ABSTRACT
A novel phytase from thermophilic Geobacillus sp. TF16 was purified approximately 5-fold using ammonium sulfate precipitation and ion exchange chromatography, and determined as a single band 106.04 kDa on sodium dodecyl sulfate-polyacrylamide gel electrophoresis. Optimum temperature and optimum pH were found to be 85°C and 4.0, respectively. The enzyme is highly thermostable and Vmax and Km values were calculated as 526.28 U/mg and 1.31 mM, respectively. It was also found that the enzyme exhibited a broad substrate selectivity and resistance toward proteases and effectively hydrolyzed soymilk phytate. These results suggest that this study provides an alternative phytase enzyme with enhanced properties.
Introduction
Phytic acid (myo-inositol hexakiphosphate) is the major storage form of phosphorus and inositol in plant seeds such cereal grains, legumes, and oilseed. It is an organic form of phosphorus including 60–90% of the total phosphorus content and is commonly referred to as an anti-nutritional factor since it reduces food intake and nutrient utilization in animals and humans.[Citation1–Citation3] Phytase (myo-inositol hexakisphosphate phosphohydrolase, EC 3.1.3.8) catalyzes the hydrolysis of phytic acid to myo-inositol and phosphoric acid. This process enhances the nutritional quality of phytic acid-rich plant material in feed and food industries by increasing the bioavailability of inositol, phosphoric acid and minerals, for human and monogastric animals.[Citation4] Therefore, phytase has been accepted as an animal feed or a food additive in the worldwide.[Citation5]
Although phytases are found in plants, animals, and microorganisms,[Citation6–Citation9] research has shown that microbial phytases are the most promising candidate to produce phytases used for biotechnological applications on a commercial scale.[Citation1,Citation3] A range of studies has showed that bacterial phytases are more advantageous than their fungal counterparts since they have a high thermal stability, wide pH profile, phytate substrate specificity, and proteolysis resistance[Citation10–Citation12] so that they have been used for industrial applications in food and feed processing.[Citation5] This strongly suggests the importance of purification and characterization of new phytases. In this study, we aimed to determine a new phytase from a thermophilic microorganism, and we report a novel thermal and pH stable phytase enzyme which is resistant to proteolysis purified from Geobacillus sp. TF16. It is, therefore, expected that this novel phytase can be used as an alternative for other phytases. Furthermore, this is one of the first reports studying a phytase from Geobacillus species.
Material and methods
Chemicals
All chemicals used in the study were reagent grade and purchased from Sigma Chemical Co. (St. Louis, MO) and Merck Millipore (Darmstadt, FRG).
Bacterial strain, culture conditions, and preparation of the enzyme extract
Geobacillus sp. TF16 was isolated from Germencik Ömerbeyli Jeotermal in Aydin City, Turkey. Bacterial strain was grown in an enzyme producing medium according to Powar and Jagannathan[Citation13] with slight modifications and incubated at 55°C for 4 days. The medium contained 10% (w/v) white flour to induce the enzyme. Bacterial culture was separated by centrifuge (Hettich, Rotina 35 R model) at 20,000 rpm and 4°C for 20 min and supernatant was used as the crude enzyme extract.
Enzyme purification
Proteins in the crude extract were precipitated using ammonium sulfate. They were fractioned from the supernatant with the addition of ammonium sulfate until different saturation levels reached (10–90%). The obtained precipitates were pelleted by centrifugation at 20,000 rpm for 20 min at 4°C and resuspended in a 50 mM and pH 5.0 sodium acetate buffer. The suspension was desalted in a dialysis bag (MWCO 12,000 Da) using the same buffer and then loaded onto a DEAE-Sepharose Fast Flow ion-exchange column (2 × 30 cm). Proteins were eluted at 3.5 mL/min using a 50 mM and pH 5.0 sodium acetate buffer containing a linear NaCl-gradient with the concentrations between 0 and 1 M. The presence of proteins in collected fractions was monitored by measuring absorbance at 280 nm (ISCO, Retriever 500 model). Fractions were examined for phytase activities using sodium phytate as the substrate. Active fractions were pooled and concentrated via ultrafiltration (Ultracell Membrane 10,000 MWCO Millipore, Amicon, USA). Protein concentration was determined according to the Bradford method[Citation14] with Coomassie Brillant Blue G-250 and bovine serum albumin was used as the standard. The values were obtained by graphic interpolation on a calibration curve at 595 nm. The protein purity was determined by sodium dodecyl sulfate-polyacrylamide gel electrophoresis (SDS-PAGE) analysis. SDS-PAGE was carried out as previously described by Laemmli.[Citation15]
Determination of phytase activity and substrate specificity
Phytase activity was separately determined using a spectrophotometric assay with sodium phytate, 4-methylumbelliferil phosphate, glucose 6-phosphate, adenosine diphosphate (ADP), and adenosine triphosphate (ATP) as the substrates in pH 4.0 and 50 mM Mcilvaine buffer system.[Citation16] The reaction mixture containing 150 µL of the purified enzyme and 450 µL of 5 mM substrate solution was incubated at 85°C for 30 min. The reaction was stopped by adding 600 µL of 15% (w/v) trichloroacetic acid (TCA). Then, 600 µL of the color reactive (6 N H2SO4; 2.5% ammonium molybdate; 10% ascorbic acid; water in the ratio of 1:1:1:2, respectively) was added to the mixture and it was incubated at room temperature for 10 min. Enzyme activity was measured at 625 nm absorbance by spectrometer (Perkin Elmer, Lambda 25 model). A control reaction was carried out with the absence of the enzyme. The standard curve was drawn using 0.5–7.5 μg/mL of KH2PO4 and used for the enzyme quantification assay. A unit (U) of phytase activity was defined as the amount of enzyme required to release 1 μM of inorganic phosphate (Pi) per minute under the assay condition. All the samples were assayed in independent triplicates.
