ABSTRACT
A highly sensitive high-performance liquid chromatography coupled with electrospray ionization quadrupole time-of-flight mass spectrometry method was established for the identification of procyanidin components in the lotus seedpod, mangosteen pericarp, and camellia flower. It was shown that the main procyanidins of lotus seedpod were monomer, dimmer, and trimer-procyanidins, in which dimers and trimers were in the majority (80.8%). However, mangosteen pericarp and camellia flower procyanidins were mostly composed of monomers (47.7 and 62.4%, respectively). In addition, their antioxidant activities were also compared and the strongest antioxidant activities were found in lotus seedpod (1,1-diphenyl-2-picrylhydrazyl: 1.57 μmol Trolox equivalent/g dry weight; 2’-azino-bis-(3-ethyl-benzthiazoline-6-sulphonate acid): 1.23 μmol Trolox equivalent/g dry weight; Fe2+/H2O2: 2.17 μmol Trolox equivalent/g dry weight), followed by mangosteen pericarp and camellia flower. Moreover, the lotus seedpod, mangosteen pericarp, and camellia flower extracts exhibited considerable dose-dependent protective effects on the H2O2-induced damage of human umbilical vein endothelial cells. Among them, lotus seedpod extracts showed relatively stronger cell-based antioxidant enzyme activities and lower contents of malondialdehyde and nitric oxide than those of camellia flower and mangosteen pericarp. From these results, lotus seedpod, mangosteen pericarp, and camellia flower would be available agricultural wastes and their utilization may produce easier access of functional ingredients for medicinal exploration.
Introduction
Increasing attention has been paid to the potential beneficial effects of flavonoids and other phenolic compounds. Recent studies[Citation1,Citation2] have associated the regular consumption of flavonoid-rich foods with a decreased risk for cardiovascular diseases and select cancers, although a causative relationship has not been proven. One of the mechanisms is related to their important antioxidant properties observed in several chemical and biological systems, which were thought to contribute to the putative positive health effects deriving from consuming these phytochemicals.[Citation3] Procyanidins, widely distributed in plants, have been proven to improve human health by affecting the cellular and physiological processes.[Citation4] Procyanidins were reported to reduce the risk of many chronic diseases, and exhibit cardioprotective, antibacterial, anti-inflammatory, immune-stimulating, and estrogenic activities.[Citation5]
Lotus (Nelumbo nucifera Gaert) is widely cultivated in China and the inedible part lotus seedpod (LS) is usually discarded after the harvest of lotus seeds except occasionally used as a hemostatic.[Citation6] Mangosteen (Garcinia mangostana Linn) is commonly distributed in the tropical rainforest in many countries such as Indonesia, Thailand, Malaysia, Burma, and the Philippines. Mangosteen pericarp (MP) has been widely used in Southeast Asia as a medicinal agent in the treatment of inflammation and diarrhea, skin infections, and wounds.[Citation7,Citation8] Camellia flower (CF; Camellia Japonica L) is originated from the subtropical regions of China, Japan and thought to be beneficial to human health including cough suppressant, deodorization, expectorant, and skin care.[Citation9]
The materials used in this article are inedible parts of plants with few practical purposes at present. However, these materials have been reported to be rich in procyanidins with many functions. For example, Duan et al.[Citation10] reported the inhibitory effect of LS procyanidins on mouse melanoma B16. Ohno et al.[Citation11] found that MP procyanidins was useful in ameliorating skin elasticity and the anti-cariogenic properties of CF was reported by Hamilton et al.[Citation12] It is true that procyanidins components and their antioxidant activities are related to the cultivar, maturity, color, growth location, season, environment, and others. It has been reported by previous researches.[Citation13,Citation14] In this study, the most common and consumption LS, MP, and CF in China were chosen as the samples. Procyanidins in the LS, MP, and CF were identified by high-performance liquid chromatography coupled with electrospray ionization quadrupole time-of-flight mass spectrometry (HPLC-QTOF-MS), and their antioxidant activities were also compared by four different in vitro assays. In addition, the cellular experiments were also conducted on H2O2-induced cell injury. The full information of procyanidins in the LS, MP, and CF will make it completed to explore the functional foods.
Materials and methods
Materials
LS (cultivar, green) was grown at Guangchang Country (Jiangxi, China 116°24’ E, 26°35’ N) in July 2014. Mangosteen (Malaysia, purple) and CF (cultivar, red) were Jiangxi products, all purchased from a local grocery store (July 2014). All of the samples were all obtained after fully harvested. 1,1-diphenyl-2-picrylhydrazyl (DPPH), 2, 2’-azino-bis-(3-ethyl-benzthiazoline-6- sulphonate acid; ABTS) were obtained from Sigma-Aldrich (USA). Human umbilical vein endothelial cells (HUVECs) were obtained from the medical school at Nanchang University. The malondialdehyde (MDA), superoxide dismutase (SOD), glutathione peroxidase (GSH-Px), nitric oxide (NO), ET-1 assay kits were purchased from Jiancheng Biological Engineering Institute (Nanjing, China). Acetone, trichloromethane, diethyl ether, ethyl acetate, ethanol methanol (analytical grade), and formic acid, acetonitrile (gradient grade) were all purchased from reliable sources.
