shoes, cosmetic products, toothpaste, and detergents, which are in chronic direct contact with the human body. Further, these particles are used in medical and pharmaceutical formulations, drug delivery, tissue/tumor imaging, photothermal therapy, catalysis, optoelectronics, water purification, and biosensing applications.[Citation6–Citation9] Several physical and chemical methods such as laser ablation, lithography, chemical reduction, photochemical reduction, electrochemical reduction, and heat evaporation[Citation10–Citation12] have been used for the synthesis of noble metal nanoparticles with different shapes and sizes. Normally, various amounts of hazardous chemicals and solvents are required in the course of chemical routes used for the synthesis of fine and stable noble metal particles.[Citation13] On the other hand, in the case of physical synthetic procedures, there also exist problems due to the consumption of enormous amounts of energy for maintaining the high temperature and pressure conditions needed.[Citation6] Thus, the development and optimization of eco-friendly procedures, eliminating the use of toxic chemical reagent, for the synthesis nanoparticles is an essential need. To reach to this goal, biosynthesis procedures based on plant extracts or biological micro-organisms, like bacteria and fungi, have been used as alternatives to chemical methods.[Citation14–Citation16]
The application of plant extracts in the preparation of nanoparticles, through simply mixing them with solutions of metallic ions under ambient temperatures, has proven to offer advantages as compared to biological approaches. This is due to the fact that the presence of such extracts helps skip the measures taken to preserve cell cultures. These compounds tend to act as reducing agents in the synthesis of nanoparticles, yet they have also shown stabilizing and capping effects.[Citation17] Various extracts contain different amounts and ratios of compounds like terpenoids, flavonoids, phenol derivatives, hydrogenases, reductases, quinones, and their derivatives, di-hydric phenols,[Citation12] which provide the extracts with the capability of reducing metallic ions, in addition to capping properties, which allows them to restrict the dimensions of the produced particles to nanoscale ranges.[Citation18]
Given that a survey on the literature revealed no records on the evaluation of the antioxidant and antibacterial properties of T. longipetalus, the present work was directed toward the evaluation of the in vitro antioxidant and antibacterial activities of the extract of the leaves of this plant, as well as its application in a green synthesis procedure for the production of silver nanoparticles.
Materials and methods
Plant material
The leaves of T. longipetalus were collected in August 2015 from Kashan (Isfahan Province, Iran, with voucher specimen number of KBG 1450), and were dried in the shade (at room temperature). A voucher specimen of the plant was deposited at the Herbarium of Kashan botanical garden.
Chemicals
Linoleic acid, 2,6-di-tert-butyl-4-methylphenol (butylated hydroxytoluene [BHT]), 2,2-diphenyl-1-picrylhydrazyl (DPPH; 95%), gallic acid and β-carotene were procured from Sigma-Aldrich Chemie (Steinheim, Germany). Analytical grade methanol, ethanol, and dimethyl sulphoxide (DMSO), high-performance liquid chromatography (HPLC) grade chloroform, standard Folin–Ciocalteu’s phenol reagent, anhydrous sodium sulphate, ferric chloride, sodium carbonate, potassium ferricyanide, phosphate buffer solution (PBS), and Tween 40 were obtained from Merck (Darmstadt, Germany).
Preparation of the methanol extract
Thirty grams of the dried and powdered plant material were subjected to extraction with methanol in a Soxhlet apparatus at 60°C for 12 h. The extract was filtered and concentrated under vacuum at 40°C by a rotary evaporator (Heidolph, Laborota 4000, Schwabach, Germany), yielding a waxy material (1.2 g, 4.0% w/w), which was next suspended in water and subjected to extraction with chloroform (4 × 100 mL) to obtain 0.6 g (2.0%) of polar and 0.25 (0.8%) of non-polar extracts. The extract was next stored in the dark at 4°C, and used within a maximum period of 1 week.
Antioxidant properties
Scavenging capacity on DPPH radical
The free radical scavenging activities of the extracts were evaluated using DPPH as described by Gholivand et al.[Citation19,Citation20] According to this approach, 3 mL of various concentrations of the extract in methanol were added to 1 mL of a 0.5 mM methanol solution of DPPH. The mixture was vigorously shaken and left to stand at room temperature for 60 min in the dark. Then the absorbance of the samples was measured at 517 nm against a blank. Inhibition of free radical, DPPH, was calculated according to the following equation:
where Ab is the absorbance of the control reaction (containing all reagents except the test compound), and As is the absorbance of the test compound. The sample concentration leading to a 50% inhibition (IC50) was calculated from the plot of the inhibition percentage versus sample concentration. All experiments were repeated three times.