pH Optimum and Stability
The activity of phytase as a function of pH was assayed at 55°C using sodium phytate as the substrate and 50 mM buffer systems; Glycine-HCl (pH 2.2), Mcilvaine (pH 3.0–7.0) or Glycine-NaOH (pH 8.0–9.0). The activity was expressed as a percent relative activity with respect to maximum activity (100%). The effect of pH on the enzyme stability was determined by incubating the enzyme at 4, 37, and 85°C for different incubation times in the buffer solutions of different pH values; Glycine-HCl (pH 2.2) and sodium acetate (pH 4.0, 6.0). After incubation, the phytase activity was assayed under the standard reaction conditions. Control treatments were performed without incubations at different buffer solutions for various times and these were included for each assay under standard conditions of enzyme activity assay. The percentage residual enzyme activity was calculated by comparison with non-incubated enzyme which is considered as the control (100%).[Citation17]
Temperature optimum and thermal stability
Optimum temperature of the enzyme was determined at 50 mM, pH 4.0 Mcilvaine buffer by measuring the enzyme activity at different temperatures from 25 to 105°C with 10°C intervals and sodium phytate was used as the substrate. The activity was expressed as the percent relative activity in relation to the temperature optimum, which was considered as 100%. In order to determine the thermal stability of phytase, the enzyme solutions were separately incubated at 4, 37, and 85°C for different times. Then, phytase activity was assayed under standard reaction conditions. Control treatments were performed without incubations at different temperatures for various times and these were included for each assay under standard conditions of enzyme activity assay. The percentage residual enzyme activity was calculated by comparison with non-incubated enzyme which is considered as the control (100%).[Citation17]
Enzyme kinetics
Kinetic parameters of phytase were obtained by measuring the rate of phytate hydrolysis at various substrate concentrations ranging from 0.08 to 3.3 mM in the standard reaction mixture (at 85°C and 50 mM, pH 4.0 Mcilvaine buffer). The Michaelis–Menten constant (Km) and maximum velocity (Vmax) values were determined from the Lineweaver–Burk plot using Microsoft Excel software.
Effect of some metal ions and chemicals on the enzyme activity
The effect of metal ions on the enzyme activity was separately investigated with the addition of chloride salts of Na+, K+, Li+, Mg2+, Mn2+, Zn2+, Co2+, Ca2+, Ni2+, Cu2+, Hg2+, Fe2+/3+, and Al2+ directly to the standard reaction mixture in a final concentration of 1, 5, and 10 mM. Enzyme activity determined in the absence of metal ion was defined as 100%. To study the effect of some chemicals on the enzyme activity, ethanol, methanol, acetone, 2-propanol, SDS, Triton X-100, Triton X-114, Tween 20, and ethylenediaminetetraacetic acid (EDTA) were separately added to standard reaction mixture in the final concentrations of 1, 5, and 10%. The percentage residual activities were expressed by comparison with standard assay mixture without chemical.[Citation17]
Assay of proteolysis resistance
To measure the phytase resistance against trypsin and chymotrypsin, the purified enzyme (the final concentration was 0.1 mg/mL) was incubated with protease mix (the final concentration was 0.1 mg/mL; tryipsin-chymotryipsin; Sigma-Aldrich, Protease Type VIII; bacterial from Bacillus licheniformis) in 50 mM, pH 8.0 Tris-HCl buffer at the ratio of 1:1 (w/w) for 30, 60, and 90 min at 37°C. The residual phytase activity was assayed using the standard phytase assay. The percentage residual activities were expressed by comparison with standard assay mixture without protease.[Citation18]
Dephytinization of soymilk by the phytase
The potential application of the purified phytase in the nutrition industry was assessed according to Pi release from soymilk. Therefore, soybean seeds were soaked overnight in distilled water and ground in a mixer grinder. The slurry was filtered through double-layered muslin cloth and the particle-free liquid was used as soymilk. Soymilk was incubated with the purified enzyme (10 U/mL) at 85°C and 100 rpm. The samples were drawn at desired intervals, centrifuged, and analyzed with standard activity assay for inorganic phosphate released. To draw a standard calibration curve used for calculating phosphate released, K2HPO4 between 0.5–5.0 mM was used as the substrate and standard assay was performed and percentage of phytate hydrolysis was calculated.[Citation19]
Results and discussion
In this study, a novel phytase from the thermophilic bacterium Geobacillus sp. TF16 was purified and characterized biochemically. Its resistance to proteolysis enzymes and the effect of dephytinization of soymilk were also investigated. The purified phytase shares some enzymatic properties in common with other phytases, but it shows some differences to phytases of other bacterial species suggesting that this study provides an alternative phytase enzyme with enhanced properties.
Production of Geobacillus sp. TF16 phytase
The maximum phytase activity was found at 96 h after Geobacillus sp. TF16 was cultured in phytase-producing medium containing white flour. After centrifugation of culture broth, supernatant was used as the crude extract for phytase purification.
