ABSTRACT
Diospyros lotus fruit polyphenol oxidase was purified using affinity chromatography, resulting in a 15-fold enrichment in specific activity. The purified enzyme, having 16.5 kDa molecular weight on sodium dodecyl sulfate-polyacrylamide gel electrophoresis, exhibited the highest activity toward 4-methylcatechol. Maximum diphenolase activity was reached at pH 7.0 and 60°C in the presence of 4-methylcatechol. Km and Vmax values were calculated as 3.8 mM and 1250 U/mg protein, respectively. Ascorbic acid was a promising inhibitor with an IC50 value of 0.121 µM. The activity of the purified enzyme was stimulated by Fe2+, Sr2+, Zn2+, and K+ and deeply inhibited by Hg2+, at 1 mM final concentration. Aqueous extract of Diospyros lotus L. fruit showed strong substantial urease and acetylcholinesterase inhibition, with IC50 values of 1.55 ± 0.05 and 16.75 ± 0.11 mg/mL, respectively.
Introduction
Polyphenol oxidase (PPO) is an enzyme causing a browning reaction during the handling, storage, and processing of fruits and vegetables. The enzyme exerts its action via hydroxylation of monophenols to their corresponding o-diphenols (monophenolase activity), and oxidation of o-diphenols to their congeneric o-quinones (diphenolase or catechol oxidase activity) using phenolic compounds and molecular oxygen as substrates.[Citation1] The resulting o-quinones easily polymerize or react with a variety of cellular constituents, such as amino acids and proteins, to form complex brown, red, or black pigments.
Diospyros lotus L. (D. lotus; plum persimmon) is a deciduous tree, native to China and Asia,[Citation2] growing naturally in northeast and southern Anatolia, where it has been cultivated for its edible fruits.[Citation3] D. lotus fruit is a potential source of natural antioxidants owing to its significant antioxidant[Citation2] activity and has strong antiproliferative effects against C32 and A375 cells.[Citation4] A number of PPOs from Diospyros kaki have been characterized;[Citation5–Citation7] however, there are not any reports existing on D. lotus PPO.
Free radicals, such as superoxide anion (O2−), hydroxyl radical (OH•), and hydrogen peroxide (H2O2), are mainly generated by metabolic processes in the human body, and some environmental factors have essential roles in cell signaling, apoptosis, and gene expression.[Citation8] However, when the level of reactive oxygen species (ROS) exceeds the capacity for defense or antioxidant systems, the result is the induction of ageing, abnormal physiological functions, or various human diseases such as inflammation and neurodegenerative conditions including Alzheimer’s disease (AD). The main strategy in the clinical treatment of AD involves the maintenance of adequate levels of acetylcholine (ACh) at neurotransmission sites. Thus, the inhibition of acetylcholinesterase (AChE) prevents the hydrolysis of ACh, thereby maintaining normal memory function. The consumption of antioxidants is highly correlated with lower incidences of AD.[Citation9,Citation10] The urease activity of Helicobacter pylori plays an important role in the pathogenesis of gastric and peptic ulcers. Acetohydroxamic acid has been clinically used for the treatment of urinary tract infections by urease inhibition.[Citation11] However, it exhibits severe side effects such as difficulty in breathing and swelling of the face, lips, tongue, or throat. Therefore, there is a need to explore more effective antiulcer agents and urease inhibitors possessing enhanced efficacy against microorganisms while exhibiting less toxicity to human cells.
According to prior studies, nutritional constituents, phenolic content, antioxidant activities, and radical scavenging properties of the D. lotus fruit have been studied.[Citation2,Citation3] To the best of our knowledge, neither PPO enzyme, nor urease and AChE inhibitory properties have been studied in D. lotus.
In addition to the literature, this work covers investigation of urease and AChE enzyme inhibitory properties, characterization of PPOs, effects of pH and temperature on PPO activity and stability, effects of inhibitors on PPO activity, and substrate specificity of PPO from D. lotus.
Materials and methods
D. lotus was procured directly from a local garden from Iyidere town in Rize province, Turkey. The fruits were processed in the laboratory immediately after picking in December. All the reagents used in this study were analytical grade (Sigma-Aldrich Inc., St. Louis, MO, USA) and were used without further purification.