Chemical analysis
Pretreatment of samples
The extraction and purification of procyanidin were similar to Liu et al.[Citation15] Briefly, 70% (v/v) acetone (w/v = 1:2) with 1 g/L of L-ascorbic acid was used as the extractant. After 12 h of extraction, the mixture was filtered, and the supernatants were collected and pooled. The filtrate was evaporated using a RE-85Z Rotary Evaporator (Valley instrument factory, China) at 45°C and then edulcorated with trichloromethane, diethyl ether, and ethyl acetate (each 100 mL). The residual organic solvent was again vacuum-dried (rotary evaporator) and freeze-dried (–50°C, 0.9 MPa, SJIA-10N vacuum freeze dryer, Ningbo, China) into powder.
The preliminary purification was carried out using AB-8 resin column (Φ 1.5 × 35 cm), with water and 50% ethanol (200 mL each) as the eluants. The ethanol part was collected and evaporated. Further purification was conducted by Sephadex LH-20 column (Φ 3.2 × 40 cm). The column was eluted with 0, 25, and 50% methanol–water reagents and 70% acetone, and the ultimate organic eluant was collected and freeze-dried to powder. The products were referred as procyanidin extracts from LS (LSPC), MP (MPPC), and CF (CFPC).
HPLC-QTOF-MS analysis
The qualitative analysis of procyanidins was carried out by HPLC-QTOF (Agilent Technologies, USA). Each powder was dissolved by methanol at concentration of 80 µg/mL. Then these samples were eluted by an ODS C18 column (250 × 4.6 mm, 5 μm, Agilent Technologies) at 25°C and the ultraviolet (UV) detection wavelength was set at 280 nm. Mobile phases were 0.1% formic acid in A: water and B: acetonitrile with a flow rate of 0.5 mL/min. The solvent gradient was conducted as follows: 0–15 min, 5–8% B; 15–25 min, 8–13% B; 25–26 min, 13–5% B; 26–35 min, 5% B. The mass data was collected in positive mode and m/z was set in the range of 50–1700. The parameters of full scans were as follows: source voltage, –4200 V; collision energy, –30 V; capillary temperature 400°C with N2 as curtain gas, 50 arbitrary units (a.u.); the nebulizing gas pressure, 40 psi.
Antioxidant activity
The DPPH free radical scavenging activity was determined by measuring the absorbance decrease of DPPH solution at 517 nm based on Sebastián’s method.[Citation16] The ABTS radical cation decolorization assay was based on Lu’s method.[Citation17] The ferric reducing antioxidant power (FRAP) assay was carried out according to the method of Zhang et al.[Citation18] The hydroxyl radical scavenging activities were determined using the method of Mustafa.[Citation19]
Cell culture
HUVECs were cultured in a dulbecco’s modified eagle medium (DMEM) medium supplemented with 10% fetal Bovine Serum (FBS), 0.03 mg/mL cell growth supplement, 0.1 mg/mL heparin, 100 g/mL streptomycin, and 100 U/mL penicillin. Cells were grown in a humidified atmosphere (95%) of 5% CO2 with media replenishment every 3 days. After four to five generations, the cells were used for the following experiments.
Cytotoxicity and cytoprotective effect
The cytotoxicity of LSPC, MPPC, and CFPC on HUVECs were measured by the 3-(4,5-Dimethylthiazol-2-yl)-2,5-diphenyltetrazolium bromide (MTT) method.[Citation20] The dosage of LSPC, MPPC, and CFPC on cells were given with different concentrations (10, 50, 100, 200, 400, and 800 μg/mL), while the control group was added with the same volume of phosphate buffer solution (PBS). In the cytoprotective experiment, cells were, first, treated by 150 µmol/L H2O2 for 4 h and then cultured with procyanidin samples at different contractions (10, 50, 100, and 200 μg/mL, respectively). The optical density (OD) of every group was measured by an enzyme-linked immuno sorbent assay (ELISA) reader at the wavelength of 490 nm. Cell viability was calculated with the following formula: cell viability (%) = OD (experimental group) /OD (control group) × 100%.
MDA, SOD, GSH-Px, NO, and ET-1 assays
Cell grouping and cultivation were the same as mentioned previously. The dissociated HUVECs were centrifuged at 4°C for 10 min at 3000 × g and then 600 µL lysis buffer was added, and the lysates were centrifuged at 28,000 × g for another 15 min. The supernatant was collected for the analysis of MDA, SOD, GSH-Px, NO, and ET-1 contents. MDA level was measured by thiobarbituric acid (TBA) method.[Citation21] The inhibition of xanthine/xanthine oxidase-mediated reduction on 2-(4-iodophenyl)-3-(4-nitrophenol)-5-phenyltetrazo-lium chloride (INT) was considered as an evaluation index for SOD activity.[Citation22] GSH-Px activity was assayed by 5,5’-Dithiobis(2-nitrobenzoic acid (DNTB) method,[Citation23] and colorimetric method[Citation24] was used to determine NO level in the cells. ET-1 content was determined by ELISA kit according to the instructions.