Purification and properties of the enzyme
The phytase obtained from Geobacillus sp. TF16 was purified using ammonium sulfate precipitation and DEAE-Sepharose ion exchange chromatography (). The enzyme was purified approximately 5-fold from the crude extract with 3.13% yield. The purified phytase exhibits a high specific activity of 1080 U/mg protein (). Phytase enzymes were purified 77-, 71.5-, and 25-fold from Bacillus sp. DS11,[Citation20] Aspergillus ficuum NTG-23[Citation18] and Aspergillus niger ATCC 9142[Citation21] with the specific activities 20, 105, and 90 U/mg, respectively.
Table 1. Purification of Geobacillus sp. TF16 phytase.
SDS-PAGE analysis of the purified phytase showed a single band with a subunit molecular mass of 106.04 kDa as shown in . The molecular masses of phytases purified from Klebsiella pneumoniae 9-3B, Bacillus sp. DS11, Lactobacillus sanfranciscensis CB1 and were found 45, 44, and 50 kDa, respectively.[Citation1,Citation20,Citation22] Other Bacillus species and Escherichia coli phytases were shown to have the molecular masses of 36–38 kDa and 42 kDa, respectively.[Citation8,Citation13,Citation23] These suggest that the molecular mass of subunit of Geobacillus sp. TF16 phytase was 2-fold more than bacterial phytases. Our finding is also interesting since the detected molecular mass is closer to its fungal counterparts not to bacterial ones. For instance, the well-known Aspergillus phytases had larger molecular masses; 214 kDa for Aspergillus terrus, 85–100 kDa for A. ficuum, and 200 kDa for A. niger.[Citation24–Citation26]
Figure 1. SDS-Polyacrylamide gel electrophoresis of Geobacillus sp. TF16 phytase. Lane: molecular weight standards (116, 66.2, 45, and 35 kDa), M: non-inducing crude extract, 1: induced crude extract, 2: after 60% ammonium sulfate precipitation, 3: after dialysis, 4: after DEAE-sepharose column chromatography.
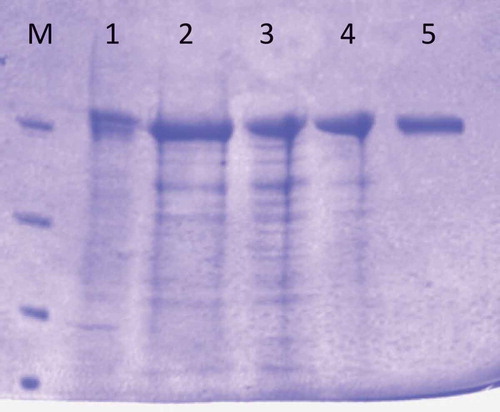
Determination of substrate specificity
Phytase activity was assayed by five substrates; (1) sodium phytate, (2) 4-methylumbelliferil phosphate, (3) glucose 6-phosphate, (4) ADP, or (5) ATP. The highest activity was observed in the presence of 4-methylumbelliferil phosphate, but relative activity was found 48.7% in the presence of sodium phytate (). Similar results were obtained from Bacillus subtilis N-77, A. ficuum NTG-23 and L. sanfranciscensis CB1 phytases.[Citation8,Citation18,Citation22] K. pneumoniae 9-3B, A. niger ATCC 9142, and Candida krusei WZ-001 phytase showed the highest activities for phytate, but they also had an activity with different ratios on other phosphorylated compounds.[Citation1,Citation21,Citation27] On the other hand Bacillus sp. DS11 phytase had high activity for phytate, but no activity on other phosphorylated compounds.[Citation20] These results imply that Geobacillus sp. TF16 phytase is not just specific for inositol polyphosphate but also some inorganic compounds.
Table 2. Substrate specifity of Geobacillus sp. TF16 phytase.
Effect of pH and Temperature on the Enzyme Activity
The maximum phytase activity of Geobacillus sp. TF16 was found at pH 4.0 and approximately 70% of maximum activity was found at pH 3.0 and 5.0 (). These results showed that Geobacillus sp. TF16 phytase was an acidic phytase, whereas phytases from several Bacillus sp. showed the maximum activity around neutral conditions (pH 6.0–8.0).[Citation5,Citation20,Citation28,Citation29] Optimum pH values of some other bacterial phytases purified from K. pneumoniae 9-3B, L. sanfranciscensis CB1, E. coli, Geobacillus stearothermophilus strain DM12, Selenomonas ruminantium, and Pseudomonas sp. were found 4.0, 4.0, 4.5, 4.5, 4.0–4.5, and 5.5, respectively.[Citation1,Citation22,Citation23,Citation30–Citation32] However, fungal phytases from A. niger, Aspergillus oryzae, Emericella nidulans, and Penicillium lycii had maximum activities in the values of 2.2–5.0, 5.5, 6.5, and 5.5, respectively.[Citation33]
Figure 2. A: Effect of pH on Geobacillus sp. TF16 phytase. Assays were performed in 50 mM of different buffer systems at indicated pH; Glycine-HCl (pH 2.2), Mcilvaine (pH 3.0–7.0), and Glycine-NaOH (pH 8.0–9.0). B: Effect of temperature on Geobacillus sp. TF16 phytase. Reactions were performed in 50 mM, pH 4.0 Mcilvaine buffer at different temperatures from 25 to 105°C.