Polyphenoloxidase extraction and purification
Frozen D. lotus fruit without cores (100 g) was ground in 200 mL of cold extraction solution composed of acetate buffer (pH 5.0, 50 mM) with 2 mM ethylenediaminetetraacetic acid (EDTA), 1 mM MgCl2, 1 mM phenylmethylsulphonyl fluoride and 6% (w/v) Triton X-114.[Citation12] The solution obtained was centrifuged at 10,000 × g for 30 min at 4°C. The supernatant was kept at 4°C for 24 h and homogenized using a blender (1 min). The homogenate was filtered through filter paper, and the filtrate was used as the enzyme extract. To precipitate proteins, four volumes of cold acetone were added to the supernatant, and the mixture was incubated overnight at −20°C then centrifuged at 10,000 rpm for 30 min at 4°C. The precipitate was redissolved in an appropriate volume of 50 mM acetate buffer (pH 5.0) and was recentrifuged at 5000 rpm for 10 min at 4°C to remove undissolved components. This supernatant was then used as the enzyme solution.
The enzyme solution was applied to a 1 × 15 cm Sepharose 4B-L-tyrosine-p-amino benzoic acid affinity column,[Citation13] pre-equilibrated with 50 mM acetate buffer (pH 5.0). The affinity gel was washed with the same buffer, and PPO was eluted with a solution of 50 mM phosphate buffer containing 1 M NaCl (pH 8.0).
Determination of protein concentration
Protein quantity was determined using the Bradford method,[Citation14] with bovine serum albumin as a standard.
Enzymatic assay and characterization of PPO
Enzyme activity
Enzyme activity was assayed by measuring the rate of increase in absorbance at 494 nm for 4-methylcatechol, and at 500 nm for all other substrates, at optimum conditions, as described previously.[Citation15] Unless otherwise stated, the standard reaction mixture consisted of substrate (stock 100 mM), an equal volume of 3-methyl-2-benzothiazolinone hydrazone hydrochloride hydrate (stock 10 mM) and 20 µL dimethylformamide, which was diluted to 950 µL with buffer, and then 50 µL crude enzyme extract was added. The reference cuvette comprised all the reactants except the crude enzyme. One unit of PPO activity was defined as the amount of enzyme causing 0.001 increase of absorbance per minute in 1 mL of reaction mixture.[Citation5]
pH optimum
PPO activity, as a function of pH, was ascertained by conducting a standard activity assay at different pHs. The following buffers were used: 50 mM glycine-HCl buffer pH 3.0, 50 mM acetate pH 4.0 and 5.0, 50 mM phosphate buffer between pH 6.0 and 7.0, and Tris-HCl buffer between pH 8.0 and 9.0. 4-Methylcatechol was used as a substrate.
Thermal activity
The optimal reaction temperature was assessed by performing enzyme activity tests at temperatures ranging from 10 to 80°C, in 10°C increments, using 4-methylcatechol as the substrate.[Citation16]
Substrate specificity and enzyme kinetics
The substrate specificity of PPO was determined by measuring its activity against catechol, 4-methylcatechol, 3-(3,4-dihydroxyphenyl) propionic acid (DHPPA), L-3,4-dihydroxyphenylalanine (L-DOPA), and p-hydroxyphenyl propionic acid (PHPPA).
The Michaelis–Menten constants (Km) and the maximum reaction velocity (Vmax) were determined by using 4-methylcatechol. The standard activity assay was carried out for different concentrations of the substrate at optimum pH and temperature. Data from the linear range of the Michaelis–Menten plot was used to construct a double-reciprocal plot according to the method described by Lineweaver and Burk.[Citation17] The value of Vmax was deduced from the reciprocal of the intercept of the straight line on the ordinate, and the values of Km from the negative reciprocal of the intercept on the x-axis, using the following equation: 1/V = Km/Vmax + 1/([S] + 1/Vmax), in which V is the initial reaction rate, [S] is the initial substrate concentration, Vmax is the maximum reaction rate acquired at the absolute initial substrate concentration, and Km is the Michaelis–Menten constant.