Statistical analysis
Results were expressed as mean ± SD of three independent extractions. Variance and significant differences were analyzed by the analysis of variance (ANOVA) followed by Duncan’s multiple range tests. The difference between groups was considered as significant difference at p-values < 0.05.
Results and discussion
Qualitative analysis of procyanidins
HPLC-QTOF-MS is relatively useful method for the compound identification by combining the pseudomolecular ion mass with the MSn fragmentation, and also by comparison with authentic standards.[Citation25] QTOF-MS has better sensitivity and selectivity compared to other equipments and has wide applications for the identification. In this study, the main peaks of LSPC, MPPC, and CFPC at 280 nm were shown in and the detailed information of each peak, including the retention times (tR), observed m/z, error (ppm), and MS spectra, was shown in and .
Table 1. The procyanidin compounds in lotus seedpod, mangosteen pericarp and camellia flower identified by HPLC−QTOF-MS.[Citation26–Citation30]
The procyanidins profiles of LSPC were quite different from those of CFPC and MPPC. A total of 10 peaks were identified. Peak 2′ and 9′ were identified as catechin and gallocatechin (molecular weight 290 and 306, respectively) in the positive mode. Peaks 5′, 6′, and 7′ with m/z of 579.1484 were indicative of procyanidin dimers (molecular weight, 578).[Citation26] Peak 8′ with the spectra signal of m/z 867 corresponded to a C–C–C procyanidin trimers in line with Gu et al.[Citation27] Peak 1′ with the spectra showing [M-H]+ of 595.1445 was identified as gallocatechin/epigallocatechin bounded to catechin/ecatechin. Peak 3′, 4′ and 10′ were identified as 1 GC/2 C procyanidin trimers, which meant the procyanidins was composed of two catechin/epicatechin units and one gallocatechin/epigallocatechin unit.[Citation28]
The HPLC chromatograms of purified CFPC and MPPC were quite similar, in which peaks 1, 5, 6, 7, and 9 were in common (Fig. 1). A total of 10 peaks were identified in CFPC. Peaks 1, 2, 5, and 9 with different tR (8.067, 8.953, 13.274, and 18.540 min) had the same pseudomolecular ions [M−H]+ of 579.1460 were identified as procyanidin dimers. Peaks 3, 4, and 6 showed m/z of 291.0845 and the MS data combined with the ultraviolet-visible (UV-Vis) spectra led to the tentative identification of catechin or ecatechin. M/z of 867.2081 in peak 7 was identified to be procyanidin trimers. Peaks 12 and 14 with m/z of 595.1415 were gallocatechin/epigallocatechin bounded to catechin/ecatechin.[Citation29]
Peaks 1, 5, 11, and 13 in MPPC, with tR of 8.193, 13.36, 20.713, and 28.34 min and the same m/z of 579.1457, were identified to be procyanidin dimers. Peak 6 with m/z of 291.0864 was determined to be catechin/ecatechin. Peaks 7, 8, 9, and 10 with a pseudomolecular ion of 867.2086 were recognized as procyanidin trimmers.[Citation30] The procyanidins contents in the purified CF, MP, and LS were measured by comparing the area of HPLC chromatogram. The result showed that CF, MP, and LS procyanidins were consisted of monomers (62.4, 47.7, and 20.9%, respectively), dimers (32.5, 24.1, and 43.5%, respectively), and trimer (5.1, 26.0, and 37.4%, respectively).
Chemical-based antioxidant activity
LSPC showed greater DPPH free radical scavenging activity than those of CFPC and MPPC (1.57, 1.07, and 1.01 μmol Trolox equivalent/g dry weight (DW), respectively, ). Meanwhile, the same tendency was found in ABTS assays (1.23, 0.97 and 0.96 μmol TE/g DW, respectively). MPPC and CFPC exhibited no significant differences on both DPPH and ABTS antioxidant activities. In FRAP assay, MPPC exhibited relatively higher reducing power than CFPC and LSPC. Besides, the results of hydroxyl radical scavenging activity showed that LSPC exhibited the highest radical scavenging activity (2.17 μmol TE/g DW; p < 0.05), while CFPC and MPPC showed almost the same antioxidant activities (1.78 and 1.96 μmol TE/g DW, respectively).
Table 2. The antioxidant activities of LSPC, MPPC, and CFPC.