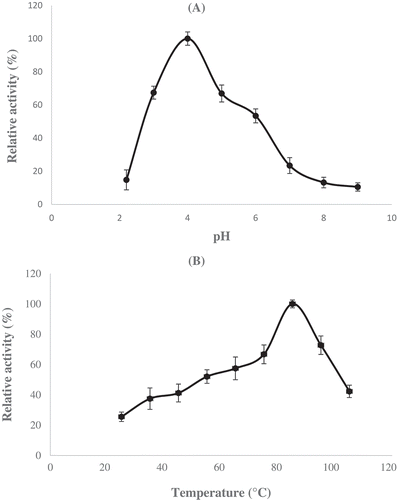
Optimum temperature of Geobacillus sp. TF16 phytase was determined as 85°C at pH 4.0 (). Additionally, it showed 72% relative activity at 95°C. It was higher than the reported optimum temperatures of 60°C for B. subtilis N-77,[Citation8] 70°C for Bacillus sp. DS11,[Citation20] 45°C for L. sanfranciscensis,[Citation22] 50°C for G. stearothermophilus DM12,[Citation30] 70°C for Bacillus amyloliquefaciens,[Citation33] 55°C for E. coli,[Citation33] 40°C for C. krusei,[Citation33] 55°C for A. niger,[Citation33] 70°C for A. terreus,[Citation33] 50°C for A. oryzae,[Citation33] 50°C for P. lycii,[Citation33] and 50–60°C for Enterobacter sp. 4.[Citation34] This finding is therefore crucial as 85°C is the highest optimum temperature reported until now. It is known that higher temperature optimum is important for the phytases as they are widely used to increase nutritional value for pretreatment applications of animal feeds at high temperatures (>80°C). Therefore, we propose that Geobacillus sp. TF16 phytase can be suitable and advantageous for animal husbandary and related fields.
Enzyme kinetics
Kinetic parameters of phytase were determined at various substrate concentrations. Optimum substrate concentration was determined as approximately 2 mM from the Michaelis–Menten graphic (data not shown). Km and Vmax values of Geobacillus sp. TF16 phytase were calculated from the Lineweaver–Burk plot as 1.31 mM and 526.28 U/mg protein, respectively (). Km is represents the enzyme affinity for a specific substrate and this value can alter according to environmental circumstances, e.g., pH, temperature, and ionic strength. While a small Km demonstrated that the enzyme reaches saturation with a small amount of substrate, a large Km demonstrated the requirement of high substrate concentrations to reach maximum reaction velocity.[Citation35] The Km values of phytases are reported to range from 0.08 to 10 mM.[Citation36,Citation37] The Km values of phytases in the presence of sodium phytate substrate were reported to be 0.04, 0.295, 0.55, 0.1, 0.03, 0.177, and 2.19 mM in K. pneumoniae 9-3B, A. ficuum NTG-23, Bacillus sp. DS11, A. niger ATCC 9142, C. krusei, Geobacillus sp. DM12, and B. subtilis 168, respectively.[Citation1,Citation18,Citation20,Citation21,Citation27,Citation30,Citation38] These results showed that 1.31 mM of Km for Geobacillus sp. TF16 phytase is not significantly higher than reported before. Vmax represents the maximum velocity of an enzymatic reactions when the binding site is saturated with substrate.[Citation35] Vmax values were also reported for phytases from A. ficuum NTG-23, A. niger ATCC 9142, G. stearothermophilus DM12, A. niger, Sporotrichum thermophile, and Rhizopus oligosporus ATCC 22959 as 55.9 nmol s−1, 7 nmol s−1, 1.126 µmol/min, 54.35 U/mL, 83 nmol mg−1 s−1 and 1.32 nmol s−1, respectively.[Citation18,Citation21,Citation30,Citation39–Citation41] The findings of current study also present that Vmax value of purified phytase was fairly higher than other phytases determined before.
Thermal stability of phytase
shows the activity profile of the purified enzyme incubated for different incubation periods at various temperatures. The purified phytase was almost completely stable at 4°C after 24 h. The enzyme also conserved its activity above 75% when incubating at 4, 37, and 85°C for 24 h and 50% when incubating at 4, 37, and 85°C for 48 h.
Figure 4. A: Thermal stability of Geobacillus sp. TF16 phytase. Incubations were carried out from 2 to 72 h at temperatures of 4, 37, and 85°C, separately. B, C, D: pH stability of Geobacillus sp. TF16 phytase. Incubations were carried out in the buffers having different pH values Glycine-HCl (pH 2.2) and sodium acetate (pH 4.0, 6.0) from 2 to 96 h at 4°C (B) and from 1 to 4 h at 37 (C) and 85°C (D), separately.
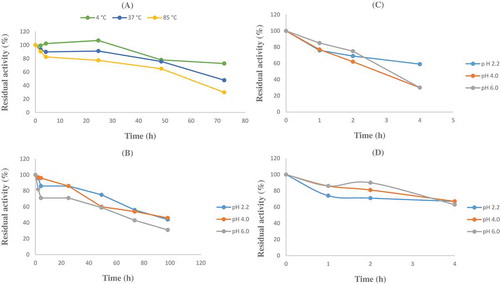
The thermal stability of phytase from Geobacillus sp. TF16 was found higher than observed in phytases of Bacillus nealsonii ZJ0702 (40% at 80°C for 30 min),[Citation5] A. ficuum NTG-23 (85% at 80°C for 60 min),[Citation18] L. sanfranciscensis CB1 (70% at 70°C for 30 min),[Citation22] Penicillium chrysogenum (20% at 80°C for 60 min),[Citation42] and Aspergillus flavus ITCC 6720 (20% at 50°C for 15 min).[Citation43] Additionally, thermal stability studies were performed for A. niger ATCC 9142 and two commercial phytases.[Citation21] Residual activity of A. niger phytase retained 22% after 3 min incubation at 80°C, while comparative values for the commercial products were 18 and 6%. Both commercial enzymes displayed 0% residual activity after 4 min, while A. niger ATCC 9142 enzyme retained 18% activity after 4 min and 12% activity after 5 min. These results illustrated that Geobacillus sp. TF16 phytase has a stronger thermal stability than other phytases containing also its commercial types.
