ABSTRACT
Pomegranate is a medicinal and nutritional fruit that has several bioactivities including antimicrobial, antiviral, antioxidant, anti-inflammatory, antidiabetic, and anticarcinogenic activities. Pomegranate seed ethanol extract incorporated in cellulose dispersions and cellulose films using mild operating conditions. Carboxymethylcellulose showed the highest pH, conductivity, zeta-potential and particle size. Hydroxyethylcellulose had the highest viscosity and the lowest particle size. Hydroxypropylmethylcellulose revealed the lowest conductivity and viscosity. Methylcellulose contained the lowest pH and zeta-potential. Pomegranate seed ethanol extract caused a decrease in pH and conductivity and an increase in zeta potential, particle size and antioxidant power of the dispersions. The lowest tensile strength, elastic modulus, and swelling belonged to hydroxyethylcellulose film. Hydroxypropylmethylcellulose film showed the highest tensile strength, elastic modulus and swelling and the lowest elongation at break and water transmissions. Methylcellulose film contained the highest elongation at break and water transmission. Pomegranate seed ethanol extract caused a decrease in tensile strength, elastic modulus and swelling, and an increase elongation at break and water transmission of the film. Scanning electron microscope analyses indicated that pomegranate seed ethanol extract had an impact on the film morphology. Pomegranate seed ethanol extract caused a coarse film microstructure due to the appearance of pomegranate seed ethanol extract aggregates which produce discontinuities in film matrix.
Introduction
Experimenting with alternative, sustainable options for edible packaging is somewhat of a newly developed concept that holds a lot of merit. Polysaccharide polymers can be used as packaging films and offer an ultra-practical option for R&D (research and development). Production of packaging films based on polysaccharides with strong antioxidant and antibacterial capability would be a strategic choice in health global mega trends.[1,Citation2] Among these biopolymers are carboxymethylcellulose (CMC), hydroxyethylcellulose (HEC), hydroxypropylmethylcellulose (HPMC), and methylcellulose (MC) which are naturally occurring polymer obtained from cellulose. They are attractive and renewable natural materials characterized by favorable properties such as remarkable mechanical property, porosity, water absorbency, and excellent biological activity.[Citation3,Citation4] They have also good biocompatibility and biodegradability and have a variety of applications in the food[Citation5,Citation6] and biomedical[Citation7,Citation8] fields. However, cellulose exhibit very low antioxidant capacity and antimicrobial activity, which limits its application as a bio-active film. Therefore, chemical and synthetic antioxidants and antimicrobial agents have been employed in films to make them antioxidative and/or antimicrobials. However, there is growing interest in using plant extracts as natural sources of antioxidant/antibacterial compounds in the formulation of films.[Citation9]
Pomegranate is a medicinal and nutritional food product because of its multi-functionality and its great benefit in the human diet. The pomegranate fruit has been considered a beneficial food which has valuable compounds in different parts including peel, aril, and seed.[Citation10] Pomegranate has several medicinal effects, including anti-microbial,[Citation11] anti-viral,[Citation12] anti-oxidant,[Citation13] anti-inflammatory,[Citation14] anti-diabetic,[Citation15] anti-tumoral,[Citation16] and anti-hepatotoxic[Citation17] effects. Pomegranate also can improve oral health[Citation18] and skin health,[Citation19] that made it suitable for preparation of edible films. The health benefits of pomegranate have been attributed to its wide range of phytochemicals, which are predominantly polyphenols that are useful in the treatment and prevention of pathologic conditions.[Citation20] To the best of the author’s knowledge, there are no studies performed on the rheological property and antioxidant activity of pomegranate seed ethanol extract (POM) encapsulated in CMC, HEC, HPMC, and MC dispersions and films. The present study was devoted to analyzing CMC, HEC, HPMC, and MC dispersions and films prepared and incorporated with POM.
Materials and methods
Chemical materials
CMC (90 kDa), HEC (250 kDa), HPMC (40 kDa), MC (86 kDa) 1,1-diphenyl-2-picryl-hudrazyl radical (DPPH), and 2,2-azino-bis (3-ethylbenzthiazoline-6-sulfonic acid) radical (ABTS) were purchased from Sigma-Aldrich chemical Co. (Saito Louis, Mo, USA).
Plant materials
Hydro-ethanolic extract of POM was prepared by dissolving 100 g of powdered seed in 1000 mL aqueous-ethanolic solution (50% v/v). The mixture was incubated in a shaker incubator at 40°C with continuous shaking for 48 h. The mixture was held in room temperature to settle down large particles. Supernatant harvested and filtered by filter paper. The filtrate dried by speed vacuum concentrator and the final powder was collected. The dried mass (100 mg/mL) was emulsified in 1% w/v of tween 20 and stored at 4°C until the next use. This stock solution was used for preparation of dispersions. Tween/POM was a Newtonian fluid with negative zeta-potential (–2.4 mV) and 185 nm average particle size diameter.