Reducing power
The reducing power of the extract was determined according to a modified version of the method used by Rahimi-Nasrabadi et al.,[Citation21–Citation23] based on which different concentrations of the extract in methanol (1.0 mL) were mixed with 2.5 mL of a phosphate buffer (200 mM, pH 6.6) and 2.5 mL of a 1% potassium ferricyanide, and the mixture was incubated at 50°C for 20 min. Next, 2.5 mL of trichloroacetic acid (10%) was added to the mixture to stop the reaction, and the mixture was subsequently centrifuged at 3000 rpm for 10 min. There was 2.5 mL taken from the supernatant and was mixed with 2.5 mL of de-ionized water and 0.5 mL of 0.1% ferric chloride, and the absorbance of the as-prepared samples was measured at 670 nm against a blank. The assays were carried out three times and the results were expressed as the mean values ± the standard deviations (SDs). Increased absorbance values indicated a higher reducing power.
Determination of total phenolic contents
The total phenolic content of the extract was determined using the Folin–Ciocalteu reagent according to the method of Singleton and Rossi[Citation24] with some modifications, using gallic acid as the standard. According to this approach, the extract solution containing 1000 μg of the extract was mixed with 46 mL of distilled water in a volumetric flask and 1 mL of the Folin–Ciocalteu reagent were added to it. Next, the flask was vigorously shaken and the mixture was allowed to react for 3 min, and then 3 mL of an aqueous solution of 2% Na2CO3 was added to the above mixture. After incubation for 2 h at room temperature, the absorbance of each mixture was measured at 760 nm. The same procedure was applied to the standard solutions of gallic acid and a standard curve was obtained. The total phenol contents were expressed as µg of gallic acid equivalents per mg of the extract or the oil. All tests were repeated three times and the gallic acid equivalent values were reported as the means ± the SD of the triplicate measurements.
Antibacterial activity
Bacterial strains
The methanol, chloroform, and aqueous extracts were individually tested against a panel of 3 g negative, and 3 g positive microorganisms. The bacteria used in this study were provided from the Iranian Research Organization for Science and Technology (IROST) and included Staphylococcus aureus (PTCC 1431), Bacillus cereus (PTCC 1015), Escherichia coli (PTCC 1338) Klebsiella pneumonia (PTCC 1290), Bacillus subtilis (PTCC 1720), and Salmonella typhimurium (PTCC 1639).
Disc diffusion assay
The agar disc diffusion method was used as the preliminary assay for testing the antibacterial effect of methanol, chloroform and aqueous extracts[Citation25] against six microorganisms of significant importance. A suspension of the tested bacteria (0.1 mL of 106 cells per mL) was spread on the solid media plates. Filter paper discs (6 mm in diameter) were individually impregnated with 10 µL of the 20 mg mL−1 extracts (200 µg/disc) and then placed on the previously inoculated agar plates. The Petri dishes were kept at 4°C for 2 h and then incubated at 37°C for 24 h. Gentamicine (20 µg) and DMSO were used as the positive and negative controls. The diameters of the inhibition zones were measured in millimeters, and the tests were repeated three times.
Determination of minimum inhibitory concentration (MIC)
A broth micro dilution method was used to determine the MIC values.[Citation23] All tests were performed in nutrient broth. The extracts were dissolved in 10% DMSO. A series of extracts each twice more dilute than the previous, was prepared in a 96-well micro titer plate over the range of 7.8–1000 µg mL−1. To this end, the 96-well plates were prepared by dispensing 95 µL of nutrient broth and 5 µL of the inocula into each well. A 100 µL (1000 µg mL−1) aliquot of the stock extract solution was added into the first wells. Then, 100 µL of their serial dilutions was transferred into seven consecutive wells. The last well containing 195 µL of the nutrient broth and 5 µL of the inocula on each strip (without extract) was used as the negative control. The final volume in each well was 200 µL. Gentamicine in the concentration range of 7.8–1000 µg mL−1 was prepared in the nutrient broth and used as the standard drug for the positive controls. The plate was covered with a sterile plate sealer. The contents of each well were mixed on a plate shaker at 200 rpm for 40 s and then incubated at an appropriate temperatures for 24 h. Bacterial growth was determined by measuring the absorbance values at 600 nm and validated by plating 5 µL samples from clear wells on nutrient agar medium. The MIC was defined as the minimum concentration of the extracts at which the bacteria did not show visible growth.
