ABSTRACT
Selected physicochemical properties of native and modified water chestnut starch (Trapabispinosa) were studied. Single- and dual-modifications were carried out by pregelatinization, acetylation and acid-thinning methods. Chemical modification created unevenness on the surface of starch granule. Swelling power, solubility, and water retention capacity were improved by all modification techniques used. The peak viscosity was reduced in all modified starches as compared to native water chestnut starch. The setback value which is an indication of retrogradation (re-association of starch molecules) tendency in the starch paste was reduced after pregelatinization and acid-thinning but increased by acetylation.
Introduction
Starch is the main carbohydrate reservoir for numerous crops and has existed in nature in great quantities. It is located in green leafed plants, e.g., stems, roots, seeds, and fruits. Starch is used by plants as a source of carbon and energy.[Citation1] Each plant has a distinct starch signature and is unique in its morphological characters, chemical composition, and molecular structure.[Citation2] Today, most industries are using native and modified starches to impart proprietary organoleptics to the finished product. Modified food starches are now considered important and useful functional food additives.[Citation3] Comprehensive work has been done in studying the functional, rheological, and physicochemical properties of starches obtained from non-conventional sources. The functionality of starch in various foods is dependent on its physicochemical properties.[Citation4–Citation6] These days, the food processing industry is in search of starch or starches that can withstand extreme conditions of heat and shear associated with processing. Native starches alone cannot be used because of various drawbacks such as low paste clarity, low shear stress resistance, and high retrogradation. A work around to this problem is to tailor the starches via physical and chemical modifications or both to improve native functional properties and thereby, broaden its application.[Citation7] Recent reports mentioned the importance of characterizing starches isolated from unconventional sources as possible raw materials for use in industry. This has had a positive impact on the global processed food market.[Citation8,Citation9]
In Pakistan, the water chestnut locally known as Singhara is an edible aquatic angiosperm which belongs to the Trapaceae family in the genus of Trapabispinosa roxb. This variety is native to Asia, where it is used in cooking. Few South-East Asian countries import this water chestnut from China for food. In both Asia and Europe, the dried nuts are often eaten raw, though more usually they are boiled or roasted. The high carbohydrate content of water chestnuts make them a very good source of starch for both domestic and industrial uses. The food industry has not exploited water chestnut starch for its potential use in processed food. It has been underutilized because its modifications and utilization have not been fully understood. The edible portion of the water chestnut is rich in starch and is composed of thin-walled parenchymatous cells, which store starch inside. The appearance of the cells is similar to those seen in potatoes. Along with the parenchyma cells, there are vascular strands at regular intervals.
The water chestnut starch has a unique property. When heated, especially during cooking or canning, it attains a firm and crunchy texture which distinguishes it from conventional starches like potatoes and rice. Water chestnuts are actually a very good source of starch for the food and allied industry. A detailed study of its characteristics will give a positive thrust to this promising raw material, it will provide valuable data which will facilitate its use in various industries. The starch can be tailored through physicochemical modifications to meet a specific requirement. The utilization of water chestnuts will bring economic benefits to the people. Due to the limitations of native starches, it is necessary to modify these to meet the industry’s requirement.
Uses of native unmodified starches have certain shortcomings that limit their wider application and industrial uses. Functional starches available in the commercial market are usually obtained from corn or other cereals. The physical modification (mainly gelatinization) or simple chemical modifications are sometimes used to fulfill the specific needs of food and technical allied industries. Modified food starches generally show better paste clarity and stability, increased resistance to retrogradation, and freeze–thaw stability.[Citation10] Another most important use of modified starches with respect to the health is as fat replacer, which gives more benefits to product developers and at last consumer can use a reduced fat food.[Citation11]
The aim of this work was to characterize selected properties of the native starch isolated from water chestnuts by means of chemical composition, scanning electron microscopy (SEM), swelling properties, solubility, and pasting properties. The present study will contribute to improved knowledge of physicochemical properties of water chestnut starch and explore its effective utilization in food products.