Preparation of the dispersions
To prepare CMC, HEC, HPMC, and MC dispersions, one gram of the biopolymer was dissolved in 80 mL of distilled water under continuous stirring at 70°C until a homogenous solution was obtained. The homogenous dispersions were sonicated using an ultrasonic system (Bandlin, Germany) at 140 Watt for 5 min at 30°C. Different concentrations of POM (50 and 100 mg/g of polymer powder) were added to dispersions and mixed under mechanical stirring at 800 RPM for 12 h at 40°C. The dispersions named as follows; CMC, CMC/POM50, CMC/POM100, HEC, HEC/POM50, HEC/POM100, HPMC, HPMC/POM50, HPMC/POM100, MC, MC/POM50, MC/POM100. As an emulsifier, glycerol (400 mg/g of polymer) was added to all dispersions and stirred at 800 RPM in 40°C. At the end, distilled water was added until the final volume was reached to 100 mL under mechanical stirring at 800 RPM at 40°C. The final dispersions were stored at 4°C until further experiments. Before physical tests, the dispersions were equilibrated at room temperature (25–27°C) for 3 h to reach a constant temperature. The dispersions were stable for at least 150 days as we checked by visual observation through testing phase separation.[Citation21]
Conductivity and pH of the dispersions
Mettler-Toledo instrument (Cleantech, Schaffhausen, Switzerland) was used to measure the conductivity and pH of the dispersions.
Zeta-potential of the dispersions
The dispersions were diluted to a final concentration of 50 µg/mL by deionized water. The electrophoretic mobility of particles in the dispersions was determined by phase analysis light scattering (PALS) technique using a Brookhaven instruments corporation 90 Plus zeta-sizer (New York, 11742, USA). The Bi-PALS zeta-potential analyzer software provided an average of electrophoretic mobility and a measure of zeta-potential using smolouchewsky model.[Citation22]
Particle size of the dispersions
The dispersions were diluted to a final concentration of 50 µg/mL by deionized water. The effective hydrodynamic diameter of particles was determined based on the principle of dynamic light scattering (DLS) by using Brookhaven instrument corporation 90 Plus particle size analyzer. The Bi-9000 particle sizing software provided an average effective hydrodynamic diameter.[Citation21]
Apparent viscosity of the dispersions
The dispersions were decanted into a 200 mL glass cylinder (80 mm × 70 mm) and left on an anti-vibration bench with a flat level for 10 min for equilibration. The apparent viscosities (mPa. s) of the dispersions were quantified through the use of Brookfield Viscometer (DV-II + PRO digital Viscometer, Meddleboro, USA, spindle 2) at spindle rotational speed of 1, 2, 5, and 10 s−1. The software of Brookfield instrument directly provided the apparent viscosity of the dispersions.[Citation22]
Light absorbance of the dispersions
The UV-Visible absorbance spectrums of the dispersions were measured over a wavelength range of 200 to 700 nm by employing Bio Tek microplate readers (Tokyo, Japan).
Preparation of films
The filmogenic dispersions were poured into flat and leveled polystyrene petri dishes (Farazbin, Tehran, Iran) for film casting. Once set, the petri dishes were held overnight at 50 °C in a vacuum oven (Fan Azma Gostar, Tehran, Iran) to yield a uniform thickness and were then cooled to ambient temperature before peeling off the petri dishes.[Citation22] The films samples were stored in plastic bags and held in desiccators at 60% relative humidity over a saturated solution of magnesium nitrate for further testing. Thicknesses of films were measured with a micrometer (The L.S. Starrett Co. LTD. Great Britain, UK) and the average thickness were taken for mechanical properties and water vapor permeability (WVP) tests.