Effect of metal ions on PPO activity
PPO activity in the presence of Na+, K+, Hg2+, Fe2+, Ba2+, Mg2+, Ca2+, Mn2+, Cd2+, Co2+, Ni2+, Zn2+, Al3+, and Sr2+ at 1.0 and 5.0 mM final concentrations were measured under the optimum reaction conditions, using 4-methylcatechol as the substrate. The percentage residual activity was determined by comparing with a standard assay mixture with no metal ions added.[Citation5]
Effect of inhibitors on PPO activity
The effects of inhibitors, such as sodium metabisulphite, ascorbic acid, sodium azide, and benzoic acid, on D. lotus PPO activity were studied in the presence of 4-methylcatechol as substrate, at different concentrations to determine a range of inhibitors needed to estimate the concentration of inhibitor that gave 50% inhibition of maximal absorbance (IC50 value). Assays were then repeated with/without an inhibitor, at a determined range of inhibitor concentrations.[Citation18]
Polyacrylamide gel electrophoresis
For sodium dodecyl sulfate-polyacrylamide gel electrophoresis (SDS-PAGE) and native PAGE, protein samples were subjected to a Mini-PROTEAN Tetra Cell electrophoresis unit (Bio-Rad Laboratories, Inc.) in 10% polyacrylamide gel.[Citation19] After electrophoresis, the native PAGE gel was stained with 24 mM L-DOPA for an hour. Coomassie Brilliant Blue R-250 was used for staining SDS-PAGE, and the molecular weight of the apparent polypeptide was determined by applying a calibration curve of the protein standards.[Citation12]
Urease and AChE Inhibition of Diospyros lotus L. extract
Preparation of aqueous extract of Diospyros lotus L. for enzyme inhibition studies
Approximately 20 g of D. lotus was blended and extracted with 100 mL bidistilled water and shaken in an ultrasonic bath (Heidolph Promax 2020, Schwabach, Germany) for 3 h at room temperature. The crude mixture was then centrifuged at 10,000 rpm for 30 min at 4°C, to remove solid particles, and the filtrates were lyophilized (Christ-Alpha 1-4 LD plus, Osterode am Harz, Germany). The lyophilized filtrate was dissolved in 20 mL distilled water. The aqueous extract of D. lotus was used for enzyme inhibition studies.
Urease and AChE inhibition properties of Diospyros lotus L
Urease is an enzyme that catalyses the hydrolysis of urea into carbon dioxide and ammonia. The production of ammonia was measured using the indophenol method, used to determine urease inhibitory activity.[Citation20] Reaction mixtures including 200 μL of jack bean urease, 500 μL of buffer (100 mM urea, 0.01 M K2HPO4, 1 mM EDTA, and 0.01 M LiCl, pH 8.2) and 100 μL of the extract were incubated at room temperature for 20 min. Phenol reagent (550 μL, 1% w/v phenol and 0.005% w/v sodium nitroprusside) and alkali reagent (650 μL, 0.5% w/v sodium hydroxide and 0.1% v/v NaOCl) were added to each tube and the increased absorbance at 625 nm was measured after 50 min, using a ultraviolet-visible (UV/Vis) spectrophotometer (1601 UV-Shimadzu, Australia). Thiourea was used as the standard inhibitor. The percentage inhibition was calculated from the formula % Inhibition = [(ODcontrol − ODtest)/(ODcontrol) × 100]. In order to calculate IC50 values, different concentrations of extracts and standards were assayed in the same reaction conditions.
The aqueous extracts of D. lotus were subjected to Ellman’s test[Citation21] in order to evaluate their potency to inhibit AChE from electric eels (Sigma-Aldrich, St. Louis, MO, USA). This method is based on the reaction of released thiocholine to give color to a product with a chromogenic reagent, 5,5-dithio-bis(2-nitrobenzoic) acid (DTNB). The enzyme solution was prepared in gelatin solution (1%), at a concentration of 2.5 units/mL. AChE (50 µL) and extract (50 µL) were added to 3.0 mL phosphate buffer (pH 8.0, 0.1 M) and incubated at 25°C for 5 min. The reaction was started by adding DTNB (100 µL) and acetyl thiocholine chloride (ATC, 20 µL) to the enzyme–inhibitor mixture. The production of the yellow anion was recorded after 10 min at 412 nm, using a UV/Vis spectrophotometer (1601UV-Shimadzu, Australia). As a control, an identical solution of the enzyme without the inhibitor was processed with the same protocol. AChE inhibition activity was expressed as the percentage of remaining AChE activity, calculated as [(B/A) × 100], where A is the change in absorbance of the assay without the test samples (Δ abs with enzyme − Δ abs without enzyme), and B is the change in absorbance of the assay with the test sample (Δ abs with enzyme − Δ abs without enzyme). Donepezil hydrochloride was used as a positive control. All processes were assayed in triplicate.
Results and discussion
Enzyme purification
PPO was purified from D. lotus by a Sepharose 4B-L-tyrosine-p-amino benzoic acid affinity column,[Citation13] from which it could be observed that the enzymatic activity was eluted in one peak, coinciding with the protein peak (). The protein content was determined spectrophotometrically at 280 nm, and PPO activity was assayed for all fractions. The fraction with the highest protein content and PPO activity was used in further studies. There was an approximately 15-fold increase in activity after the purification (). However, the affinity chromatography technique used in this study has also been successfully used in PPO purified from Morus alba,[Citation13] Boletus erythropus,[Citation5] Lactarius piperatus,[Citation22] Ocimum basilicum,[Citation23] and Macrolepiota gracilenta[Citation12] with 11.5- to 74-fold increases in purification.
Table 1. Purification profile of PPO from D. lotus.