Material and methods
Isolation of water chestnut starch
Cleaned and dried water chestnuts were used for isolation of starch. All chemicals used were of reagent grade. The starch was isolated by the using the method of Tulyathan et al.[Citation12] The selected dehydrated water chestnuts were comminuted and 1 kg of sample was stirred for 30 min with 2 L of water, while maintaining pH 9.0 by adding 0.2% sodium hydroxide solution. The slurry was filtered through 100 and 170 mesh sieves and centrifuged at 3000 rpm using a Beckman Coulter AllegraTM X-22 centrifuge. The protein layer was scraped off using a spatula. The residue was washed with water to remove color. The starch was then air dried in an oven at 45 ± 1ºC to 13% moisture content.
Starch proximate analysis
The Standard Association of Official Analytical Chemistry (AOAC) methods[Citation13] were adopted for estimating moisture, ash, crude fiber, protein, and fat content.
Purification of water chestnut starch
Purity (on dry basis) expressed as a total starch percentage was calculated as:
The percent yield was calculated using the equation:
reported by Pérez Sira et al.[Citation14]
Physical and chemical modification
Physical modification
The physical modification of starch granules is simple, cheap, and safe. These techniques do not require any chemical or biological agents and are, therefore, preferred when the product is intended for human consumption.[Citation15]
Pregelatinization
Pregelatinization process of starch is classified as a physical modification. This type of modification enhances the solubility in cold water. Pregels are generally used as thickeners in food that receive minimal heat processing.[Citation16] The pregelatinization method as described by Waliszewski et al.[Citation17] was used. A sample of 300 g starch was suspended in 1 L of distilled water and heated to 80°C for 15 min with slow stirring. Pregelatinized starch was placed on stainless steel tray in the form of a thin film (1–2 mm) and dried in a convection oven at 40°C for 48 h, ground in a mortar to pass through a 100 mesh screen, and stored at room temperature in an air-tight glass jar.
Chemical modification
Chemical modification involves the introduction of functional groups on the starch molecule without affecting the morphology or size distribution of the granules. Chemical modifications generate significant changes in starch behavior, gelatinization capacity, retrogradation, and paste properties.[Citation18]
Acetylation
Acetylation of starch can also be termed as stabilization of starch molecules which in turns promotes its use in frozen products. The method of Sathe and Salunkhe[Citation19] was used. One hundred grams of starch was dispersed in 500 mL of distilled water and stirred through magnetic stirrer for 20 min. The pH of the slurry obtained was adjusted to 8.0 using 1 M NaOH. Acetic anhydride (10.2 g) was added over a period of 1 h, while maintaining a pH range 8.0–8.5. The reaction proceeded for 5 min after the addition of acetic anhydride. The pH of the slurry was adjusted to 4.5 using 0.5M HCl. It was filtered and washed four times with distilled water before drying at 30 ± 2°C for 48 h.
Acid-thinning
The method of Lawal[Citation20] was employed with some modifications. One hundred grams of native starch was slurried in 500 mL of 0.05 M HCl. The mixture was stirred magnetically for 8 h, while maintaining a temperature of 50°C. The acid-modified starch was filtered and the residue obtained was washed four times with distilled water. It was air dried for 48 h at 30 ± 2°C. The principle purpose of this type of modification is the production of starch with low viscosity at a higher temperature so that a higher concentration of starch can be added if needed.[Citation21]
Dual modification
Dual modification is a combination of chemical and other methods which results in a modified starch with optimized functionality.[Citation15] Pregelatinized water chestnut starch prepared by using the previously mentioned method was further modified by acetylation and acid-thinning using the procedure of Sathe and Salunkhe[Citation19] and Lawal,[Citation20] respectively, to prepare dual-modified pregelatinized acetylated starch and pregelatinized acid-thinned starch.
Morphological properties
SEMs of native and modified starch granules were taken at 1000 and 600× magnification with a JSM-6380 A microscope (JEOL, Tokoyo, Japan). Starch samples were sprinkled on adhesive tape, attached to specimen studs, and coated with gold JFC-1500 (JEOL, Tokoyo, Japan).