Mechanical properties of the films
The tensile strength, elongation at break, and elastic modulus were determined according to ASTM method D 882[Citation23] with an Instron testing machine (Santam; Iran) equipped with a tension cell of 100N. The film was cut to 60 × 10 mm. The area of film used for each experiment was 60 × 10 mm; however, 20 mm of the film fell within the jaws, so the initial length of the film was taken as 40 mm. The thickness of the film was measured at different points with a micrometer and the average taken. The initial cross-sectional area of the film cut was 10 mm × average thickness in mm. The film cut was conditioned at 27°C and 60% ± 5% relative humidity by placing it in a desiccator over a saturated solution of magnesium nitrate for 2 days. Tensile strength testing was then performed by stretching the film cut at a speed of 50 mm/min. The software of testing machine directly provided the tensile strength, elongation at break, and elastic modulus of the film. All the mechanical tests were carried out at room temperature.[Citation24]
WVP of the films
The WVP of the film samples was examined using aluminum cups filled with 20 g silica gel to produce 0% relative humidity below the film. The film cuts were conditioned at 27°C in a desiccator contained 60 ± 5% relative humidity prepared by saturated solution of magnesium nitrate for three days. After taking the initial weight of the test cups, they were placed in a box. The weight gain of the cups was measured at 3 h intervals during one day using electronic balance (Acculab, Sartorius group, Germany). The plot of weight gain (g) versus time (h) for each cup was prepared. The slope of the linear portion of this plot showed the amount of water vapor diffusion through the film per time unit and expressed as gram unit per time unit (g/h). Water vapor transmission rates (WVTR) of the film were calculated from the slope of the linear portion of this plot per square meter (m2) and expressed as g/m2.h. The WVP (g.mm/m2.kPa.h) was calculated using the following formula:
where T is the film thickness (mm) and ΔP is the partial water vapor pressure difference (kPa) between the two sides of the film (4.2449 kPa at 27°C).[Citation25]
Swelling of the films
The film was cut into a 20 mm radius disc, dried in a vacuum oven (Fan Azma Gostar, Tehran, Iran) at 70°C for 2 h, and then weighed to determine the initial dry mass. The film cut was immersed in 50 ml falcon tube containing 25 mL of distilled water. The samples were kept at room temperature for 1 h. Each sample was removed from its tube, wiped with filter paper to remove the excess surface water, and weighed to determine the final weight of the swollen film. The percentage of weight gaining was taken as swelling (S) percentage and calculated by the equation:
where Ws was the weight of the swollen film and Wd was the dry weight of the film.[Citation22]
Scanning electron microscopy (SEM) of the films
Cross-sectional analyses of cellulose films were performed with a Hitachi 570 scanning electron microscope (FESEM Hitachi S4160, Japan) according to manual instruction. To obtain the cross section of the films, the film samples were prepared by immersion in liquid nitrogen in order to avoid deformation during fracture. The film samples coated with a layer of gold allowing cross-section visualization. Cross-section pictures of films with 2 kX magnification were taken with an accelerating voltage of 5 kV.
Atomic force microscopy of the films
The surface morphology of the film samples were analyzed using Olympus atomic force microscope instrument system (California, USA) according to manual instruction. The images were acquired in the air on an anti-vibration bench and within an acoustic enclosure. Single-beam silicon cantilevers tips (PPP-NCHR-10) were used for data acquisition with about less than 10 nm nominal radius and 42 N/m elastic forces constant for high sensitivity. The resonance frequency was defined around 280 kHz. The scan rate was maintained at 0.2 Hz.
ABTS• radical scavenging assay of dispersions
Twenty microliters of different concentrations of the dispersions were added to 1 mL of diluted ABTS• radical solution (7 mM ABTS• and 2.54 mM potassium persulfate). After mixing, the light absorbance (A) was measured at 734 nm. The percentage of ABTS• scavenging was calculated via the following formula:ABTS• scavenging (%) = [(AABTS• – Atest) /AABTS•] ×100. Where, AABTS• is the absorbance of ABTS• solution and Atest is the absorbance of ABTS• solution in the presence of the dispersions. The equations obtained from the graphs plotting the ABTS• scavenging percentage versus different concentrations of the dispersions were used to calculate 50% inhibitory concentration (IC50).[Citation26]
DPPH• radical scavenging activity of dispersions
To assay the antioxidant activity by DPPH method twenty microliters of different concentrations (0-1000 µg/mL) of the dispersions were added to 1.0 mL of 0.2 mM DPPH• in 95% methanol. The mixture was blended and allowed to stand for 30 min at room temperature in darkness. The decrease in the light absorbance (A) of each sample at 517 nm was determined. DPPH• scavenging activity of dispersions was expressed as DPPH• scavenging percentage by the following formula:
where, ADPPH is absorbance of DPPH• solution and Atest is absorbance of DPPH• solution in the presence of the dispersions. The equations obtained from the graphs plotting the DPPH• scavenging percentage versus different concentrations of the dispersions were used to calculate IC50.[Citation26]
Statistical analysis
All data are presented from at least four independent experiments and expressed as the mean ± standard deviations. The significant differences between treatments were analyzed by Duncan test at p < 0.05 by using statistical package for the social sciences (SPSS, Abaus Concepts, Berkeley, CA) software version 16. The graphs and related equations were obtained using Microsoft Excel software version 2010.
Results and discussion
ph of the dispersions
MC dispersion showed the lowest pH value (4.66) followed by HPMC (6.43), HEC (6.51) and then CMC (7.07; ). POM significantly decreased (p < 0.05) the pH of CMC, HEC, HPMC and MC. These results are consistent with the results of Sanchez-Gonzalez et al.[Citation21] who used the oil of tea tree oil in HPMC emulsion and the results of Sanchez-Gonzalez et al.[Citation22] on HPMC emulsion incorporated with bergamot oil. CMC and MC/POM100 had highest and lowest pH values, respectively (). The decrease in the pH levels is most probably due to the acidic nature of some compounds in POM. Pomegranate seeds contain high levels of unsaturated fatty acids such as linoleic, linolenic, punicic, oleic, stearic and palmitic acids, as well as polyphenols[Citation10] which may lead to a slight decrease in the pH of solutions.
Table 1. pH, conductivity (µS/cm), Zeta-potentioal (mV), and particle size (nm) of carboxymethylcellulose (CMC), hydroxyethylcellulose (HEC), hydroxypropylmethylcellulose (HPMC), and methylcellulose (MC) dispersions incorporated with ethanol extract of pomegranate seed (POM).