The antioxidant activities of CFPC, MPPC, and LSPC were different in this study, which might be related to the contents and the forms of procyandins. In our study, the antioxidant activities followed the order of LS > MP > CF, which was the same as the procyanidin contents reported before.[Citation8,Citation31,Citation32] We might get the conclusion that, the antioxidant activity of procyanidins was related to its procyanidin content. On the other side, the degree of procyandins polymerization (from monomer to pentamer) may also influence the antioxidant activity of plant extracts. Ana et al.[Citation33] measured the protection effect of different degree of procyandins against peroxyl radicals, and the results showed that the protective effect increased up to fraction II (average molecule weight of 800). That being said, with higher degree of polymerization, the antioxidant activities of procyanidins increased. In our study, dimmer and trimmer procyanidins were the major structures in LS, while in CF and MP extracts, mono-procyanidin made up the majority of the purified extracts. It has been reported that dimmer and trimmer procyanidins exhibited better antioxidant activities than mono-procyanidin[Citation33] and that might be another reason why LSPC showed the better antioxidant activity than MPPC and CFPC.
Cytotoxicity effects
The toxicity of procyanidin extracts on cells was measured by exposing HUVECs in LSPC, MPPC and CFPC (0–800 μg/mL) for 4 h. Compared to the control group, there were no significant cytotoxic effects for LSPC, MPPC, and CFPC treated groups in HUVECs when the sample concentration was below 200 μg/mL. On the contrary, a slight enhancement effect on cell viability was found (;
Figure 3. Effects of LSPC, MPPC, and CFPC at different concentrations on HUVECs. Control group meant cells without treatment of procyanidins, and the experimental groups were treated with LSPC, MPPC, and CFPC at 10, 50, 100, 200, 400, and 800 μg/mL, respectively. The values were expressed as means ± SD (n = 3). Different letters indicate significant difference by ANOVA, p < 0.05.
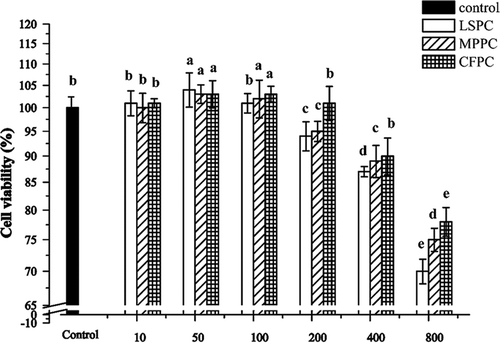
It has been found that low concentrations of grape seed extract (GSE; 1 mg gallic acid equivalent/mL) could intensify the inhibitory action of platelet reactivity on HUVECs, which was due to the protective effects of procyanidins against cardiovascular diseases.[Citation34] However, GSE at high concentrations (up to 10 mg/mL) could significantly impair endothelial cell proliferation in vitro. Agarwal et al.[Citation35] also reported that GSE exhibited great anti-angiogenic potential by inhibiting the growth of HUVECs. Moreover, GSE significantly inhibited the cell growth (<91%) and viability (<64%) at concentrations higher than 25 mg/mL. These results demonstrated that GSE could be toxic to HUVECs at certain high concentrations. In our experiment, the effects of LSPC, MPPC, and CFPC on HUVECs were in line with the previous results. Procyanidins showed no cytotoxic effect below 200 μg/mL, but significant toxicity with higher doses at 400–800 μg/mL. Therefore, the concentrations less than 200 μg/mL were used for the further study.
Cytoprotective effect
The cytoprotective effects of plant extracts were evaluated against the H2O2-induced oxidative damage. A significant decrease of cell viability (almost 50%) was observed by treatment with H2O2 alone ()
Figure 4. Effects of LSPC, MPPC, and CFPC on cell viability in H2O2 injured HUVECs. The results were expressed as percentage of control, and each value represents mean ± SD of three independent experiments. Control group meant cells without treatment of procyanidins. H2O2 group meant cells treated with 150 μM H2O2 for 8 h. The experimental groups were treated with 150 μM H2O2 + 10, 50, 100, 200 μg/mL LSPC, MPPC, and CFPC, respectively. Different letters (a, b, c) mean significantly different (p < 0.05) with each other.
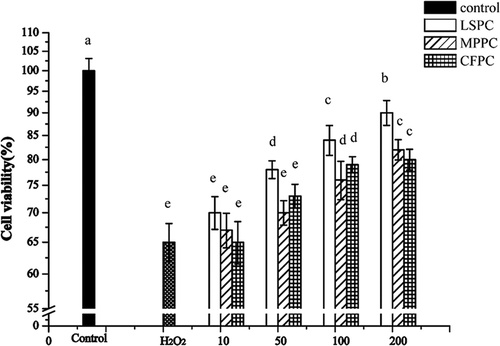
Lipid peroxidation in HUVECs
MDA is generally considered as a marker of oxidative damage,[Citation37] since it has strong toxicity to the cell membrane and would cause the decrease of cell viability.[Citation38] The free radical would react with polyunsaturated fatty acids leading to lipid peroxidation, and yielding excessive MDA. As shown in , the MDA content in HUVECs exposed to H2O2 was significantly increased as compared to the control group (from 31.25 to 66.48 nmol/mg prot). However, after pretreatment with LSPC, MPPC, and CFPC at the concentration of 50, 100, and 200 μg/mL, the MDA contents were significantly reduced (p < 0.05) in a dose-dependent manner. Among these three sources of procyanidins, LSPC showed the best inhibitory effect of MDA, indicating best prevention effect against lipid peroxidation.