Swelling power and solubility
The swelling power and solubility determinations were carried out in the temperature range of 50–90°C, respectively, using the method of Walizewski et al.[Citation17] Five suspensions of starch (1% w/w) were prepared in a flask. The suspensions were heated to 50, 60, 70, 80, and 90°C, respectively, for 30 min, shaking after every 5 min, and left to cool to room temperature, then centrifuged for 15 min at 3000 × g. The supernatant was decanted, and the residual volume was determined. The solid part was dried in an oven for 2 h at 130°C.
Water retention capacity
Five grams of starch and 100 mL of water were added to tare centrifuged tubes at room temperature and then heated to 50, 60, 70, 80, and 90°C for 15 min, shaking after every 5 min interval. The tubes were centrifuged at 3000 × g for 15 min the supernatant was decanted, and the tubes were allowed to drain for 10 min by keeping an angle of 45°. The tubes were then weighed, and the gain in weight was used to calculate the water retention capacity.[Citation17]
Paste clarity
The paste clarity was determined by Waliszewski et al.[Citation17] with some modifications. Starch suspensions (1%) in tubes with threaded caps were placed in a water bath at 100ºC for 30 min, agitated by vortexing every 5 min, and left to cool to room temperature. Percentage of transmittance (%T) was determined from these suspensions at 650 nm using a spectrophotometer (Beckman DU650, CA, USA).
Freeze–Thaw stability
Freeze–thaw stability, a modified method based on Narkrugsa,[Citation22] was used. Five percent starch solution was heated at a temperature of 95°C with continuous mixing for 20 min. The paste was poured in plastic cups and frozen at –10°C for 1 week. The mixtures were allowed to thaw at 30°C for 4 h and the amount of separated water (FTS, %) from the mixture in the centrifuge tube was reported.
Water absorption
The method of Okezie and Bello,[Citation23] was used for the determination of water absorption of starch. One gram of each sample was taken in 50 mL conical graduated centrifuge tube and thoroughly mixed at high speed with 50 mL distilled water. The samples were allowed to stand at room temperature for 30 min and centrifuged at 5000 × g for 30 min. The volume of free water was measured using a burette. The amount of water absorbed (total minus free) was multiplied by its density for conversion to grams. Absorption capacity was expressed as the grams of water absorbed (or retained) per gram of sample. Determinations were made in triplicate and average values recorded.
Microviscoamylography
Pasting properties of native, acetylated, acid-thinned, pregelatinized, pregelatinized-acetylated, and pregelatinized acid-thinned water chestnut starches were studied using a Brabender Microviscoamylgraph (Model D-47055, Germany). The sample starch was mixed in 100 mL of distilled water, heated from 30 to 95°C with a constant heating rate of 1.5°C/min and then held at 95°C for 30 min. The paste was cooled to 50°C with the same rate. It was finally kept at 50°C for 10 min.
Statistical analysis
Analysis of variance was used to calculate significant differences between the means, and Duncan’s test at p ≤ 0.05 was used to separate means using SPSS software (version 17, SPSS Inc., USA).
Result and discussion
Compositional analysis
Water chestnut starch contained 0.17% of ash, 0.1% of protein, and 0.19% of fat, thus giving 99.54% pure starch (). Moisture content was observed 8.5%, the moisture content plays a significant role in the flow and other mechanical properties, although it depends largely on the method, extent of drying, and the humidity in the surrounding atmosphere.
Table 1. Proximate composition of native water chestnut starch (nWCS).