The thermal stability of phytases is essential for animal feed preparation as these enzymes can be normally incorporated into the grains for feed pelleting by treatment for few seconds at 80–85°C.[Citation44] Additionally, thermal stability of the enzyme at 4°C also adds further advantage for easily applicable storage conditions. The results suggest that Geobacillus sp. TF16 phytase is stable both at high and low temperatures, and this feature can make it more attractive for animal feed applications.
pH stability of phytase
pH stability of the purified phytase was determined after enzyme incubation for 2–96 h at 4°C and 1–4 h at 37 and 85°C in the buffers with different pH values, 2.2, 4.0, and 6.0. Following incubation, the activity was assayed under standard reaction conditions. , , and shows that the enzyme retained about 50% of its original activity after 72 h incubation at 4°C and also protected its activity above 60% after 2 h incubation at 37°C and 4 h incubation at 85°C, at pH 2.2, 4.0, and 6.0.
The pH stability profile of B. nealsonii ZJ0702 phytase showed that enzyme lost almost complete of its original activity after 30 min incubation at pH 4.0 and 40% of its original activity after 30 min incubation at pH 6.0.[Citation5] In the other study, it was observed that phytase enzyme from S. thermophile retained 55% of its original activity at pH 5.0 and 30% at pH 3.0 after 960 min incubation period at 60°C.[Citation40] Regarding storage conditions, high stability of the enzyme at 4°C and different pH during extended periods appear to be important. Consequently, it can be said that Geobacillus sp. TF16 phytase is fairly stable in acidic pH at 4 and 85°C.
Effect of some metal ions and chemicals on the enzyme activity
The effect of Na+, K+, Li+, Ca2+, Co2+, Cu2+, Fe2+, Hg2+, Mg2+, Mn2+, Ni2+, Zn2+, Fe2+, and Al2+ on the phytase activity of Geobacillus sp. TF16 was tested at 1, 5, and 10 mM final concentrations of each ion in the reaction mixture. Cu2+ ion at the concentration of 10 mM unexpectedly increased the enzyme activity about 4.5 times, whereas Fe2+, Mg2+, Fe2+, and Al2+ ions at the same concentration slightly increased the activity at different ratios (). The phytase activity of Geobacillus sp. TF16 was also significantly increased by Ca2+ at 1 mM (about 50%), but higher concentration (10 mM) had a slight impact on its inhibitory ability. This concludes that any metal ions used did not remarkably inhibit the phytase activity.
Figure 5. A: Effect of some metal ions on the phytase activity. B: Effect of some chemicals on the phytase activity. C: Residual activity of the purified phytase after treating with tryipsin-chymotryipsin at 1:1 (w/w) of protease/phytase ratio.
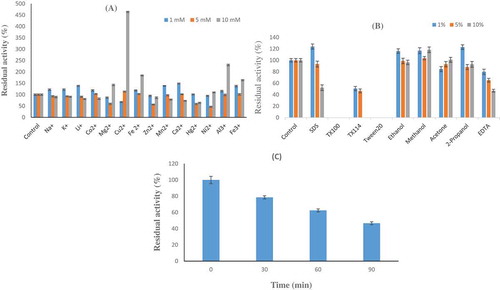
It is known well that the metal ions can affect the enzyme either by increasing or decreasing its activity. Since metal ions can acts as Lewis acids and coordination compounds of these can have different coordination numbers and geometries, they may behave differently toward proteins as ligand. These differences may also result in metal binding to different sites, and, therefore, change the enzyme structure in different ways and affect the enzyme activity.[Citation45] Different bacterial phytases differ in their requirement of metal ions for enzyme activity. Mullaney et al.[Citation46] stated that all Bacillus phytases require Ca2+ ions for both activity and thermal stability. Phytase activity from A. ficuum NRRL 3135 on the other hand is unaffected by calcium ions but inhibited by iron.[Citation47] Another two studies showed that Mg2+, Mn2+, and Cd2+ ions displayed a significant stimulatory effect on A. niger ATCC 9142 phytase and Ca2+, Mn2+, Zn2+, Cu2+, and Co2+ ions and EDTA displayed a partial inhibition on Lactobacillus brevis phytase.[Citation21,Citation48]
Chemicals can alter the microenveriment and 3-D structure of a protein and thus can affect its activity in different ways. Studies on phytase activity also showed that activity can be affected by chemicals in different ways. The enzyme was completely inhibited in the presence of Triton X-100 and Tween 20 and slightly inhibited with EDTA. Additionally, it was shown that low concentrations of SDS stabilized the enzyme (). The presented study provides different findings than previously reported studies for other bacterial and fungal phytases. Fugthong et al.[Citation49] reported that a non-ionic detergent, Tween 20, stabilizes Eupenicillium parvum BCC17694 phytase activity, an anionic detergent, SDS, severely inhibited it. In another study, SDS showed a strong inhibitory effect on other phytases, such as A. niger van Teighem phytase which lost 92% of its activity at 0.1% SDS.[Citation50] In case of S. thermophile phytase, Tween-20, Tween-40, Tween-80, and Triton X-100 stabilized the enzyme activity, while the anionic detergent (SDS) severely inhibited the enzyme even the detergent was at a low concentration.[Citation40] On the other hand, ethanol, methanol, acetone and 2-propanol were not considerably affected the activity (). This observation suggested that hydrophobic residues might not be involved in the catalytic activity of phytase as reported for the phytase of A. niger van Teighem.[Citation50] Ethanol was also shown to inhibit the phytase activity of S. thermophile to some extent, while hexane, butanol, and acetone induced its stabilization.[Citation40]
Proteolysis resistance
Proteolysis resistance is an important characteristic for the enzymes used as feed supplement. Geobacillus sp. TF16 e phytase showed good resistance toward proteolytic enzymes at the ratio of 1:1 (protease/phytase, w/w). Approximately 80 and 60% of phytase activity remained after incubation in the protease solution for 30 and 60 min, respectively (). Trypsin resistance activity of the three commercial phytases from A. niger, A. oryzae, and E. coli was determined as 10, 84, and 79% after 20 min incubation, respectively.[Citation18] However, E. parvum phytase was found to be sensitive to trypsin at ratio of 1:500 (tyripsin:phytase), since relative activity was less than 40%.[Citation49] These results can, therefore, suggest that Geobacillus sp. TF16 phytase is more stable than A. niger, E. coli, and E. parvum phytases.