Native and SDS polyacrylamide gel electrophoresis
Following the affinity chromatography step, purity and molecular weight were examined using denaturating and non-denaturating PAGE. The purified PPO was homogenous, as it produced a single protein band on SDS-PAGE corresponding to a molecular mass of approximately 16.5 kDa (). The molecular masses of PPOs from Diospyros kaki, after SDS denaturation, displayed two main protein bands corresponding to approximately 22–28 kDa.[Citation6] In another study, chestnut kernel PPOs also displayed two bands, corresponding to approximately 30–35 kDa.[Citation24] Beside these examples, a staining profile of mulberry PPO displaying a protein band corresponding to a molecular weight as high as 66 kDa has also been reported.[Citation13]
Figure 2. (a) SDS-PAGE of purified D. lotus PPO. Gel stained with Coomassie Brilliant Blue R-250. M: molecular weight marker; 1: crude extract; 2: supernatant obtained after the acetone precipitation step; 3: PPO purified by affinity chromatography. (b) Native electrophoresis of purified D. lotus PPO. Gel revealed with L-DOPA. 1: crude extract; 2: supernatant obtained after the acetone precipitation step; 3: PPO purified by affinity chromatography.
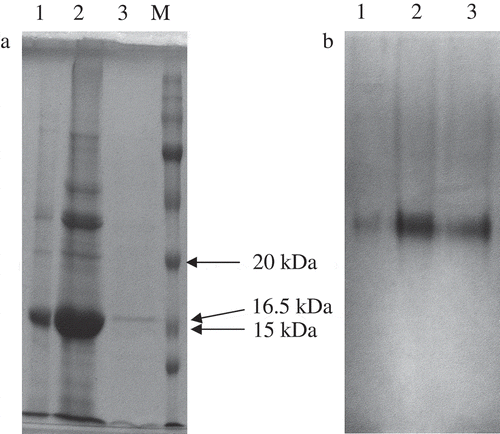
Non-denaturing PAGE was carried out at 4°C, by using a 10% separating gel, and then stained with L-DOPA that demonstrated only one band as having the same Rf value for crude enzyme extract and pure enzyme eluate (). Both D. lotus crude extract and the purified eluate displayed only one band having an active PPO.
Substrate specificity and enzyme kinetics
The oxidative activity of the purified PPO was processed with well-known substrates, namely catechol, 4-methylcatechol, DHPPA, L-DOPA, PHPPA, and L-tyrosine. 4-Methylcatechol showed the maximum activity, and this was taken as 100%, in order to compare its activity with other substrates ().
Table 2. Substrate specificity of D. lotus polyphenol oxidase.
4-Methylcatechol was efficiently oxidized by the purified PPO; as a result, higher enzyme activity was observed with substrates having a catechol ring structure or with a smaller organic moiety. With an increase in molecular mass of this group, the activity decreased, as observed in the case of DHPPA. The enzyme did not display any activity against monophenols such as tyrosine. 4-Methylcatechol and catechol are reported to be substrates that are usually chosen for determining the activity of PPO isolated from plants.[Citation5,Citation12,Citation22,Citation25–Citation27] The effect of 4-methylcatechol concentration on PPO activity was further investigated. The Michaelis–Menten constant (Km) and the maximum velocity (Vmax) values for 4-methylcatechol were calculated from a plot of 1/V against 1/[S].[Citation17] The Km value can change depending upon the structure of the enzyme, pH, temperature and ion strength.[Citation28] The apparent Km and Vmax values for D. lotus PPO were calculated as 3.8 mM and 1250 U/mg protein at optimum reaction conditions, respectively. The Km value, using 4-methylcatechol as a substrate, was computed as 3.57 mM for Pyrus elaeagnifolia subsp. elaeagnifolia Pallas wild pear,[Citation29] 3.88 mM for Cape gooseberry,[Citation30] 14.6 mM for Turkish persimmon,[Citation1] and 94 mM for chestnut kernel.[Citation24] It is clear that previous reports reveal the variability of apparent Km values of PPOs from different sources.
pH optimum and thermal activity
Among the pH-dependent properties of macromolecules, two are of particular interest, activity and stability.[Citation31] The relative activity of D. lotus PPO at a pH range of 3.0–9.0 is shown in . D. lotus PPO has an optimum pH value of 7.0 with 4-methylcatechol as substrate. It is apparent that PPO activity is exceedingly affected by pH at room temperature. It was reported that Rojo Brillante persimmon PPO showed an optimum pH at 7.5.[Citation6] The pH value can change the charge state of the substrate molecule and enzyme molecule, which affects the combination of enzyme and substrate. A higher or lower pH could affect enzyme stability, irreversibly destroying it.[Citation24] The pH optimum for PPO is reported to be observed in the range 4.0–9.0 depending on extraction method, substrate, location of enzyme in the cell and enzyme source.[Citation32] The maximum activity was found around pH 9.0 for Amasya apple,[Citation33] 7.5 for Cimin grape,[Citation34] 7.0 for Pyrus elaeagnifolia subsp. elaeagnifolia Pallas wild pear,[Citation29] and 6.0 for aubergine[Citation35] for PPOs in the presence of 4-methylcatechol as substrate.