Synthesis of AgNPs
Polar sub-fractions of methanol extract showed high antioxidant activities especially in reducing power assay and thus, were considered as a proper reducer in the synthesis of AgNPs. It was observed that upon the addition of the extract (10.0 mg mL−1, 5 mL) into a reactor containing the aqueous silver nitrate solution (0.01 M), the color of the solution changed to yellowish-brown within 30 min. The reaction was carried out at room temperature under a stirring rate of 750 rpm. This indicates the production of silver nanoparticles.[Citation26] The ultraviolet–visible (UV-Vis) spectra were recorded after 10, 30, 60, and 120 min. Using the UV-Vis spectra, the influence of the reaction time on the formation of Ag NPs was investigated and it was observed that the peak became sharper over time (). The increase in the intensity could be due to increasing the number of nanoparticles produced as a result of reduction of silver ions present in the aqueous solution.[Citation27,Citation28] It has been reported earlier that the absorbance at around 440 nm, is a distinctive character of these metal particles. After the completion of the reaction, the solution was centrifuged at 13,000 rpm for 15 min and the collected particles were washed with distilled water and ethanol to remove the remaining soluble ions on the surface of the particles and dried at 70°C for 5 h.
Figure 1. UV-Vis absorption spectra of the silver nanoparticles prepared via green synthesis method recorded after 10, 30, 60, and 120 min.
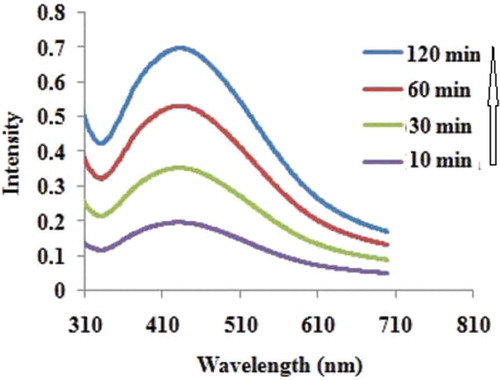
The size dispersion profile of the prepared Ag NPS samples were evaluated through dynamic light scattering (DLS) by a particle size analyzer (PSA: Malvern Zetasizer Nano-ZS). The X-ray diffraction (XRD) studies on the produced NPs were conducted using a powder X-ray diffractometer instrument (Rigaku D/max 2500V) with a Cu target and a graphite monochromator. The product was characterized through transmission electron microscopy (TEM) using a Phillips CM-100 electron microscope. The optical absorption spectra were used to characterize synthesized Ag NPs, with an Epoch UV-Vis spectrophotometer (BioTek Germany, Bad Friedrichshall, Germany).
Results and discussion
Total content of phenolic
The Folin–Ciocalteu method was used for determining the total content of phenolic compounds in T. longipetalus extracts which was expressed in terms of the gallic acid equivalent. Folin–Ciocalteu oxidizes phenolates, producing a molybdenum-tungsten blue complex, which is spectrophotometrically visible at a wavelength of 760 nm. shows the results for the total content of phenol in the PS and non-polar sub-fractions of methanol extract (NS), indicating that the overall phenolic capacity of NS (87.4 ± 2.3 µg/mg) was less than that of the polar one (175.8 ± 5.8 µg/mg as gallic acid equivalents).
Table 1. Antioxidant activities of Tribulus longipetalus extracts.
Antioxidant characteristics
The amount of DPPH radical scavenging
IC50 is commonly used for expressing the DPPH scavenging and is described as the amount of the antioxidant required for scavenging 50% of DPPH in a sample. Thus, the concentrations of the extracts causing a 50% inhibition (IC50) were studied and the results are illustrated in . Lower IC50 is indicative of higher DPPH radical scavenging values, as well as higher reducing powers. With a minimum IC50 value of 70.3 ± 2.1 μg mL−1, the polar sub-fractions obtained from T. longipetalus methanol extracts showed the maximal radical scavenging behavior. Using ascorbic acid (AA) and BHT standards, it was observed that the IC50 of the extracts increased from AA to NS, in order of AA > BHT > PS > NS.