Dephytinization of soymilk by the phytase
The phytate of soymilk was degraded by the phytase of Geobacillus sp. TF16 at 85°C from 30 min to 4 h and inorganic phosphate liberated was then determined. Enzyme and soymilk incubated at optimal conditions and examples were taken for every 30 min until 4 h is completed. The amount of phosphate liberated was determined using standard calibration curve of K2HPO4 with standard activity assay. Phytic acid concentration of soymilk which was untreated with enzyme was found 28.16 mM. Hydrolysis capacities of Geobacillus sp. TF16 phytase enzyme were 5, 8, 11, and 20% after 30 min, 1, 2, and 4 h incubation, respectively. The phytase cloning from P. chrysogenum was assayed for Pi release from feed cereals. After a 3 h incubation at 37°C, 1.3-, 2.5-, and 1.2-fold increases were obtained in the release of Pi from corn, soybean, and wheat, respectively.[Citation42] Additionally, the avocado phytase hydrolyzed 35% of soymilk phytate in 4 h at 60°C,[Citation19] while the phytase from P. chrysogenum released 2.2-fold Pi from soymilk.[Citation42]
Conclusion
An ideal phytase is desired to have a maximum enzyme activity and stability in high temperatures, a proteolysis resistance, also expected to be able to actively work at acidic pH levels, because it is employed as one of the additives in the diets of monogastric animals.[Citation51,Citation52] This study reports a novel phytase enzyme which was purified and characterized from Geobacillus sp. The results demonstrated that the phytase from Geobacillus sp. TF16 shows optimal activity at acidic pH and broad substrate specificity for compounds containing inorganic phosphate. Purified enzyme has also the greatest optimum temperature, and stronger thermal stability than other phytases reported before. Additionally it showed resistance for some proteases and degradation effect for soymilk phytate. These unique properties can make this phytase an attractive enzyme for the feed and food processing industries when required hydrolysis of phytic acid and phytates.
Acknowledgments
The authors would like to thank Assistant Professor Dr. Kadriye Inan Bektas for providing the bacterium strain.
Funding
This work was supported by TUBITAK (Project number is 214Z190).
Additional information
Funding
References
- Escobin-Mopera, L.; Ohtani, M.; Sekiguchi, S.; Sone, S.; Abe, A.; Tanaka, M.; Meevootisom, V.; Asano, K. Purification and Characterization of Phytase from Klebsiella Pneumoniae 9-3B. Journal of Bioscience and Bioengineering 2012, 113(5), 562–567.
- Yıldırım, A.; Oner, M.D. Electrical Conductivity, Water Absorption, Leaching, and Color Change of Chickpea (Cicer Arietinum L.) During Soaking with Ultrasound Treatment. International Journal of Food Properties 2015, 18(6), 1359–1372.
- Sapna, J.J.; Singh, B.; Characteristics and Biotechnological Applications of Bacterial Phytases. Process Biochemistry 2016, 51, 159–169.
- Pandey, A.; Szakacs, G.; Soccol, C.R.; Rodriguez-Leon, J.A.; Soccol, V.T. Production, Purification and Properties of Microbial Phytases. Bioresource Technology 2001, 77, 203–214.
- Yu, P.; Chen, Y. Purification and Characterization of a Novel Neutral and Heat-Tolerant Phytase from a Newly Isolated Strain Bacillus Nealsonii ZJ0702. BMC Biotechnology 2013, 13(78), 1–7.
- Fu, D.W.; Huang, H.Q.; Luo, H.Y.; Wang, Y.R.; Yang, P.L.; Meng, K.; Bai, Y.G.; Wu, N.F.; Yao, B. A Highly pH-Stable Phytase from Yersinia Kristeensenii: Cloning, Expression, and Characterization. Enzyme and Microbiol Technology 2008, 42(6), 499–505.
- In, M.J.; Jang, E.S.; Kim, Y.J.; Oh, N.S. Purification and Properties of an Extracellular Acid Phytase from Pseudomonas Fragi Y9451. Journal of Microbiology and Biotechnology 2004, 14, 1004–1008.