Figure 3. (a). pH activity profile for PPO from D. lotus. The effect of pH on PPO activity was measured for pH 3.0–9.0. The buffers used were: glycine-HCl (50 mM, pH 3.0), acetate (50 mM, pH 4.0 and 5.0), phosphate (50 mM, pH 6.0 and 7.0) and Tris-HCl (50 mM, pH 8.0 and 9.0). (b) Effect of temperature on PPO activity from D. lotus. The dependence of PPO activity on temperature was determined under standard assay conditions between 10 and 80°C. The relative activity percentage was calculated based on the maximum activity taken as 100%; 4-methylcatechol was used as substrate.
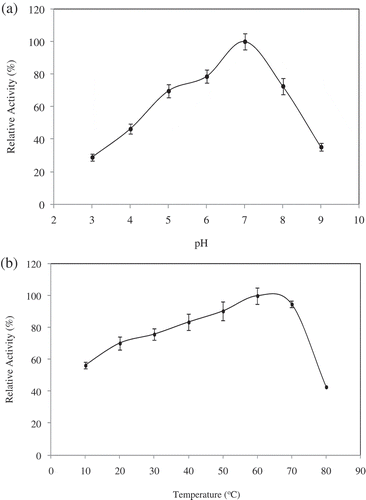
The thermal activity of PPO from D. lotus PPO is presented in . Maximum activity was attained at about 60°C. Optimum temperatures reported in the literature for other Diospyros varieties were different from each other. D. lotus PPO optimum temperature was similar to that reported for the PPO from a Spanish Diospyros variety,[Citation6] but different from the PPO obtained in a Turkish Diospyros variety.[Citation1] Authorities mentioned that optimum temperatures for PPOs from other natural sources are generally lower than 60°C. PPO from chestnut kernel,[Citation24] Pouteria sapota,[Citation36] Phaseolus vulgaris,[Citation27] rape flower,[Citation37] Macrolepiota gracilenta,[Citation12] and Lactuca sativa var. capitata[Citation38] showed optimum temperatures at 40, 25, 37, 40, 40, 30–40, and 35°C, respectively.
Effect of metal ions and possible inhibitors of PPO activity
Previous studies revealed that various metal ions either inhibited or stimulated PPO activity.[Citation5,Citation22,Citation25] Thus, the effects of fourteen metal ions at 1.0 and 5.0 mM final concentrations on PPO activity were compared (). At 1.0 mM final concentration, Fe2+, Sr2+, Zn2+, and K2+ stimulated activity by 19, 22, 15, and 17%, respectively. However, Hg2+ deeply inhibited activity (99%). In the case of Ni2+, Mn2+, Ba2+, Cd2+, Mg2+, and Na+, activity was decreased by 18, 13, 11, 12, and 35%, respectively, but Ca2+, Cd2+, Co2+, and Al3+ did not cause significant inhibition. At 5.0 mM final concentration, it was observed that all metal ions inhibited the enzyme at a different ratio. Metal ions may have different coordination numbers and geometries in their coordination compounds and potentials, such as Lewis acids. So, they may behave differently toward proteins such as ligands. These differences may also result in metal binding to different sites, and, therefore, may disturb the enzyme structure in a different way.[Citation39]
Table 3. Effects of various metal ions on PPO activity of D. lotus.
PPO activity was measured in the presence of four different inhibitors: sodium azide, ascorbic acid, benzoic acid and sodium metabisulphite, using 4-methylcatechol as substrate. Each inhibitor was tested over a wide range of concentrations sufficient to reduce PPO activity by 50% or more. summarizes the IC50 values for each inhibitor. The most effective inhibitor was ascorbic acid. In contrast, sodium azide was an ineffective inhibitor of D. lotus PPO, with a similar inhibition profile having been reported for Turkish D. kaki PPO, using the same substrate.[Citation1] Ascorbic acid is commonly used as an anti-browning agent because of non-toxicity concerns. Inhibition by metabisulphite, with an IC50 of 0.118 mM, may be considered extremely strong, which is consistent with earlier reports indicating that thiol compounds are potent inhibitors of PPOs.[Citation1,Citation40] Reaction of sulphite with intermediate quinones results in the formation of sulphoquinones. These sulphoquinones inhibit PPO irreversibly, resulting in its complete inactivation.[Citation41] The inhibition mechanism for each compound is different and seems to depend on the reducing agent used as inhibitor. Inhibition by thiol compounds is mainly due to stable colourless products and/or binding to the PPO active site.[Citation42]
Table 4. Apparent IC50 of some inhibitors of PPO from D. lotus.