Determining the reducing power
Different studies have indicated the electron donation capacity, reflecting the reducing power, of bioactive compounds in association with their antioxidant activity. The existence of reducing agents (antioxidants) in plant samples, leads to the Fe3+ – Fe2+ reduction reaction, in the reducing assay. Hence, the concentration of the Fe2+ complex might be determined through monitoring the formation of Perl’s Prussian blue (Fe4[Fe(CN)6]3) at 670 nm. The reduction ability of the extract samples with different concentrations are illustrated in . As can be seen in this figure, PS has shown a higher reducing power than the non-polar sub-fraction, which is comparable to the reducing power of AA. Thus PS can be regarded as an effective antioxidant in the reducing power assay and may serve as an effective reducing agent in the preparation of Ag NPs.
Antibacterial activity
The in vitro antimicrobial activities of T. longipetalus extracts were evaluated against the microorganisms previously mentioned, and the activity potentials were assessed based on the presence or absence of inhibition zones, zone diameters and MIC values. Based on the results in , the aqueous extract of T. longipetalus had an appropriate antimicrobial activity against all five bacteria, except for K. pneumoniae. Among bacteria tested, neither the polar, nor the non-polar extracts could inhibit the growth of K. pneumoniae.
Table 2. Antibacterial activity of aqueous and methanol extract of T. longipetalus.
As can be seen in , the highest inhibition zone (12.0 mm ± 1) and the lowest MIC value (256 µg mL−1) were achieved for B. subtilis, which indicated this microorganism as the most sensitive species to polar and non-polar extracts. Other microorganisms sensitive to the extracts of T. longipetalus were E. coli and B. cereus with an MIC value of 500 µg mL−1. Among the two extracts tested, polar and non-polar extracts were found to reveal a maximum inhibition of 9.8 mm at in B. cereus, followed by E.coli (10.3 mm).
Characterization of AgNPs
The synthesized AgNPs were characterized by DLS, in order to determine their size. As shown in , the DLS results revealed that the prepared nanoparticles were about 15 nm in average size. Further evaluations on the produced silver nanoparticles were made through chemical characterizations such as XRD, UV-Vis, and TEM.
The resulting AgNPs were characterized by XRD to evaluate their composition and purity. shows the XRD patterns of the Ag nanoparticles synthesized using T. longipetalus leaf-extract. The characteristic diffraction peaks in the XRD pattern were observed at 2θ = 38.4, 44.5, 64.8, 77.7, and 81.7, and were assigned to the (111), (200), (220), (311), and (222) crystallographic planes of face-centered cubic (fcc) structure for the silver powder sample, respectively (JCPDS file No. 04-0783). No additional diffraction peaks, other than the characteristic peak of the silver structure, were observed indicating the purity of the synthesized silver nanoparticles.
The formation of Ag NPs was also confirmed by UV-visible spectroscopy. A broad absorption peak was obtained at 438 nm, based on the surface Plasmon resonance (SPR) of AgNPs (). No additional peak was obtained in the spectrum which approved the purity of the synthesized products. The microscopic structure of the AgNPs was investigated by TEM. The obtained TEM images are shown in , based on which it is evident that the morphology and general size of the produced silver nanoparticles has been spherical, which was in agreement with the shape of SPR band in the UV-Vis spectra. As can be seen, the approximate average size of the synthesized nanoparticles was estimated to be about 15 nm, which fully complies with the particle size value calculated based DLS analysis.
Conclusion
The extracts of T. longipetalus were used and found to be promising antioxidant and antimicrobial agents in various assays, which can be proposed as a natural additive in food and pharmaceutical industries. Furthermore, the successful and controlled synthesis of AgNPs through a green synthesis route, as a simple, fast, and cost-effective method based on the application of T. longipetalus leaf-extracts was evaluated and the results obtained based on the optimized process showed that silver nanoparticles could be prepared through controlling the effective parameters. The morphology and chemical composition of the silver nanoparticles were characterized by various techniques, i.e., UV-Vis, DLS, XRD, and TEM.
References
- Rahimi-Nasrabadi, M. Electrochemical Synthesis and Characterization of Zinc Sulfide Nanoparticles. Journal of NanoStructures 2014, 4, 211–216.