- Shimizu, M. Purification and Characterization of Phytase from Bacillus Subtilis (Natto) N-77. Bioscience, Biotechnology and Biochemistry 1992, 56(8), 1266–1269.
- Chadha, B.S.; Harmeet, G.; Mandeep, M.; Saini, H.S.; Singh, N. Phytase Production by the Thermophilic Fungus Rhizomucor Pusillus. World Journal of Microbiology and Biotechnology 2004, 20(1), 105–109.
- Oh, B.C.; Choi, W.C.; Park, S.; Kim, Y.O.; Oh, T.K. Biochemical Properties and Substrate Specificities of Alkaline and Histidine Acid Phytases. Applied Microbiology and Biotechology 2004, 63(4), 362–372.
- Kerovuo, J.; Lappalainen, I.; Reinikainen, T. The Metal Dependence of Bacillus Subtilis Phytase. Biochemical Biophysical Research Communications 2000, 268(2), 365–369.
- Kim, D.H.; Oh, B.C.; Choi, W.C.; Lee, J.K.; Oh, T.K. Enzymatic Evaluation of Bacillus Amyloliquefaciens Phytase as a Feed Additive. Biotechnology Letters 1999, 21(11), 925–927.
- Powar, V.K.; Jagannathan, V. Purification and Properties of Phytate-Specific Phosphatase from Bacillus Subtilis. Journal of Bacteriology 1982, 151(3), 1102–1108.
- Bradford, M.M. A Rapid and Sensitive Method for the Quantitation of Microgram Quantities of Protein Utilizing the Principle of Protein-Dye Binding. Analytical Biochemistry 1976, 72, 248–254.
- Laemmli, U.K. Cleavage of Structural Proteins During the Assembly of the Head of Bacteriophage T4. Nature 1970, 227, 680–685.
- Garchow, B.G.; Jog, S.P.; Mehta, B.D.; Monosso, J.M.; Murthy, P.P.N. Alkaline Phytase from Lilium Longiflorum: Purification and Structural Characterization, Protein Expression and Purification. 2006, 46, 221–232.
- Yildirim Akatin, M. Characterization of a β-Glucosidase from an Edible Mushroom, Lycoperdon Pyriforme. International Journal of Food Properties 2013, 16(7), 1565–1577.
- Zhang, G.Q.; Dong, X.F.; Wang, Z.H.; Zhang, Q.; Wang, H.X.; Tong, J.M. Purification, Characterization, and Cloning of a Novel Phytase with Low pH Optimum and Strong Proteolysis Resistance from Aspergillus Ficuum NTG-23. Bioresource Technology 2010, 101, 4125–4131.
- Bicak Celem, E.; Onal, S. Immobilization of Avocado Phytase on Epoxy-Activated Sepabead EC-EP and its Application in Soymilk Phytate Hydrolysis, Artificial Cells, Blood Substitues, and Biotechnology 2009, 37, 195–202.
- Kim, Y.O.; Kim, H.K.; Bae, K.S.; Yu, J.H.; Oh, T.K. Purification and Properties of a Thermostable Phytase from Bacillus sp. DS11. Enzyme and Microbial Technology 1998, 22, 2–7.
- Casey, A.; Walsh, G. Purification and Characterization of Extracellular Phytase from Aspergillus Niger ATCC 9142. Bioresource Technology 2003, 86, 183–188.
- De Angelis, M.; Gallo, G.; Corbo, M.R.; McSweeney, P.L.H.; Facciae, M.; Giovine, M.; Gobbetti, M. Phytase Activity in Sourdough Lactic Acid Bacteria: Purification and Characterization of a Phytase from Lactobacillus Sanfranciscensis CB1. International Journal of Food Microbiology 2003, 87, 259–270.
- Greiner, R.; Konietzny, U.; Jany, K.D. Purification and Characterization of Two Phytase from E.Coli. Archives Biochemistry and Biophysics 1993, 303, 107–113.
- Yamada, K.; Minoda, Y.; Yamada, K. Phytase from Aspergillus Terreus. Part I. Production, Purification and Some General Properties of the Enzyme. Agricultural and Biological Chemistry 1968, 32, 1275–1283.
- Gibson, D. Production of Extracellular Phytase from Aspergillus Ficuum on Starch Media. Biotechnology Letters 1987, 9(5), 305–310.
- Skowronski, T. Some Properties of Partially Purified Phytase from Aspergillus Niger. Acta Microbiologica Polonica 1978, 27, 41–48.
- Quan, C.S.; Fan, S.D.; Zhang, L.H.; Wang, Y.J.; Ohta, Y. Purification and Properties of a Phytase from Candida Krusei WZ-001. Journal of Bioscience and Bioengineering 2002, 94(5), 419–425.
- Kerovuo, J.; Lauraeus, M.; Nurminen, P.; Kalkkinen, N.; Apajalahti, J. Isolation, Characterization, Molecular Gene Cloning and Sequencing of a Novel Phytase from Bacillus Subtilis. Applied and Environmental Microbiology 1998, 64, 2079–2085.
- Gulati, H.K.; Chadha, B.S.; Saini, H.S. Production and Characterization of Thermostable Alkaline Phytase from Bacillus Laevolacticus Isolated from Rhizosphere Soil. Journal of Industrial Microbiology and Biotechnology 2007, 34, 91–98.