Table 3. The effects of LSPC, MPPC, and CFPC on MDA content, SOD and GSH-Px activities, NO and ET-1 contents in H2O2-induced HUVECs.
Activities of antioxidant enzymes in HUVECs
In this study, the effects of LSPC, MPPC, and CFPC on the alteration in the activities of H2O2-induced antioxidant enzymes, such as SOD and GSH-Px were also investigated. From the results, H2O2 could significantly reduce the SOD and GSH-Px activities by approximately 40 and 50%, respectively (). But after treatment of LSPC, MPPC, and CFPC, enzymes activities were evidently up-regulated in a dose-dependent manner. Although all of LSPC, MPPC, and CFPC treated groups enhanced the enzyme activities, the highest SOD and GSH-Px activities were still found in the control group (150 and 189 U/mg prot, respectively). In addition, LSPC and MPPC seemed to be more effective on inhibiting the cell oxidation than CFPC at the same concentrations (). This might be caused by the procyanidins contents and the degrees of polymerization in different samples. LSPC and MPPC showed greater contents of procyanidins than CFPC. What is more, dimmer and trimmer procyanidins contributed more proportions in LSPC and MPPC than those in CFPC.
The cellular antioxidant enzymes including SOD and GSH-Px played important roles in defending the ROS injury.[Citation39] SOD converts reactive superoxide into less harmful hydrogen peroxide and finally breaks it down into oxygen and water. Simultaneously, lipid hydroperoxides could be catalyzed to hydroxides with the function of GSH-Px.[Citation40] Procyanidins worked as a powerful antioxidant possibly due to its free radical scavenging activity. It was suggested that LSPC had rejuvenation effect on the antioxidant and cholinergic systems with ameliorating memory impairment of cognitively impaired aged rats.[Citation41] Zhou et al.[Citation7] found that the antioxidant effect of MP was mainly because of its procyanidins, and was recognized as potent peroxyl radical scavengers by the high oxygen radical scavenging capacity (1.7 × 104 μmol TE/g), much higher than those of pine bark and grape seed (7.5 × 103 and 1.0 × 104 μmol TE/g, respectively).[Citation8] The CF extracts exerted strong hydroxyl radical scavenging effects in the Fenton reaction system and NO suppressing effects in LPS-induced RAW 264.7 cells.[Citation32] Increased SOD and GSH-Px activities in procyanidin-treatment groups revealed that procyanidins may enhance the anti-free radical activities in HUVECs by protecting the antioxidant enzyme system and thus reducing the cellular injury.
NO release and ET-1 production
It showed that H2O2 led to a decrease of NO production (from 67.96 to 38.25 mmol/L, ). However, pre-incubation with LSPC, MPPC, and CFPC at different concentrations could significantly reverse the decline. These results were in parallel with the increases of SOD and GSH-Px activities. Briefly, all doses of LSPC exhibited greater NO regulation effect than those of CFPC and MPPC at the same concentrations, indicating better antioxidant activity than CFPC and MPPC.
The ET-1 protein expression was monitored by ELISA method. Obvious increases of ET-1 expression were observed after treated alone with H2O2 (from 122 to 161 pg/L, p < 0.05, ). However, this elevation was remarkably inhibited by pre-incubation with LSPC, MPPC, and CFPC. At concentrations from 10 to 200 μg/mL, the ET-1 contents decreased from 152 to 127 pg/L in LSPC-treated groups, from 154 to 129 pg/L in MPPC and from 166 to 141 pg/L in CFPC. Almost each concentration of samples expressed significant ET-1 inhibition effect compared to the H2O2-treated group (except for 10 μg/mL).
NO, synthesized by the action of endothelial NO synthase (eNOS) in many animal cells, is always recognized as a marker of inflammation for its high immune and inflammatory responses.[Citation42] ET-1 is also produced by endothelial cells and is probably the major isoform in the cardiovascular system.[Citation43] Oligomeric procyanidins (OPC) have been attributed with several cardioprotective effects including the induction of eNOS and NO-mediated vasorelaxation in rat and human arteries,[Citation44] reducing blood pressure and improving cardiac performance in an experimental model of ischemia reperfusion damage.[Citation45] As recently reported by Corder et al.,[Citation46] OPC were also responsible for the inhibition effect of ET-1 in endothelial cells, proving the antiatherosclerotic actions of regular red wine consumption. The results in our study demonstrated that LSPC, MPPC, and CFPC commonly increased the NO release and inhibited the ET-1 production, showing their strong antioxidant activities and possible effects on the cardiovascular system.