- Rahimi-Nasarabadi, M.; Ahmadi, F.; Hamdi, S.; Eslami, N.; Didehban, K.; Ganjali, M.R. Preparation of Nanosized Chromium Carbonate and Chromium Oxide Green Pigment Through Direct Carbonation and Precursor Thermal Decomposition. Journal of Molecular Liquids 2016, 216, 814–820.
- Jafari, S.; Faridbod, F.; Norouzi, P.; Shiralizadeh Dezfuli, A.; Ajloo, D.; Mohammadipanah, F.; Ganjali, M.R. Detection of Aeromonashydrophila DNA Oligonucleotide Sequence Using a Biosensor Design Based on Ceria Nanoparticles Decorated Reduced Graphene Oxide and Fast Fourier Transform Square Wave Voltammetry. Analytica Chimica Acta 2015, 895, 80–88.
- Shabani Shayeh, J.; Ehsani, A.; Ganjali, M.R.; Norouzi, P.; Jaleh, B. Conductive Polymer/Reduced Graphene Oxide/Au Nano Particles as Efficient Composite Materials in Electrochemical Supercapacitors. Applied Surface Science 2015, 353, 594–599.
- Alizadeh, T.; Ganjali, M.R.; Akhoundian, M.; Norouzi, P. Voltammetric Determination of Ultratrace Levels of Cerium (III) Using a Carbon Paste Electrode Modified with Nano-Sized Cerium-Imprinted Polymer and Multiwalled Carbon Nanotubes. Micro Chimica Acta 2016, 183, 1123–1130.
- Pourmortazavi, S.M.; Taghdiri, M.; Makari, V.; Rahimi-Nasrabadi, M. Procedure Optimization for Green Synthesis of Silver Nanoparticles by Aqueous Extract of Eucalyptus Oleosa. Spectrochimica Acta Part A: Molecular and Biomolecular Spectroscopy 2015, 136, 1249–1254.
- Rahimi-Nasrabadi, M.; Pourmortazavi, S.M.; Shandiz, S.A.S.; Ahmadi, F.; Batooli, H. Green Synthesis of Silver Nanoparticles Using Eucalyptus Leucoxylon Leaves Extract and Evaluating the Antioxidant Activities of Extract. Natural Product Research 2014, 28, 1964–1969.
- Ghaedi, M.; Yousefinejad, M.; Safarpoor, M.; Zare Khafri, H.; Purkait, M.K. Rosmarinus Officinalis Leaf Extract Mediated Green Synthesis of Silver Nanoparticles and Investigation of Its Antimicrobial Properties. Journal of Industrial and Engineering Chemistry 2015, 31, 167–172.
- Sadeghi, B.; Gholamhoseinpoor, F. A Study on the Stability and Green Synthesis of Silver Nanoparticles Using Ziziphora Tenuior (Zt) Extract At Room Temperature. Spectrochimica Acta Part A: Molecular and Biomolecular Spectroscopy 2015, 134, 310–315.
- Miri, A.; Sarani, M.; Rezazade Bazaz, M.; Darroudi, M. Plant-Mediated Biosynthesis of Silver Nanoparticles Using Prosopis Farcta Extract and Its Antibacterial Properties. Spectrochimica Acta Part A: Molecular and Biomolecular Spectroscopy 2015, 141, 287–291.
- Baghizadeh, A.; Ranjbar, S.; Kumar Gupta, V.; Asif, M.; Pourseyedi, S.; Karimi, M.J.; Mohammadinejad, R. Green Synthesis of Silver Nanoparticles Using Seed Extract of Calendula Officinalis in Liquid Phase. Journal of Molecular Liquids 2015, 207, 159–163.
- Loo, Y.; Woei Chieng, B.; Nishibuchi, M.; Radu, S. Synthesis of Silver Nanoparticles by Using Tea Leaf Extract from Camellia Sinensis. International Journal of Nanomedicine 2012, 7, 4263–4267.
- Amooaghaie, R.; Saeri, M.R.; Azizi, M. Synthesis, Characterization and Biocompatibility of Silver Nanoparticles Synthesized from Nigella Sativa Leaf Extract in Comparison with Chemical Silver Nanoparticles. Ecotoxicology and Environmental Safety 2015, 120, 400–408.
- Ghaffari-Moghaddam, M.; Hadi-Dabanlou, R. Plant Mediated Green Synthesis and Antibacterial Activity of Silver Nanoparticles Using Crataegus Douglasii Fruit Extract. Journal of Industrial and Engineering Chemistry 2014, 20, 739–744.