Anti-urease and anti-AChE activity
Plants have always been the main source of new drugs and folk medicines. Several naturally occurring medicinal plants, herbs, and fruit extracts have been shown to possess antimicrobial activity against H. pylori. Also, polyphenols, especially flavonoids, have been pointed out as notable H. pylori urease inhibitors.[Citation43] Our results showed that an aqueous extract of D. lotus had promising activity to inhibit urease up to 99.97 ± 0.59% at a final concentration of 12.00 mg/mL with an IC50 value of 1.55 ± 0.05 mg/mL (). It was reported that aqueous extracts of Pyrus elaeagnifolia exhibited strong antioxidant capacity and substantial xanthine oxidase and urease inhibition, with IC50 values of 10.75 ± 0.11 and 0.97 ± 0.03 mg/mL, respectively.[Citation29] It was found that Fagonia arabica L. whole plant extract and Casuarina equisetifolia L. fruit extract indicated a non-competitive inhibition mechanism on H. pylori urease.[Citation44] Plants are known to contain active metabolites which are useful in the treatment of various infectious diseases with little or no toxicity. Also, aqueous extract of D. lotus exhibited highly efficient anti-AchE activity with an IC50 value of 16.75 ± 0.11 mg/mL (). In another study, ethanolic propolis extracts exhibited variable inhibition effects on some clinically important enzymes, but the higher the phenolic content the lower the inhibition values (IC50 = 0.074 to 1.560 mg/mL). IC50 values of the P5 propolis sample having the highest total phenolic content, obtained from Zonguldak, for AchE, urease and xanthine oxidase were 0.081 ± 0.009, 0.080 ± 0.006, and 0.074 ± 0.011 µg/mL, respectively.[Citation45]
Table 5. Percentage inhibition and IC50 values of urease and AChE of D. lotus aquatic extract.
Conclusions
In the present study, PPO from D. lotus was purified and characterized for the first time. The optimum temperature for maximum enzyme activity was 60°C, which is much higher than the values commonly reported for PPOs from other Diospyros varieties and plants. PPO purified from D. lotus possesses diphenolase activity, having greatest substrate specificity to 4-methylcatechol. Ascorbic acid strongly inhibited the oxidative activity of D. lotus PPO. Further research is needed for a more detailed characterization of treatments during ripening and postharvest which would make it easier to obtain innovative and profitable D. lotus-derived products which could be used. Aqueous extract of D. lotus was a promising anti-urease and anti-AChE agent.
References
- Özen, A.; Colak, A.; Dincer, B.; Güner, S. A Diphenolase from Persimmon Fruits (Diospyros Kaki L., Ebenaceae). Food Chemistry 2004, 85, 431–437.
- Gao, H.; Cheng, N.; Zhou, J.; Wang, B.; Deng, J.; Cao, W. Antioxidant Activities and Phenolic Compounds of Date Plum Persimmon (Diospyros Lotus L.) Fruits. The Journal of Food Science and Technology 2014, 51, 950–956.
- Ayaz, F.A.; Kadıoǧlu, A.; Reunanen, M. Changes in Phenolic Acid Contents of Diospyros Lotus L. during Fruit Development. Journal of Agricultural and Food Chemistry 1997, 45, 2539–2541.
- Loizzo, M.R.; Said, A.; Tundis, R.; Hawas, U.W.; Rashed, K.; Menichini, F.; Frega, N.G.; Menichini, F. Antioxidant and Antiproliferative Activity of Diospyros lotus L. Extract and Isolated Compounds. Plant Foods for Human Nutrition 2009, 64, 264–270.
- Özel, A.; Colak, A.; Arslan, O.; Yildirim, M. Purification and Characterisation of a Polyphenol Oxidase from Boletus Erythropus and Investigation of Its Catalytic Efficiency in Selected Organic Solvents. Food Chemistry 2010, 119, 1044–1049.
- Navarro, J.L.; Terrega, A.; Sentandreu, M.A.; Sentandreu, E. Partial Purification and Characterization of Polyphenol Oxidase from Persimmon. Food Chemistry 2014, 157, 283–289.
- Núñez-Delicado, E.; Sojo, M.M.; García-Carmona, F.; Sánchez-Ferrer, A. Partial Purification of Latent Persimmon Fruit Polyphenol Oxidase. Journal of Agricultural and Food Chemistry 2003, 51, 2058–2063.