Morphological properties
Scanning electron micrographs of the water chestnut starches (native and modified) at 1000× magnifications are presented in –. For comparison in size and morphology, water chestnut starch () has round or oval shaped granules with “horn(s)” protruding from its surface. This is in conformity to the earlier studies by Tulyathan et al.[Citation12] In previous studies, oval and round shapes have been reported for potato starches and native beans,[Citation24] while oval and spherical shapes were reported for the field pea.[Citation11] Roughness on the surface of acid-thinned () and acetylated () starches were clearly observed. However, compared to acid-thinned starch (atWCS), acetylation resulted in greater degree of surface erosion, indicating the harshness of acetylated treatment. Dual modification of pregelatinized starch via acetylation further increased the unevenness and roughness on the surface of pregelatinized water chestnut starch granules. The induction of the acetyl groups in acetylated-pregelatinized starch (pgaWCS) alter the inter- and intra-granular interactions within the starch granules that could have resulted in changing the microstructure of starch and more opening of surface pores. Therefore, the results of scanning micrographs reveals an enhanced effect of dual modification on the morphology and microstructure of starch compared to single-step chemical modification.
Figure 1. Scanning electron micrographs of native water chestnut starch (nWCS) under 1000× magnification.
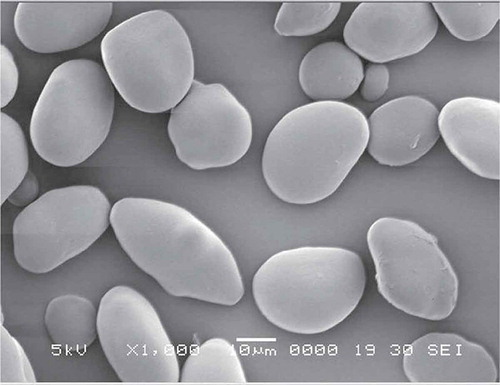
Figure 2. Scanning electron micrographs of pregelatinized water chestnut starch (pgWCS) under 1000× magnification.
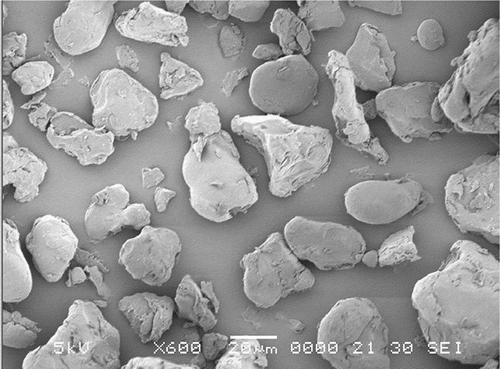
Figure 3. Scanning electron micrographs of acetylated water chestnut starch (aWCS) under 1000× magnification.
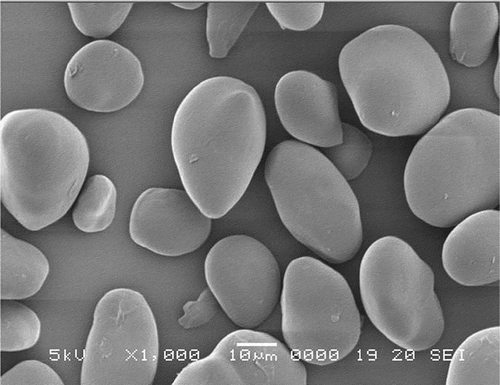
Figure 4. Scanning electron micrographs of acid-thinned water chestnut starch (atWCS) under 1000× magnification.
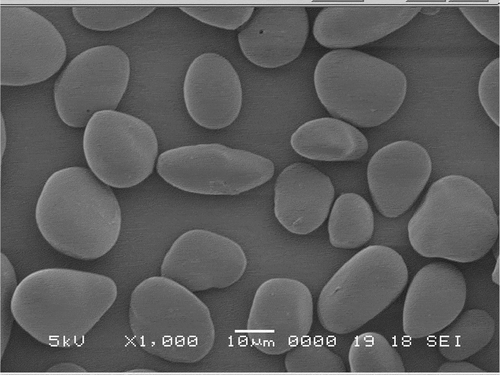
Swelling power and solubility
When starch molecules are heated with excess water the semi-crystalline structure is broken and water molecules associate by hydrogen bonding to hydroxyl groups exposed on the amylose and amylopectin molecules. This association causes swelling and increases the granule size and solubility.[Citation25] Swelling power of native and modified starches was increased linearly with the rise in temperature up to 50–90°C as shown in . According to the results, acid-thinned and pregelatinized starches noticeably restricted the swelling capability compared to nWCS at 70 to 90°C. Similar results have been reported by Lawal.[Citation20] Reduction in swelling power due to acid-thinning was more pronounced than the effect of pregelatinization. In acid modification, acid attacks the surface of starch granule before it penetrates into the inner region. Similarly it attacks on the amorphous region first and then on the crystalline area. Increase in the crystalline area and the presence of dents and physical damage on the surface of granules could be the main reason for restricted swelling. The significant elevation of swelling power was noticed in aWCS than WCS particularly at 80 to 90°C. The induction of acetyl groups on the starch chain played an important role in the improvement of hydrophilic tendency of starch granules which ultimately increased the swelling power.