Conductivity of the dispersions
CMC dispersions showed the highest conductivity (4140 µS/cm), followed by HEC (2035 µS/cm), HPMC (962 µS/cm) and MC (875 µS/cm; ). The high conductivity of CMC may be related to the negative charges of carboxyl groups in the CMC chain. POM caused a significant (p < 0.05) decrease in the conductivity of all dispersions. Our data reveal that CMC had the highest conductivity while MC/POM100 had the lowest one. The conductivity of dispersions was associated to the number of charged particles in the dispersion and electrical conductivity and/or electrical insulation of all additives incorporated in dispersions. Negatively charged carboxyl groups may be related to conductivity of these polymers. Since POM basically has hydrophobic nature, it is less soluble in water and the effects of POM on the conductivity of the dispersion could be the related to its hydrophobicity.[Citation27]
Zeta-potential of the dispersions
The zeta-potential values of CMC (–2.7 mV), HPMC (–1.7 mV), HEC (–1.6 mV), and MC (–1.5 mV) were negative (). POM leads a significant (p < 0.05) increase in the zeta-potential of CMC, HEC, HPMC and MC dispersions. Our laboratory data has revealed that CMC/POM100 had the highest zeta potential and MC had the lowest one. Previous study by Sanchez-Gonzalez et al.[Citation22] has reported that bergamot oil increased electrophoretic mobility and zeta-potential values of HPMC emulsion, while lemon oil had no significant effects on the zeta-potential of HPMC emulsion. The increase in negative charges on the surface of cellulose dispersion by the addition of POM can be linked to the interaction between cellulose molecules and pomegranate extract. Interaction between POM (slight negative charge) and cellulose (negative charges) increases net negative charge on the surface of filmogenic particles. It means that POM that emulsified in Tween 20 makes a droplet-like structure. Cellulose chain (with negative charges) is arranged at surrounding of these droplets, thus zeta-potential tend to be increased.[Citation28]
Particle size of the dispersions
CMC showed the higher particle size (217 nm) than HPMC (138 nm), MC (132 nm) and HEC (86 nm). POM leads a significant (p < 0.05) increase in the particle size of the all dispersions. The highest and lowest particle sizes belonged to HPMC/POM100 and HEC, respectively (). The addition of POM to cellulose dispersions caused an increase in mean particle size, which forms greater particles contained higher negative charge. An increase in the particle size of HPMC with increasing essential oil (bergamot oil, lemon oil, basil oil and thyme oil) content had also been reported by others.[Citation21,Citation22] The increase in the particle size may be related to an interfacial interaction between of POM and cellulose chain. These interactions along with low electrostatic repulsion between polymer particles and POM increase aggregation in the emulsified system which leads to increase in particle sizes.[Citation29] It can be suggested that POM emulsified in tween 20 make a droplet-like structure surrounded by cellulose and increase the particle sizes.[Citation30]
Apparent viscosity of the dispersions
The viscosity of the cellulose dispersion as a function of POM and in the specified shear rate range is summarized in . HEC showed higher viscosity (282 mPa.s) than MC (125 mPa.s), CMC (114 mPa.s), and HPMC (34 mPa.s). Incorporation of POM into the CMC, HPMC, and MC dispersion had no significant effect on the viscosity of them, while increased the viscosity of HEC dispersion. The highest and lowest viscosity belonged to HEC and HPMC dispersions, respectively. Sanchez-Gonzalez et al.[Citation22] reported that addition of bergamot and lemon oil content decreased the viscosity of chitosan dispersion. The viscosity of the dispersions evaluated in the present study slightly increased through the increase in the rotational speed of the spindle in the analyzed speed ranges, except for HEC, HEC/POMSE50, and HEC/POMSE100 which are decreased. Thus, CMC, HPMC, and MC dispersions evaluated here were non-Newtonian shear-thickening fluids, while HEC was non-Newtonian shear-thinning fluid. The physical properties of the dispersed particle, including the average particle size and zeta-potential will influence the rheological properties of dispersions. When the particle size is decreased, the number of particles increases, hence the number of particle-particle interactions increases, which causes an increase in viscosity of the sample.[Citation31] For a shear thickening fluid, on the increase in the shear rate the particle start to physically collide with each other so the particle-particle interactions increased, causes a shear thickening effect. Accordingly, the authors suggest a high interaction force between CMC, MC, and HPMC particles at high shear rate; however, more specialized studies is necessary. The increase in the shear rate also increased the physical interactions among the adjacent polymer chains and also a significant increase in viscosity in tested shear rate range.[Citation32] For a shear thinning fluid, the particle-particle interaction occurred with smaller forces, so this interaction can be broken down by an increase in the shear rate. The decrease in the viscosity values of HEC, HEC/POMSE50, and HEC/POMSE100 dispersions at higher shear rate may be explained by the fact that the orientation of polymer chains and spindle rotation at higher share rate turn out to be same. It leads to physical interactions among the adjacent polymer chains and so the viscosity decreases.[Citation33]
Table 2. Viscosity (mPa.s) of carboxymethylcellulose (CMC), hydroxyethylcellulose (HEC), hydroxypropylmethylcellulose (HPMC), and methylcellulose (MC) dispersions incorporated with ethanol extract of pomegranate seed (POM).