- Dayem, A.A.; Choi, H.-Y.; Kim, J.-H., Cho, S.-G. Role of Oxidative Stress in Stem, Cancer, and Cancer Stem Cells. Cancers 2010, 2, 859–884.
- Howes, M.J.; Houghton, P.J. Plants Used in Chinese and Indian Traditional Medicine for Improvement of Memory and Cognitive Function. Pharmacology Biochemistry and Behavior 2003, 75, 513–527.
- Houghton, P.J.; Howes, M.J.; Lee, C.C.; Steventon, G. Uses and Abuses of in Vitro Tests in Ethnopharmacology: Visualizing an Elephant. Journal of Ethnopharmacology 2007, 110, 391–400.
- Sahu, J.K.; Ganguly, S.; Kaushik, A. Triazoles: A Valuable Insight into Recent Developments and Biological Activities. Chinese Journal of Natural Medicines 2013, 11, 456–465.
- Kolcuoǧlu, Y. Purification and Comparative Characterization of Monophenolase and Diphenolase Activities from a Wild Edible Mushroom (Macrolepiota Gracilenta). Process Biochemistry 2012, 47, 2449–2454.
- Arslan, O.; Erzengin, M.; Sinan, S.; Ozensoy, O. Purification of Mulberry (Morus Alba L.) Polyphenol Oxidase by Affinity Chromatography and Investigation of Its Kinetic and Electrophoretic Properties. Food Chemistry 2004, 88, 479–484.
- Bradford, M.M. A Rapid and Sensitive Method for the Quantitation of Microgram Quantities of Protein Using the Principle of Protein Dye Binding. Analytical Biochemistry 1976, 72, 248–254.
- Espín, J.C.; Morales, M.; García-Ruiz, P.A.; Tudela, J.; García-Cánovas, F. Improvement of a Continuous Spectrophotometric Method for Determining the Monophenolase and Diphenolase Activities of Mushroom Polyphenol Oxidase. Journal of Agricultural and Food Chemistry 1997, 45, 1084–1090.
- Batista, K.A.; Batista, G.L.A.; Alves, G.L.; Fernandes, K.F. Extraction, Partial Purification and Characterization of Polyphenol Oxidase from Solanum Lycocarpum Fruits. Journal of Molecular Catalysis B: Enzymatic 2014, 102, 211–217.
- Lineweaver, H.; Burk, D. The Determination of Enzyme Dissociation Constants. Journal of the American Chemical Society 1934, 56, 658–666.
- Colak, A.; Özen, A.; Dincer, B.; Güner, S.; Ayaz, F.A. Diphenolases from Two Cultivars of Cherry Laurel (Laurocerasus Officinalis Roem.) Fruits at an Early Stage of Maturation. Food Chemistry 2005, 90, 801–807.
- Laemmli, U.K. Cleavage of Structural Proteins During the Assembly of the Head of Bacteriophage T4. Nature 1970, 227, 680–685.
- Mentese, M.Y.; Bayrak, H.; Uygun, Y.; Merme, A.; Ulker, S.; Karaoglu, S.A.; Demirbas, N. Microwave Assisted Synthesis of Some Hybrid Molecules Derived from Norfloxacin and Investigation of Their Biological Activities. European Journal of Medicinal Chemistry 2013, 67, 230–242.
- Ellman, G.L.; Courtney, K.D.; Andres, V.; Featherstone, R.M. A New and Rapid Colorimetric Determination of Acetylcholinesterase Activity. Biochemical Pharmacology 1961, 7, 88–95.
- Öz, F.; Colak, A.; Özel, A.; Sağlam Ertunga, N.; Sesli, E. Purification and Characterization of a Mushroom Polyphenol Oxidase and Its Activity In Organic Solvents. Jounal of Food Biochemistry 2013, 37, 36–44.
- Dogan, S.; Turan, P.; Dogan, M.; Arslan, O.; Alkan, M. Purification and Characterization of Ocimum Basilicum L. Polyphenol Oxidase. Journal of Agricultural and Food Chemistry 2005, 53, 10224–10230.
- Gong, Z.; Li, D.; Liu, C.; Cheng, A.; Wang, W. Partial Purification and Characterization of Polyphenol Oxidase and Peroxidase from Chestnut Kernel. Food Science and Technology 2015, 60, 1095–1099.
- Colak, A.; Sahin, E.; Yildirim, M.; Sesli, E. Polyphenol Oxidase Potentials of Three Wild Mushroom Species Harvested from Lişer High Plateau, Trabzon. Food Chemistry 2007, 103, 1426–1433.