Table 2. Effect of temperature on (%) swelling power of nWCS, pgWCS, aWCS, atWCS, pgatWCS, and pgaWCS at 50, 60, 70, 80, and 90°C.
Solubility was increased after all types of modifications in water chestnut starch () like swelling power, solubility was also found temperature dependent. The linear increase in solubility with rise in temperature was observed in nWCS, pgWCS, aWCS, atWCS, pgatWCS, and pgaWCS. Higher solubility mainly in acid-thinning due to the disruption of starch chain which ultimately results in less controlled leaching of amylose chains and consequently increased the solubility of starches. Acetylation of water chestnut starch introduced acetyl groups that increase the repulsion forces between the adjacent starch molecules. The lowering of the intramolecular hydrogen bonding forces results in more pronounced increase in solubility especially at 80 and 90°C. The unevenness and roughness created on the surface of starch granules due to single-step pregelatinzation and in dual modification resulted in faster penetration of water into the starch granule and ensuing more solubilization of amylose chains, which resulted in marked increase in solubility.
Table 3. Effect of temperature on (%) solubility of nWCS, pgWCS, aWCS, atWCS, pgatWCS, and pgaWCS at 50, 60, 70, 80, and 90°C.
Water retention capacity
The results of water retention capacity (WRC) of native and modified starches subjected to heating from 50–90°C are shown in . The prominent increase in WRC in modified starches was noticed. Acetylated and acid-thinned starches showed a momentous increase in WRC as compared to nWCS at 80 and 90°C. Similar results were obtained by Wooton and Bamunuarachchi.[Citation26] During acid hydrolysis of starch chains, the more hydroxyl groups were exposed, which results in breakdown of high molecular weight polymer chains to low molecular weight dextrins, thus increased the availability of water binding sites. Acetylation of pregelatinized starch significantly improved WRC from 50 to 70°C. A linear increase in WRC is observed with increase in temperature. The higher mobility of polymer chains due to the disruption of hydrogen bonds with the rise in temperature could be the main cause for the overall availability of water binding sites. The water retention capacity of nWCS is useful to enhance the functional properties of food for example. In instant mixes formulated with instant modified starch. The higher retention power results enlarger granular size of starch which will ultimately reduce the surface area available for hydration and thus results in slower water uptake and prevents lump formation.
Table 4. Effect of temperature on (%) water retention capacity of nWCS, pgWCS, aWCS, atWCS, pgatWCS, and pgaWCS at 50, 60, 70, 80, and 90°C.
Paste clarity
The effect of storage period on the paste clarity of starches is shown in . The result showed that both single and dual modification improved the clarity of water chestnut starch paste. The marked increase was observed in acetylated water chestnut starch compared to native and other modifications, noticeable upturn in percentage of transmittance after acetylation was basically due to the chemical substitution of the hydroxyl groups in starch molecule by the acetyl functional groups. These functional groups produced repulsive forces between the adjacent starch chain which prevented the molecules to re-associate and were responsible for the increase in the percentage of transmittance.
Table 5. Effect on (%) transmittance of nWCS, pgWCS, aWCS, atWCS, pgatWCS, and pgaWCS at 0 h.