Light absorbance of the dispersions
CMC, HEC, HPMC, and MC dispersions had no light absorbance at tested range, 200 to 500 nm. POM caused a significant (p < 0.05) increase in the light absorbance of the dispersions with maximum light absorbance at 300 nm. It has been declared that the light absorbance of POM is related to phenolic and flavonoids compounds, principally anthocyanin.[Citation10] POM droplets that were localized in the matrix increased the light absorbance of the dispersions. Therefore, the resulting dispersions contain light barrier properties, which can be interesting in certain applications for preventing UV-induced lipid peroxidation.
Mechanical properties of the films
Mechanical properties of cellulose films, including tensile strength, elastic modulus and elongation at break are summarized in . HPMC film showed higher tensile strength (56 MPa) than MC (34 MPa), CMC (25 MPa), and HEC (2.4 MPa). HPMC film had the highest elastic modulus (765 MPa) than MC (537 MPa), CMC (470 MPa), and HEC (48 MPa). Elongation at break was high in MC (70%) while HEC (34%), CMC (27%), and HPMC (8%) had considerably lower elongation at break. POM caused a significant (p < 0.05) decrease in tensile strength and elastic modulus and a significant increase in elongation at break of the cellulose films (). Thus, incorporation of POM to the film structure, it became less stiff and more flexible. A decrease in the tensile strength and increase in the flexibility of different cellulose films with increasing plant extract content has been reported formerly by various authors.[Citation21,Citation22,Citation34,Citation35]
Table 3. Tensile strength (MPa), elastic modulus (MPa), elongation at break (%), water vapor permeability (mg.mm/kPa.m2.h) and swelling capacity (%) of carboxymethylcellulose (CMC), hydroxyethylcellulose (HEC), hydroxypropylmethylcellulose (HPMC), and methylcellulose (MC) films incorporated with ethanol extract of pomegranate seed.
Cellulose films were mainly stabilized by the weak bonds including hydrogen bond between hydroxyl groups.[Citation36] The reducing tensile strength of the films by the addition of POM is likely related to the formation of intermolecular interaction between hydroxyl groups of cellulose and POM. Thus, the original hydrogen bonds between cellulose chains that stabilized film matrix may be replaced with new hydrogen bonds between cellulose molecules and POM; therefore, it decreases tensile strength.[Citation24,Citation34] By the incorporation of POM, the film structure becomes less stiff and more flexible. This phenomenon could be related to replacement of original hydrogen bonds between cellulose chains with new hydrogen bonds between cellulose and POM, which caused an increase in the segmental mobility of cellulose chains.[Citation37,Citation38] The increasing flexibility of the film samples by the addition of POM, as described above, could be related to the formation of new hydrogen bonds between POM and cellulose chain which resulted in a loss compact structure of cellulose matrix and more sliding effect of cellulose chains against each other.[Citation39,Citation40]
WVP of the films
The results of WVP and swelling capacity of CMC, HEC, HPMC and MC dispersions are summarized in . MC film showed higher WVP (718 mg.mm/m2.kPa.h) than HEC (593 mg.mm/m2.kPa.h), CMC (423 mg.mm/m2.kPa.h), and HPMC (267 mg.mm/m2.kPa.h). HPMC film showed higher swelling (480%) than CMC (337%), MC (293%), and HEC (273%). POM caused a significant increase in the WVP and decrease in the swelling of the films. Since cellulose contains large amount of hydroxyl groups this is a highly hydrophilic material which has ability to absorb large amount of water molecules which may lead to lower water transmission. Incorporation of POM into the films caused a significant increase in their WVP. It might be ascribed by the establishment of polymer-POM interactions, which weaken the interactions that stabilize the polymer network. POM interacts with hydroxyl groups of the polymer chain and competitively weakens chain-to-chain hydrogen binding in polymer structure. Moreover, the original hydrogen binding between polymer chains replaced with the new hydrogen bonds between hydroxyl groups of polymer and POM. This event increases the distance between polymer chain and introduced twisting pore in the polymer matrix. It results to increased water vapor transmission across the film samples.[Citation25] Cellulose is hydrophilic because of the hydroxyl groups and can absorb large amounts of water molecules. POM could reduce the swelling capacity of the film. The hydroxyl groups of cellulose can interact with POM through hydrogen bonding, increasing the interaction between cellulose and POM. This event saturates the cellulose network with POM, preventing the water molecules from interacting with cellulose and decreasing swelling.[Citation39]
SEM