- Gao, Z.J.; Han, X.H.; Xiao, X.G. Purification and Characterisation of Polyphenol Oxidase from Red Swiss Chard (Beta Vulgaris Subspecies Cicla) Leaves. Food Chemistry 2009, 117, 342–348.
- Guo, L.; Ma, Y.; Shi, J.; Xue, S. The Purification and Characterisation of Polyphenol Oxidase from Green Bean (Phaseolus Vulgaris L.). Food Chemistry 2009, 117, 143–151.
- Dogan, S.; Salman, U. Partial Characterization of Lettuce (Lactuca Sativa L.) Polyphenol Oxidase. European Food Research and Technology 2007, 226, 93–103.
- Baltas, N. Investigation of a Wild Pear Species (Pyrus Elaeagnifolia subsp. Elaeagnifolia Pallas) from Antalya, Turkey: Polyphenol Oxidase Properties and Anti-Xanthine Oxidase, Anti-Urease and Antioxidant Activity. International Journal of Food Properties 2016, DOI:10.1080/10942912.2016.1171777
- Bravo, K.; Osorio, E. Characterization of Polyphenol Oxidase from Cape Gooseberry (Physalis Peruviana L.) Fruit. Food Chemistry 2016, 197, 185–190.
- Talley, K.; Alexov, E. On the pH-Optimum of Activity and Stability of Proteins. Proteins 2010, 78, 2699–2706.
- Beena, P.; Gowda, L.R. Purification and Characterization of a Polyphenol Oxidase from the Seeds of Field Bean (Dolichos Lablab). Journal of Agricultural and Food Chemistry 2000, 48, 3839–3846.
- Oktay, M.; Kufrevioglu, I.; Kocacaliskan, I.; Sakiroglu, H. Polyphenol Oxidase from Amasya Apple. Journal of Food Science 1995, 60, 495–499.
- Faiz, Ö. Purification and Characterization of a Polyphenol Oxidase from Cimin Grape (Vitis Vinifera spp. Cimin). Research Journal of Biotechnology 2016, 11, 1–8.
- Dogan, M.; Arslan, O.; Dogan, S. Substrate Specificity, Heat Inactivation and Inhibition of Polyphenol Oxidase from Different Aubergine Cultivars. International Journal of Food Science and Technology 2002, 37, 415–423.
- Palma-Orozco, G.; Ortiz-Moreno, A.; Dorantes-Alvarez, L.; Sampedro, J.G.; Nájera, H. Purification and Partial Biochemical Characterization of Polyphenol Oxidase from Mamey (Pouteria Sapota). Phytochemistry 2011, 72, 82–88.
- Sun, H.J.; Wang, J.; Tao, X.M.; Shi, J.; Huang, M.Y.; Chen, Z. Purification and Characterization of Polyphenol Oxidase from Rape Flower. Journal of Agricultural and Food Chemistry 2012, 60, 823–829.
- Gawlik-Dziki, U.; Złotek, U.; Świeca, M. Characterization of Polyphenol Oxidase from Butter Lettuce (Lactuca Sativa var. Capitata L.). Food Chemistry 2008, 107, 129–135.
- DiTusa, C.A.; Christensen, T.; McCall, K.A.; Fierke, C.A.; Toone, E.J. Thermodynamics of Metal Ion Binding. 1. Metal Ion Binding by Wild-Type Carbonic Anhydrase. Biochemistry 2001, 40, 5338–5344.
- Dincer, B.; Colak, A.; Aydin, N.; Kadioglu, A.; Güner, S. Characterization of Polyphenoloxidase from Medlar Fruits (Mespilus Germanica L., Rosaceae). Food Chemistry 2002, 77, 1–7.
- Ferrer, O.J.; Otwell, W.S.; Marshall, M.R. Effect of Bisulphite on Lobster Shell Phenoloxidase. Journal of Food Science 1989, 4, 478–480.
- Valero, E.; Garcia-Carmona, F. Hysteresis and Cooperative Behavior of a Latent Plant Polyphenoloxidase. Plant Physiology 1992, 98, 774–776.
- Lespade, L.; Bercion, S. Theoretical Study of the Mechanism of Inhibition of Xanthine Oxidase by Flavonoids and Gallic Acid Derivatives. The Journal of Physical Chemistry 2010, 114, 921–928.
- Amin, M.; Anwar, F.; Naz, F.; Mehmood, T.; Saari, N. Anti Helicobacter Pylori and Urease Inhibition Activities of Some Traditional Medicinal Plants. Molecules 2013, 18, 2135–2149.
- Baltas, N.; Yildiz, O.; Kolayli, S. Inhibition Properties of Propolis Extracts to Some Clinically Important Enzymes. Journal of Enzyme Inhibition and Medicinal Chemistry 2016, DOI:10.3109/14756366.2016.1167049