Freeze–Thaw stability
The freeze–thaw stability after 7 days of refrigerated storage are presented in . The gel strength was not improved by single and dual modification. When starch gel was kept at freezing temperature, starch-rich regions were created in the matrix and the water present in the matrix remained partially unfrozen. Upon thawing, water may be readily released from the polymeric network (syneresis). The water thus released leaves the starch gel with sponge like texture. In acetylated starches, the presence of acetyl groups on exuded amylose chains possibly interfered with the re-association of starch chains resulting in collapse to form a proper gel network. The reduced strength of acid-thinned starches could be the result of few intermolecular binding forces between the low molecular weight starch chains. This is also evident by low cold paste viscosity () of atWCS. The better freeze–thaw stability of starch will ultimately enhance its aptness for use in food products.[Citation27]
Table 6. Effect on (%) freeze thaw stability of nWCS, pgWCS, aWCS, atWCS, pgatWCS, and pgaWCS.
Table 7. The (%) water absorption of nWCS, pgWCS, aWCS, atWCS, pgatWCS, and pgaWCS.
Table 8. Effect on pasting behavior of nWCS, pgWCS, aWCS, atWCS, pgatWCS, and pgaWCS.
Water absorption
Water absorption of native and modified starches is shown in . Dual modifications, which include pregelatinization followed by acetylation, significantly increased this effect. The observed increase could be due to the electrostatic repulsion of the molecules which provide open spaces for the penetration of water in the matrix.[Citation28] The decrease in water absorption by acetylation and acid-thinning was due to the reduction of available binding sites for water in the starch granules.
Pasting properties
The pasting properties of nWCS, atWCS, aWCS, pgaWCS and pgatWCS are shown in . The gelatinization temperature was decreased after acid-thinning. Gelatinization occurs initially in the amorphous region favored by the weak hydrogen bonds present in this area. The process then extends to the crystalline region. Amylose presence reduces the fusion point in the crystalline region and the amount of energy necessary to initiate gelatinization.[Citation29] Variation in gelatinization temperature is due to the availability of water for amylopectin melting.[Citation31] Generally, greater the water availability lower the gelatinization temperature.[Citation32] This availability of water is due to the hydrolysis of polymer chains and also the surface erosion of starch granules after acid modification may further relieve the process of gelatinization as granules are already collapsed. All modifications lowered the peak viscosity of starch. The peak viscosity of nWCS > pgaWCS > aWCS > atWCS > pgatWCS > pgWCS. Acid-thinning and pregelatinization significantly reduced the peak viscosity. The reduction seems to be due to the partial breakage of glycosidic linkage after modification. This partially degraded network was not resistant to shear and could not maintain the integrity of the starch granules, thereby producing a lower viscosity.[Citation20] The time taken to reach peak viscosity reduced both after single and dual modifications, providing evidence to the fact that aWCS, atWCS, and pgWCS allowed easy penetration of water into the starch granules that led to initial development of peak viscosity in these chemically and physically customized starches. On the other hand, the results revealed that acid-thinning of water chestnut starch further reduced time to reach peak viscosity due to the breakdown of long chain of starch into dextrins, which subsequently result in less restricted water percolation. Breakdown viscosity reflects the measure of fragility of the starch against heat and shear. The negligible increase in breakdown was noticed in pregelatinized starch but it remained unaffected by other modifications. The zero breakdown was showed that modification tightened the water chestnut starch granule which made it more heat resistant, thus requiring more cooking time. The setback value which is an indication of retrogradation (re-association of starch molecules) tendency in the starch paste,[Citation15] was reduced after pregelatinization and acid-thinning but increased by acetylation. During retrogradation the amylose molecules associate with other glucose units to form a double helix while amylopectin molecules re-crystallize through association of its small chains.[Citation25] After retrogradation the starch exhibits lower gelatinization and enthalpy compared to native starch because its crystalline structure has been weakened.[Citation29] After, hydrolysis of long starch chains into short chains have less tendency to re-associate as compared to long starch chains as is obvious by reduction in setback viscosity. The increase in setback viscosity due to addition of acetyl groups in water chestnut starches could be the reason to increased solubility of aWCS () that resulted in higher release of amylose chains. In general retrogradation in starch paste as well as foods containing starch is unfavorable in terms of food quality causing syneresis of gels or hardness. Currently, retrograded starch is classified as a form of resistant starch (RS) and is used in various industries for different purposes.