Cross-section SEM images of CMC, HEC, HPMS and MC film are presented in . CMC film presented a smooth structure with several small crake in the film matrix. Incorporation of POM caused several pore in the film matrix and an increase in the number and size of crake in the film matrix. HEC film presented a smooth and compact structure without any crake in the film matrix. Incorporation of POM introduced several pore and large crake in the film matrix. HPMC had a compact and wavy structure. HPMC/POM had several pores in the film matrix without any crack. Ultrastructure of MC film presented a heterogeneous and compact structure. Addition of POM caused a large number of pores and small cracks in the MC film matrix. Ethanolic extract of POM has low solubility in the cellulose solution, which can form a crystal like structure during the film formation.[41] The presence of POM into the cellulose film can cause a coarse film structure by introducing pore and crake which caused the formation of a tangled polymer chain in the cellulose matrix. Overally, POM modifies cellulose film ultrastructure which leads to decrease in the tensile strength as well as increase in water transmission.[Citation42]
Atomic force microscopy
Atomic force microscope images of cellulose films are shown in . CMC films showed smooth and homogeneous surface while POM induced changes in the surface topography of CMC films by increasing surface roughness. HEC films had an uneven and irregular surface. The addition of POM increased surface smoothness. HPMC films revealed a rough and crooked surface. HPMC films contained POM had higher surface roughness. MC films showed a rough and crooked surface which was smoother when POM was added. Surface topology of cellulose films strongly depends on the cellulose derivative and POM incorporation. It is well known that the surface topology of polymeric films conducted the polymer chain association by hydrogen binding or van der Waals interactions. The homogenous surface structure describes the extensive association between polymer chains with each other or polymer chains with additives; while the heterogeneous surface is an indicator of low hydrogen binding and low film-forming capacity.[41] POM induced changes in the surface topography of cellulose films. This means that high concentration of POM decreased cellulose chain association in the dispersion and during the film drying and casting.[Citation43] Accordingly, POM had an impact on the surface morphology and structure of cellulose film. The change in the film morphology will affect the mechanical and water barrier properties of the films.
Figure 2. Atomic force microscopy images of carboxymethylcellulose (CMC), hydroxyethylcellulose (HEC), hydroxypropylmethylcellulose (HPMC), and methylcellulose (MC) film incorporated with ethanol extract of pomegranate seed. Cellulose films showed smooth, homogeneous or irregular surface while POM induced changes in the surface topography of the films by increasing or decreasing surface roughness.
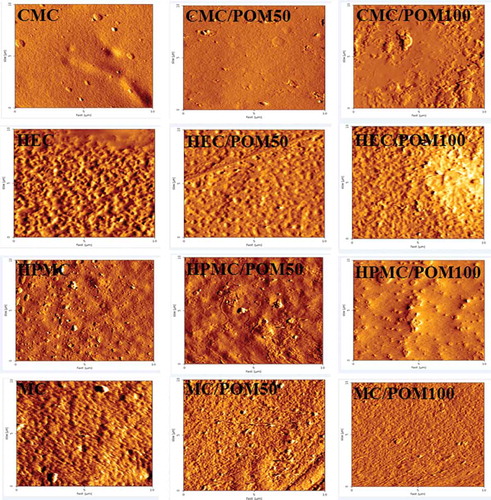
Antioxidant activity of the dispersion
We further examined the antioxidant activities of cellulose dispersions against ABTS•, DPPH• radicals (). Pure CMC, HEC, HPMC and MC dispersions showed a very low antioxidant activity against ABTS• and DPPH• at concentrations used.[Citation44] CMC, HEC, HPMC, and MC, which were mixed with POM, had potent antioxidant activity. Several studies have already reported similar DPPH• and ABTS• scavenging capacities for POM.[Citation13,Citation17] DPPH• and ABTS• radical scavenging capacity of dispersions are strong indicators of hydrogen atom and/or the electron donating capacity of POM. In the presence of POM, a hydrogen atom or an electron is transferred to DPPH• and ABTS• radicals, converting them to a non-radical state.[Citation26] It has been reported that the antioxidant activity of POM is related to the diverse phenolic compounds present in it.[Citation13] These compounds are known for their properties to scavenge free radicals or chelate metal ions.[Citation45] It has also been reported that oxidants may play important roles in the development of many chronic diseases and may result in numerous pathological conditions via oxidative damage to lipid, unsaturated fatty acids, protein, and nucleic acids.[Citation46] The antioxidant activity of the cellulose/POM dispersions imply the beneficial role of these products in reducing damages done to biological tissues or perhaps protecting tissues against oxidative damage.
Table 4. Effective concentrations (IC50) of carboxymethylcellulose (CMC), hydroxyethylcellulose (HEC), hydroxypropylmethylcellulose (HPMC), and methylcellulose (MC) dispersions incorporated with ethanol extract of pomegranate seed against ABTS and DPPH.
Conclusions
From this study it was concluded that POM is able to modify the properties of different cellulose dispersions. It decreased pH and conductivity and increased zeta potential, particle size, and antioxidant power of dispersions. POM has also decreased the tensile strength and swelling and increased the elongation at break and water vapor transmission in film samples. CMC, HEC, HPMC and MC dispersions and films exhibited different physical, mechanical and water binding capacity. Incorporation of pomegranate extract differently modified the properties on the cellulose dispersion and film.