Conclusion
In summary, LS, MP, and CF showed considerable variations in their procyanidin profiles determined by HPLC-QTOF-MS, as well as overall antioxidant activities measured by in vitro chemical assays and relevant biological cellular methods. It was indicated that antioxidant function of LS, MP, and CF might predominantly depend on their procyanidin contents and constituents. It was also found that LS had the highest level of procyanidins and the main procyanidins were dimmers (43.5%) and trimmers (37.4%). While, MP and CF mainly contained mono-procyanidins (47.7 and 62.4%, respectively), was related to the antioxidant activity (LSPC > MPPC > CFPC). Moreover, LSPC exhibited stronger antioxidant activity and protective effect on HUVECs compared to MPPC and CFPC. LS, MP, and CF were found to be excellent sources of natural antioxidants for health promotion, and it is well worth considering utilization of these agricultural wastes for the production of functional ingredients or effective constituents for medicinal exploration.
Funding
This project was funded by the Natural Science Funds of Jiangxi (Project No. 20142BAB214003; 20132BAB214002); National Natural Science Foundation of China (Project No. 31301578); and Science Foundation Project of Jiangxi Provincial Department of Education (Project No. GJJ13024).
Additional information
Funding
References
- Rasmussen, S.E.; Frederiksen, H.; Struntze, K.K.; Poulsen, L. Dietary Proanthocyanidins: Occurrence, Dietary Intake, Bioavailability, and Protection Against Cardiovascular Disease. Molecular Nutrition and Food Research 2005, 49, 159–174.
- Santosh, K.K.; Mohammad A. Grape Seeds: Ripe for Cancer Chemoprevention. Cancer Prevention Research 2013, 6, 617–621.
- Hollman, P.C.; Cassidy, A.; Comte, B.; Heinonen, M.; Richelle, M.; Richling, E.; Serafini, M.; Scalbert, A.; Sies, H.; Vidry, S. The Biological Relevance of Direct Antioxidant Effects of Polyphenols for Cardiovascular Health in Humans Is Not Established. Journal of Nutrition 2011, 342, 989–1009.
- Santos-Buelga, C.; Scalbert, A. Proanthocyanidins and Tannin Like Compounds-Nature, Occurrence, Dietary Intake and Effects on Nutrition and Health. Journal of the Science of Food and Agriculture 2000, 80, 1094–1117.
- Gong, Y.S.; Guo, J.; Hu, K.; Gao, Y.Q.; Xie, B.J.; Sun, Z.D.; Yang, E.N.; Hou, F.L. Ameliorative Effect of Lotus Seedpod Proanthocyanidins on Cognitive Impairment and Brain Aging Induced by D-Galactose. Experimental Gerontology 2016, 74, 21–28.
- Sui, Y.; Xiao, J.; Li, S.Y.; Li, X.P.; Xie, B.J.; Sun, Z.D. Combination of Procyanidins Extracted from Lotus Seedpod and N-Acetyl Cysteine Ameliorates Scopolamine-Induced Memory Impairment in Mice. Journal of Food and Nutrition Research 2015, 3, 464–470.
- Zhou, H.C.; Lin, Y.M.; Wei, S.D.; Tam, N.F. Structural Diversity and Antioxidant Activity of Condensed Tannins Fractionated from Mangosteen Pericarp. Food Chemistry 2011, 129, 1710–1720.
- Fu, C.; Loo, A.E.; Chia, F.P.; Huang, D.J. Oligomeric Proanthocyanidins from Mangosteen Pericarps. Journal of Agriculture and Food Chemistry 2007, 55(19), 7689–7694.
- Yoshikawa, M.; Wang, T.; Sugimoto, S.; Nakamura, S.; Nagatomo, A.; Matsuda, H.; Harima, S. Functional Saponis in Tea Flower (Flower Buds of Camellia Sinensis): Gastroprotective and Hypoglycemic Effects of Floratheasaponins and Qualitative And Quantitative Analysis Using HPLC. Yakugaku Zasshi 2008, 128, 141–151.
- Duan, Y.Q.; Zhang, H.H.; Xu, F.F.; Xie, B.J.; Yang, X.W.; Wang, Y.; Yan, Y.S. Inhibition Effect of Procyanidins from Lotus Seedpod on Mouse B16 Melanoma in Vivo and in Vitro. Food Chemistry 2010, 122, 84–91.
- Ohno, R.; Moroishi, N.; Sugawa, H.; Maejima, M.S.; Yamanaka, M.; Nagai, M.; Yoshimura, M.; Amakura, Y.; Nagai, R. Mangosteen Pericarp Extract Inhibits the Formation of Pentosidine and Ameliorates Skin Elasticity. Journal of Clinical Biochemistry and Nutrition 2015, 57, 27–32.
- Hamilton-Miller, J.M.T. Anti-Cariogenic Properties of Tea (Camellia Sinensis). Journal of Medical Microbiology 2001, 50, 299–302.
- Oracza, J.; Nebesnya, E. Antioxidant Properties of Cocoa Beans (Theobroma Cacao L.): Influence of Cultivar and Roasting Conditions. International Journal of Food Properties 2015, DOI:10.1080/10942912.2015.1071840
- Martí, N.; Lizama, V.; Verdú, J.A.; Muñoz, N.; Aleixandre, J.L.; Saura, D. Prediction of Phenolic Composition of Monastrell and Tempranillo Wines: Correlation Between Phenolic Content and Traditional Variables of Fruit Maturity. International Journal of Food Properties 2015, 18, 465–479.