Conclusion
The results reveal significant changes in morphological, physicochemical, and pasting properties of the water chestnut starch after physical and chemical modifications. The swelling ability of starch is increased and makes it more susceptible to enzymatic hydrolysis. In addition, cold water solubility of starch is enhanced. This affects the applicability in baking and food applications.[Citation32] With high freeze–thaw stability after acid hydrolysis the water chestnut starch can easily be used in the preparation of frozen products and in other industrial applications. The numerous functionalities of water chestnut starch guarantee the desired result and this starch is available to meet the demand. We hope that the data generated from our studies will compliment this search among novel starch sources and will be useful for both food and non-food industrial applications.
References
- Smith, A.M. The Biosynthesis of Starch Granules. Biomacromolecules 2011, 2(2), 335–341.
- Kau, M.; Bhullar, G.K. Partial Characterization of Tamarind (Tamarindusindica L.) Kernel Starch Oxidized at Different Levels of Sodium Hypochlorite. International Journal of Food Properties 2016, 19(3), 605–617.
- Ali, T.M.; Hasnain, A. Morphological, Physicochemical, and Pasting Properties of Modified White Sorghum (SorghumBicolor) Starch. International Journal of Food Properties 2014, 17, 523–535.
- Hoover, R. Composition, Molecular Structure, and Physicochemical Properties of Tuber and Root Starches: A Review. Carbohydrate Polymers 2001, 45, 253–267.
- Moorthy, S.N. Physicochemical and Functional Properties of Tropical Tuber Starches: A Review. Starch/Stärke 2002, 54, 559–592.
- Sanchez-Rivera, M.M.; Garcia-Suarez, F.J.L.; Velazquez del Valle, M.; Gutierrez-Meraz, F.; Bello-Perez, L.A. Partial Characterization of Banana Starches Oxidized by Different Levels of Sodium Hypochlorite. Carbohydrate Polymers 2005, 62, 50–56.
- Fennema, R. Carbohydrates. In Food Chemistry; BeMiller, J.N.; Roy, L.; Eds.; Marcel Dekker: New York, NY, 1996; 201.
- Madineni, M.N.; Faiza, S.; Surekha, R.S.; Ravi, R.; Guha, M. Morphological, Structural, and Functional Properties of Maranta (Marantaarundinaceal.) Starch. Food Science and Biotechnology 2012, 21(3), 747–752.
- Valencia, G.A.; Henao, A.C.A.; Zapata, R.A.V. Comparative Study and Characterization of Starches Isolated from Unconventional Tuber Sources. Journal of Polymer Engineering 2012, 32(8–9), 531–537.
- BeMiller, J.N. Starch Modification: Challenges and Prospects. Starch 1997, 49, 127–131.
- SurendraBabu, A.; Parimalavalli, R.; Jagannadham, K.; Sudhakara Rao, J.; Shalini Gaur, R. Fat Mimicking Properties of Citric Acid Treated Sweet Potato Starch. International Journal of Food Properties 2016, 19(1), 139–153.
- Tulyathan, V.; Boondee, K.; Mahawanich, T. Characteristics of Starch from Water Chestnut (Trapa Bispinsa Roxb.). Journal of Food Biochemistry 2004, 29, 337–348.
- AOAC. Official Methods of Analysis of AOAC International, 17th Ed, Vol 2; AOAC International: Gaithersburg, MD, 2000.
- Pérez Sira, E.E.; Lares Amaiz, M.A. Laboratory Scale Method for Isolation of Starch from Pigmented Sorghum. Journal of Food Engineering 2004, 64, 515–519.