Conflict of interest
The authors declare no conflict of interest. The authors alone are responsible for the content of this manuscript.
Funding
This work was supported by the financial support from Shiraz University (grant No. 88-GRAGRST-108).
Additional information
Funding
References
- Barreras-Urbina, C.G.; Ramírez-Wong, B.; Lopez-Ahumada, G.A.; Burruel-Ibarra, S.E.; Martinez-Cruz, O.; Tapia-Hernandez, J.A.; Rodríguez-Felix, F. Nano-and Micro-Particles by Nanoprecipitation: Possible Application in the Food and Agricultural Industries. International Journal of Food Properties 2015, DOI:10.1080/10942912.2015.1089279
- Venugopal, J.; Ramakrishna, S. Applications of Polymer Nanofibers in Biomedicine and Biotechnology. Applied Biochemistry and Biotechnology 2005, 125, 147–157.
- Shah, N.; Ul-Islam, M.; Khattak, W.A.; Park, J.K. Overview of Bacterial Cellulose Composites: A Multipurpose Advanced Material. Carbohydrate Polymers 2013, 98, 1585–1598.
- Su, J.F.; Huang, Z.; Yuan, X.Y.; Wang, X.Y.; Li, M. Structure and Properties of Carboxymethyl Cellulose/Soy Protein Isolate Blend Edible Films Cross-Linked by Maillard Reactions. Carbohydrate Polymers 2010, 79, 145–153.
- Shi, Z.; Zhang, Y.; Phillips, G.O.; Yang, G. Utilization of Bacterial Cellulose in Food. Food Hydrocolloids 2014, 35, 539–545.
- Sirvio, J.A.; Kolehmainen, A.; Liimatainen, H.; Niinimäki, J.; Hormi, O.E.O. Biocomposite Cellulose-Alginate Films: Promising Packaging Materials. Food Chemistry 2013, 151, 343–351.
- Fu, L.; Zhang, J.; Yang, G. Present Status and Applications of Bacterial Cellulose-Based Materials for Skin Tissue Repair. Carbohydrate Polymers 2013, 92, 1432–1442.
- Hu, W.; Chen, S.; Yang, J.; Li, Z.; Wang, H. Functionalized Bacterial Cellulose Derivatives and Nanocomposites. Carbohydrate Polymers 2014, 101, 1043–1060.
- Gomez-Estaca, J.; Lopez-de-Dicastillo, C.; Hernandez-Munoz, P.; Catala, R.; Gavara, R. Advances in Antioxidant Active Food Packaging. Trends in Food Science & Technology 2014, 35, 42–51.
- Viuda‐Martos, M.; Fernandez‐Lopez, J.; Perez‐Alvarez, J.A. Pomegranate and Its Many Functional Components As Related to Human Health: A Review. Comprehensive Reviews in Food Science and Food Safety 2010, 9, 635–654.
- Duman, A.D.; Ozgen, M.; Dayisoylu, K.S.; Erbil, N.; Durgac, C. Antimicrobial Activity of Six Pomegranate (Punica Granatum L.) Varieties and Their Relation to Some of Their Pomological and Phytonutrient Characteristics. Molecules 2009, 14, 1808–1817.
- Haidari, M.; Ali, M.; Casscells, S.W.; Madjid, M. Pomegranate (Punica Granatum) Purified Polyphenol Extract Inhibits Influenza Virus and Has a Synergistic Effect with Oseltamivir. Phytomedicine 2009, 16, 1127–1136.
- Cam, M.; Hısıl, Y.; Durmaz, G. Classification of Eight Pomegranate Juices Based on Antioxidant Capacity Measured by Four Methods. Food Chemistry 2009, 112, 721–726.
- Lee, C.J.; Chen, L G.; Liang, W.L.; Wang, C.C. Anti-Inflammatory Effects of Punica Granatum Linne in Vitro and in Vivo. Food Chemistry 2010, 118, 315–322.
- Xu, K.Z.Y.; Zhu, C.; Kim, M.S.; Yamahara, J.; Li, Y. Pomegranate Flower Ameliorates Fatty Liver in An Animal Model of Type 2 Diabetes and Obesity. Journal of Ethnopharmacology 2009, 123, 280–287.
- Hamad, A.W.; Al-Momene, W. Separation and Purification of Crude Ellagic Acid from White Flesh of Pomegranate Fruits As a Potent Anti-Carcinogenic. New Biotechnology 2009, 25, S286.
- Celik, I.; Temur, A.; Isik, I. Hepatoprotective Role and Antioxidant Capacity of Pomegranate (Punica Granatum) Flowers Infusion Against Trichloroacetic Acid-Exposed in Rats. Food and Chemical Toxicology 2009, 47, 145–149.
- Di Silvestro, R.A.; Di Silvestro, D.J.; DiSilvestro, D.J. Pomegranate Extract Mouth Rinsing Effects on Saliva Measures Relevant to Gingivitis Risk. Phytotherapy Research 2009, 23, 1123–1127.