- Liu, X.R.; Xie, R.P.; Fan, Y.W.; Hu, J.N.; Luo, T.; Li, H.Y.; Deng, Z.Y. Polymerization of Proanthocyanidins Catalyzed by Polyphenol Oxidase from Lotus Seedpod. European Food Research and Technology 2014, 238, 727–739.
- Sebastián, Z.; Luis, G.; Roxana, O.; Sergio, W.; Elba, L. Phenolic Compound Identification and Antioxidant Capacity of Alperujo Extracts from Region del Maule, Chile. International Journal of Food Properties 2015, DOI:10.1080/10942912.2015.1092162
- Lu, J.; Qin, P.Z.; Han, X.; Wang, Y.P.; Li, Z.H. Evaluation of Antioxidant and Antibacterial Properties of Extracts from Trollius Chinensis Bunge. European Food Research and Technology 2015, 240, 301–310.
- Zhang, B.; Deng, Z.Y.; Ramdath, D.D.; Tang, Y.; Chen, P.X.; Liu, R.H.; Liu, Q.; Tsao, R. Phenolic Profiles of 20 Canadian Lentil Cultivars and Their Contribution to Antioxidant Activity and Inhibitory Effects on α-Glucosidase and Pancreatic Lipase. Food Chemistry 2015, 172, 862–872.
- Mustafa, O.; Burcu, B.; Kubilay, G.; Resat, A. Hydroxyl Radical Scavenging Assay of Phenolics and flavonoids with a Modified Cupric Reducing Antioxidant Capacity (CUPRAC) Method Using Catalase for Hydrogen Peroxide Degradation. Analytica Chimica Acta 2008, 616, 196–206.
- Chang, W.; Lim, S.; Song, H.; Song, B.W.; Kim, H.J.; Cha, M.J.; Sung, J.M.; Kim, T.W.; Hwang, K.C. Cordycepin Inhibits Vascular Smooth Muscle Cell Proliferation. European Journal of Pharmacology 2008, 597, 64–69.
- Luo, T.; Xia, Z. A Small Dose of Hydrogen Peroxide Enhances Tumor Necrosis Factor-Alpha Toxicity in Inducing Human Vascular Endothelial Cell Apoptosis: Reversal with Propofol. Anesthesia and Analgesia 2006, 103, 110–116.
- Stroher, D.J.; Piccoli, J.; Coelho, A.; Bruno, J.B.; Faoro, D.; Manfredini, V. 14 Days of Supplementation with Blueberry Extract Shows Anti-Atherogenic Properties and Improves Oxidative Parameters in Hypercholesterolemic Rats Model. International Journal of Food Sciences and Nutrition 2015, DOI:10.3109/09637486.2015.1064870
- Sazuka, Y.; Tanizawa, H.; Takino, Y. Effect of Adriamycin on the Activities of Superoxide Dismutase, Glutathione Peroxidase and Catalase in Tissues of Mice. Japanese Journal of Cancer Research 1989, 80, 89–94.
- Wang, Y.K.; Hong, Y.J.; Wei, M.; Wu, Y.; Huang, Z.Q.; Chen, R.Z.; Chen, H.Z. Curculigoside Attenuates Human Umbilical Vein Endothelial Cell Injury Induced by H2O2. Journal of Ethnopharmacology 2010, 132, 233–239.
- Bernillon, S.; Guyot, S.; Renard, C.M. Detection of Phenolic Oxidation Products in Cider Apple Juice by High-Performance Liquid Chromatography Electrospray Ionisation Ion Trap Mass Spectrometry. Rapid Communications in Mass Spectrometry 2004, 18, 939–943.
- Jin, S.W.; Chen, G.L.; Du, J.J.M.; Wang, L.H.; Ren, X.; An, T.Y. Antioxidant Properties and Principal Phenolic Compositions of Cynomorium Songaricum Rupr. International Journal of Food Properties 2014, 17, 13–25.
- Gu, L.; Kelm, M.A.; Hammerstone, J.F.; Beecher, G.; Holden, J.; Haytowitz, D.; Prior, R.L. Screening of Foods Containing Proanthocyanidins and Their Structural Characterization Using LCMS/MS and Thiolytic Degradation. Journal of Agricultural and Food Chemistry 2003, 51, 7513–7521.
- Sun, B.S.; Leandro, M.C.; de Freitas, V.; Spranger, M.I. Fractionation of Red Wine Polyphenols by Solid-Phase Extraction and Liquid Chromatography. Journal of Chromatography A 2006, 1128, 27–38.
- Yoshimura, M.; Ninomiya, K.; Tagashira, Y.; Maejima, K.; Yoshida, T.; Amakura, Y. Polyphenolic Constituents of the Pericarp of Mangosteen (Garcinia Mangostana L.). Journal of Agricultural and Food Chemistry 2015, 63, 7670–7674.