- Ashogbon, A.O.; Akintayo, E.T. Recent Trend in the Physical and Chemical Modification of Starches from Different Botanical Sources: A Review. Starch/Starke 2014, 66(1–2), 41–57.
- Thomas, D.J.; Atwell, W.A. Starch Modifications. In Starches; Eagan Press: St. Paul, MN, 1999; 31–46.
- Waliszewski, K.N.; Aparicio, M.A.; Bello, L.A.; Monroy, J.A. Changes of Banana Starch by Chemical and Physical Modification. Carbohydrate Polymers 2003, 52, 237–242.
- López, O.V.; Zaritzky, N.E.; García, M.A. Physicochemical Characterization of Chemically Modified Corn Starches Related to Rheological Behavior, Retrogradation and Film Forming Capacity. Journal of Food Engineering 2010, 100(1), 160–168.
- Sathe, S.K.; Salunkhe, D.K. Isolation, Partial Characterisation and Modification of the Great Northern Bean (Phaseolus Vulgaris L.) Starch. Journal of Food Science 1981, 46, 617–621.
- Lawal, O.S. Composition, Physicochemical Properties and Retrogradation Characteristics of Native, Oxidized Acetylated and Acid-Thinned New Cocoyam (Xanthosomasagittifolium) Starch. Food Chemistry 2004, 87, 205–218.
- Thomas, D.J.; Atwell, W.A. Grains Based Products. In Starches; Eagan Press: St. Paul, MN, 1999; 53–70.>
- Narkrugsa, W. Changes in Some Physiochemical Properties of Tapioca and Glutinous Rice Starches After Microwave Heating. Kasetsart Journal (Natural Science) 1996, 30, 532–538.
- Okezie, B.O.; Bello, A.B. Physicochemical and Functional Properties of Winged Bean Flour and Isolate Compared with Soy Isolate. Journal of Food Science 1988, 53, 450–454.
- Gujska, E.; Reinhard, W.D.; Khan, K. Physicochemical Properties of Field Pea, Pinto and Navy Bean Starches. Journal of Food Science 1992, 50, 634–636.
- Singh, N.; Singh, J.; Kaur, L.; Sodhi, N.S.; Gill, B.S. Morphological, Thermal and Rheological Properties of Starches from Different Botanical Sources. Food Chemistry 2003 81(2), 219–231.
- Wooton, M.; Bamunuarachchi, A. Water Binding Capacity of Commercial Produced Native and Modified Starches. Starch/Stärke 1978, 30, 306–309.
- Lutfi, Z.; Hasnain, A. Freeze-thaw Stabilization of Water Chestnut (Trapa Bispinosa) Starch in the Presence of Gums and Salts. Trakia Journal of Sciences 2013, 11(2), 163–169.
- Lutfi, Z.; Hasnain, A. Effect of Different Hydrocolloids on Pasting Behavior of Native Water Chestnut (Trapabispinosa) Starch. Agriculturae Conspectus Scientificus 2009, 74(2), 111–114.
- Sasaki, M.; Fang, Z.; Fukushima, Y.; Adschiri, T.; Arai, K. Dissolution and Hydrolysis of Cellulose in Subcritical and Supercritical Water. Industrial & Engineering Chemistry Research 2000, 39(8), 2883–2890.
- Farrero, C.; Martino, M.N.; Zaritzky, N.E. Effect of Hydrocolloids on Starch Thermal Transitions, as Measure by DSC. Journal of Thermal Analysis 1996, 47, 1247–1266.
- White, R.J.; Abbas, I.R.; Johnson, L.A. Freeze-Thaw Stability and Refrigerated-Storage Retrogradation of Starches. Starch/Stärke 1989, 41, 176–180.
- Lutfi, Z.; Hasnain, A. Effect of Added Modified Water Chestnut (Trapabispinosa) Starch on Physical and Sensory Properties of Yeast Leavened Bread. Pakistan Journal of Scientific and Industrial Research. 2011, 54(2), 88–92.