- Parhamfar, M.; Badoei-Dalfard, A.; Khaleghi, M.; Hassanshahian, M. Purification and Characterization of an Acidic, Thermophilic Phytase from a Newly Isolated Geobacillus Stearothermophilus Strain DM12. Program in Biological Sciences 2015, 5(1), 61–73.
- Yanke, L.J.; Selinger, L.B.; Cheng, K.J. Phytase Activity of Selenomonas Ruminantium: A Preliminary Characterization. Letters in Applied Microbiology 1999, 29, 20–25.
- Irving, G.C.J.; Cosgrove, D.J. Inositol Phosphate Phosphatases of Microbiological Origin: Some Properties of a Partially Purified Bacterial (Pseudomonas sp.) Phytase. Australian Journal of Biological Sciences 1971, 24, 547–557.
- Asan, M. Microbial Phytases, Applications and Biotechnology. Tarim Bilimleri Dergisi 2007, 13(2) 147–155.
- Yoon, S.J.; Choi, Y.J.; Min, H.K.; Cho, K.K.; Kim, J.W.; Lee, S.C.; Jung, Y.H. Isolation and Identification of Phytase-Producing Bacterium, Enterobacter sp. 4, and Enzymatic Properties of Phytase Enzyme. Enzyme and Microbial Technology 1996, 18, 449–454.
- Nelson, D.L.; Cox, M.M. Enzymes. Principles of Biochemistry; Nelson, D.L.; Cox, M.M.; Eds.; W.H. Freeman and Company: New York, NY, 2008; 194–204.
- Crosgrove, D.J.; Irving, G.C.J. Inositol Phosphates: Their Chemistry, Biochemistry, and Physiology; Cosgrove, D.J.; Ed.; Elsevier Scientific Pub. Co., Amsterdam, 1980; 85–98.
- Nayini, N.R.; Markakis, P. Phytic Acid: Chemistry and Applications; Graf, E.; Ed.; Pilatus Press: Minneapolis, MN, 1986; 101–118.
- Chen, W.; Ye, L.; Guo, F.; Lv, Y.; Yu, H. Enhanced Activity of an Alkaline Phytase from Bacillus Subtilis 168 in Acidic and Neutral Environments by Directed Evolution. Biochemical Engineering Journal 2015, 98, 137–143.
- Ekren, G.S.; Metin, K. Production, Purification and Characterization of Phytase from Phytase Producing Fungus, European Biotechnology Congress, Bratislava, Slovakia, May 16–18, 2013. Current Opinion in Biotechnology. 81S.
- Singh, B.; Satyanarayana, T. Characterization of a HAP–Phytase from a Thermophilic Mould Sporotrichum Thermophile. Bioresource Technology 2009, 100, 2046–2051.
- Casey, A.; Walsh, G. Identification and Characterization of a Phytase of Potential Commercial Interest. Journal of Biotechnology 2004, 110, 313–322.
- Correa, T.L.R.; de Queiroz, M.V.; de Araujo, E.F. Cloning, Recombinant Expression and Characterization of a New Phytase from Penicillium Chrysogenum. Microbiological Research 2015, 170, 205–212.
- Gaind, S.; Singh, S. Production, Purification and Characterization of Neutral Phytase from Thermotolerant Aspergillus Favus ITCC 6720. International Biodeterioration and Biodegradation 2015, 99, 15–22.
- Rao, D.E.; Rao, K.V.; Reddy, T.P.; Reddy, V.D. Molecular Characterization, Physicochemical Properties, Known and Potential Applications of Phytases: An Overview. Critical Reviews in Biotechnology 2009, 29(2), 182–198.
- Ditusa, C.A.; Christensen, T.; McCall, K.A.; Fierke, C.A.; Toone, E.J. Thermodynamics of Metal Ion Binding. 1. Metal Ion Binding by Wild-Type Carbonic Anhydrase. Biochemistry 2001, 40, 5338–5344.
- Mullaney, E.J.; Daly, C.B.; Ullah, A.H.J. Advances in Phytase Research. Advances in Applied Microbiology 2000, 47, 157–199.
- Ullah, A. Aspergillus Ficuum Phytase, Partial Primary Structure, Substrate Selectivity and Kinetic Characterization. Preparative Biochemistry 1988, 18(4), 459–471.
- Sumengen, M.; Dincer, S.; Kaya, A. Phytase Production from Lactobacillus Brevis. Turkish Journal of Biology 2012, 36, 533–541.
- Fugthong, A.; Boonyapakron, K.; Sornlek, W.; Tanapongpipat, S.; Eurwilaichitr, L.; Pootanakit, K. Biochemical Characterization and in Vitro Digestibility Assay of Eupenicillium parvum (BCC17694) Phytase Expressed in Pichia Pastoris. Protein Expression and Purification 2010, 70, 60–67.
- Vats, P.; Banerjee, U. Biochemical Characterisation of Extracellular Phytase (Myo-Inositol Hexakisphosphate Phosphohydrolase) from a Hyper-Producing Strain of Aspergillus Niger van Tieghem. Journal of Industrial Microbiology and Biotechnology 2005, 32, 141–147.
- Singh, B.; Kunze, G.; Satyanarayana, T. Developments in Biochemical Aspects and Biotechnological Applications of Microbial Phytases. Biotechnology and Molecular Biology Reviews 2011, 6(3), 69–87.
- Singh, B.; Satyanarayana, T. Phytases from Thermophilic Molds: Their Production, Caracteristics and Multifarious Applications. Process Biochemistry 2011, 46(7), 1391–1398.