- Xiao, J.S.; Xie, B.J.; Cao, Y.P.; Wu, H.; Sun, Z.D.; Xiao, D. Characterization of Oligomeric Procyanidins and Identification of Quercetin Glucuronide from Lotus (Nelumbo Nucifera Gaertn.) Seedpod. Journal of Agricultural and Food Chemistry 2012, 60, 2825−2829.
- Wu, Q.; Chen, H.Y.; Lv, Z.J.; Li, S.Y.; Hu, B.; Guan, Y.F.; Xie, B.J.; Sun, Z.D. Oligomeric Procyanidins of Lotus Seedpod Inhibits the Formation of Advanced Glycation End-Products by Scavenging Reactive Carbonyls. Food Chemistry 2013, 138, 1493–1502.
- Lin, Y.S.; Wu, S.S.; Lin, J.K. Determination of Tea Polyphenols and Caffeine in Tea Flowers (Camellia Sinensis) and Their Hydroxyl Radical Scavenging and Nitric Oxide Suppressing Effects. Journal of Agricultural and Food Chemistry 2003, 51, 975–980.
- Ana, F.; Concei, C.; Victor, D.F.; Nuno, M. Procyanidins as Antioxidants and Tumor Cell Growth Modulators. Journal of Agricultural and Food Chemistry 2006, 54, 2392–2397.
- Luzak, B.; Kosiorek, A.; Syska, K.; Rozalski, M.; Bijak, M.; Podsedek, A.; Balcerczak, E.; Watala, C.; Golanski, J. Does Grape Seed Extract Potentiate the Inhibition of Platelet Reactivity in the Presence of Endothelial Cells? Advances in Medical Sciences 2014, 59, 178–182.
- Agarwal, C.; Singh, R.P.; Dhanalakshmi, S.; Agarwal, R. Anti-Angiogenic Efficacy of Grape Seed Extract in Endothelial Cells. Oncology Reports 2004, 11, 681–685.
- Kim, Y.; Choi, Y.; Ham, H.H.; Jeong, S.; Lee, J. Protective Effects of Oligomeric and Polymeric Procyanidin Fractions from Defatted Grape Seeds on Tert-Butyl Hydroperoxide-Induced Oxidative Damage in HepG2 Cells. Food Chemistry 2013, 137, 136–141.
- Yapislar, H.; Taskin, E. L-Carnosine Alters Some Hemorheologic and Lipid Peroxidation Parameters in Nephrectomized Rats. Medical Science Monitor 2014, 20, 399–405.
- Wei, M.X.; Wu, Y.M.; Chen, D.Z.; Gu, Y.C. Changes of Free Radicals and Digestive Enzymes in Saliva in Cases with Deficiency in Spleen-Yin Syndrome. Journal of Biomedical Research 2010, 3, 250–255.
- Fang, J.; Seki, T.; Maeda, H. Therapeutic Strategies by Modulating Oxygen Stress in Cancer and Inflammation. Advanced Drug Delivery Reviews 2009, 61, 290–302.
- Matés, J.M.; Pérez-Gómez, C.; Castro, I.N.D. Antioxidant Enzymes and Human Diseases. Clinical Biochemistry 1999, 32, 595–603.
- Xu, J.; Rong, S.; Xie, B.; Sun, Z.; Zhang, L.; Wu, H.; Yao, P.; Zhang, X.; Zhang, Y.; Liu, L. Rejuvenation of Antioxidant and Cholinergic Systems Contributes to the Effect of Procyanidins Extracted from the Lotus Seedpod Ameliorating Memory Impairment in Cognitively Impaired Aged Rats. European Neuropsychopharmacology 2009, 19, 851–860.
- Huang, L.L.; Pan, C.; Wang, L.; Ding, L.; Guo, K.; Wang, H.Z.; Xu, A.M.; Gao, S. Protective Effects of Grape Seed Proanthocyanidins on Cardiovascular Remodeling in DOCA-Salt Hypertension Rats. The Journal of Nutritional Biochemistry 2015, 26, 841–849.
- Sonmez, M.F.; Tascioglu, S. Protective Effects of Grape Seed Extract on Cadmium-Induced Testicular Damage, Apoptosis, and Endothelial Nitric Oxide Synthases Expression in Rats. Toxicology and Industrial Health 2015, 86, 1–9.
- Aldini, G.; Carini, M.; Piccoli, A.; Rossoni, G.; Facino, R.M. Procyanidins from Grape Seeds Protect Endothelial Cells from Peroxynitrite Damage and Enhance Endothelium-Dependent Relaxation in Human Artery: New Evidences from Cardio-Protection. Life Science 2003, 73, 2883–2898.
- Berti, F.; Manfredi, B.; Mantegazza, P.; Rossoni, G. Procyanidins from Vitis Vinifera Seeds Display Cardioprotection in An Experimental Model of Ischemia-Reperfusion Damage. Drugs Under Experimental and Clinical Research 2003, 29, 207–216.
- Corder, R.; Mullen, W.; Khan, N.Q.; Marks, S.C. Red Wine Procyanidins and Vascular Health. Nature 2006, 444, 566–570.