- Vilchis-Nestor, A.R.; Sánchez-Mendieta, V.; Camacho-López, M.A.; Gómez-Espinosa, R.M.; Camacho-López, M.A.; Arenas-Alatorre, J.A. Solventless Synthesis and Optical Properties of Au and Ag Nanoparticles Using Camellia Sinensis Extract. Material Letters 2008, 62, 3103–3105.
- Santhoshkumar, T.; Rahuman, A.; Bagavan, A.; Marimuthu, S.; Jayaseelan, C.; Virthi, A.; Kamaraj, C.; Rajakumar, G.; Zahir, A.A. Evaluation of Stem Aqueous Extract and Synthesized Silver Nanoparticles Using Cissus Quadrangularis Against Hippobosca Maculata and Rhipicephalus (Boophilus) Microplus. Experimental Parasitology 2012, 132, 156–165.
- Parameshwaran, R.; Kalaiselvamb, S.; Jayavel, R. Green Synthesis of Silver Nanoparticles Using Beta Vulgaris: Role of Process Conditions on Size Distribution and Surface Structure. Materials Chemistry and Physics 2013, 140, 135–147.
- Yousefzadi, M.; Rahimi, Z.; Ghafori, V. The Green Synthesis, Characterization and Antimicrobial Activities of Silver Nanoparticles Synthesized from Green Alga Enteromorpha Flexuosa (Wulfen) J. Agardh. Materials Letters 2014, 137, 1–4.
- Gholivand, M.B.; Rahimi-Nasrabadi, M.;Mehraban, E.; Niasari, M.; Batooli, H. Determination of the Chemical Composition and in Vitro Antioxidant Activities of Essential Oil and Methanol Extracts of Echinophora Platyloba DC. Natural Product Research 2011, 25, 1585–1595.
- Gholivand, M.B.; Rahimi-Nasrabadi, M.; Batooli, H.; Ebrahimabadi, A.H. Chemical Composition and Antioxidant Activities of the Essential Oil and Methanol Extracts of Psammogetoncanescens. Food and Chemical Toxicology 2010, 48, 24–28.
- Rahimi-Nasrabadi, M.; Ahmadi, F.; Batooli, H. Chemical Composition of Essential Oil and in Vitro Antioxidant Activities of the Essential Oil and Methanol Extracts of Eucalyptus Loxophleba. Natural Product Research 2012, 26, 669–674.
- Rahimi-Nasrabadi, M.; Nazarian, S.; Fallah Koohbijari, G.R.; Ahmadi, F.; Batooli, H. Chemical Composition and Antioxidant and Antibacterial Activities of the Essential Oil and Methanol Extracts of Eucalyptus Largiflorens F. Muell. International Journal of Food Properties 2013, 16, 369–381.
- Rahimi-Nasrabadi, M.; Nazarian, S.; Ahmadi, F.; Batooli, H. Chemical Composition and Antioxidant and Antimicrobial Activities of the Essential Oil and Methanol Extracts of Eucalyptus Oleosa. International Journal of Food Properties 2013, 16, 1080–1091.
- Singleton, V.L.; Rossi, J.A. Colorimetry of Total Phenolics with Phosphomolybdic–Phosphotungstic Acid Reagents. American Journal of Enology and Viticulture 1965, 16, 144–158.
- National Committee for Clinical Laboratory Standard. (NCCLS). Performance Standards for Antibacterial Disk Susceptibility Test. Sixth Approved Standard. M2–A6; NCCLS: Wayne, PA, 1997.
- Lukman, A.I.; Gong, B.; Marjo, C.E.; Roessner, U.; Harris, A.T. Facile Synthesis, Stabilization, and Anti-Bacterial Performance of Discrete Ag Nanoparticles Using Medicago Sativa Seed Exudates. Journal of Colloid and Interface Science 2011, 353, 433–444.
- Kumar, V.; Yadav, S.K. Plant‐Mediated Synthesis of Silver and Gold Nanoparticles and Their Applications. Journal of Chemical Technology and Biotechnology 2009, 84, 151–157.
- Nasrollahzadeh, M.; Sajadi, S.M.; Babaei, F.; Maham, M. Euphorbia Helioscopia Linn as a Green Source for Synthesis of Silver Nanoparticles and Their Optical and Catalytic Properties. Journal of Colloid and Interface Science 2015, 450, 374–380.