- Aslam, M.N.; Lansky, E.P.; Varani, J. Pomegranate As a Cosmeceutical Source: Pomegranate Fractions Promote Proliferation and Procollagen Synthesis and Inhibit Matrix Metalloproteinase-1 Production in Human Skin Cells. Journal of Ethnopharmacology 2006, 103, 311–318.
- Miguel, M.G.; Neves, M.A.; Antunes, M.D. Pomegranate (Punica Granatum L.): A Medicinal Plant with Myriad Biological Properties—A Short Review. Journal of Medicinal Plants Research 2010, 4, 2836–2847.
- Sanchez-Gonzalez, L.; Vargas, M.; Gonzalez-Martinez, C.; Chiralt, A.; Chafer, M. Characterization of Edible Films Based on Hydroxypropylmethyl Cellulose and Tea Tree Essential Oil. Food Hydrocolloids 2009, 23, 2102–2109.
- Sanchez-Gonzalez, L.; Chiralt, A.; Gonzalez-Martinez, C.; Chafer, M. Effect of Essential Oils on Properties of Film Forming Emulsions and Films Based on Hydroxypropylmethylcellulose and Chitosan. Journal of Food Engineering 2011, 105, 246–253.
- ASTM. Standard Test Method for Tensile Properties of Thin Plastic Sheeting: D882-02 Annual Book of American Society for Testing Materials; West Conshohocken, PA, 2002.
- Chen, G.; Zhang, B.; Zhao, J.; Chen H. Development and Characterization of Food Packaging Film from Cellulose Sulfate. Food Hydrocolloids 2014, 35, 476–483.
- Ramirez, C.; Gallegos, I.; Ihl, M.; Bifani, V. Study of Contact Angle, Wettability and Water Vapor Permeability in Carboxymethylcellulose Based Film with Murta Leaves (Ugni Molinae Turcz) Extract. Journal of Food Engineering 2012, 109, 424–429.
- Boligon, A.A. Technical Evaluation of Antioxidant Activity. Medicinal Chemistry 2014, 4, 517–522.
- Sovilj, V.J.; Petrovic, L.B. Influence of Hydroxypropylmethyl Cellulose–Sodium Dodecylsulfate Interaction on the Solution Conductivity and Viscosity and Emulsion Stability. Carbohydrate Polymers 2006, 64, 41–49.
- Bhardwaj, N.K.; Hoang, V.; Nguyen, K.L. A Comparative Study of the Effect of Refining on Physical and Electrokinetic Properties of Various Cellulosic Fibers. Bioresource Technology 2007, 98, 1647–1654.
- Huang, X.; Kakuda, Y.; Cui, W.; Hydrocolloids in Emulsions: Particle Size Distribution and Interfacial Activity. Food Hydrocolloids 2001, 15, 533–542.
- Sanghvi, S.P., Nairn, J.G. A Method to Control Particle Size of Cellulose Acetate Trimellitate Microspheres. Journal of Microencapsulation 1993, 10, 181–194.
- McClements, D.J. Critical Review of Techniques and Methodologies for Characterization of Emulsion Stability. Critical Review in Food Science and Nutrition 2007, 47, 611–649.
- Clasen, C.; Kulicke, W.M. Determination of Viscoelastic and Rheo-Optical Material Functions of Water-Soluble Cellulose Derivatives. Progress in Polymer Science 2001, 26, 1839–1919.
- Liu, L.; Zhao, Q.; Liu, T.; Kong, J.; Long, Z.; Zhao, M. Sodium Caseinate/Carboxymethylcellulose Interactions at Oil–Water Interface: Relationship to Emulsion Stability. Food Chemistry 2012a, 132, 1822–1829.
- Liu, Q.; Han, J.C.; Zhang, Y.G.; Li, S.M.; Li, J. Antimicrobial and Antioxidant Activities of Carboxymethyl Cellulose Edible Films Incorporated with Rosemary Extracts on Fresh Beef During Refrigerated Storage. Advanced Materials Research 2012b, 554, 1187–1194.
- Pastor, C.; Sanchez-Gonzalez, L.; Chiralt, A.; Chafer, M.; Gonzalez-Martinez, C. Physical and Antioxidant Properties of Chitosan and Methylcellulose Based Films Containing Resveratrol. Food Hydrocolloids 2013, 30, 272–280.
- Wang, L.Z.; Liu, L.; Holmes, J.; Kerry, J.F.; Kerry, J.P. Assessment of Film-Forming Potential and Properties of Protein and Polysaccharide-Based Biopolymer Films. International Journal of Food Science and Technology 2007, 42, 1128–1138.
- De Moraes, J.O.; Muller, C.M.O.; Laurindo, J.B. Influence of the Simultaneous Addition of Bentonite and Cellulose Fibers on the Mechanical and Barrier Properties of Starch Composite-Films. Food Science Technology International 2011, 18, 35–45.
- Huang, H.; He, P.; Hu, N.; Zeng, Y. Electrochemical and Electrocatalytic Properties of Myoglobin and Hemoglobin Incorporated in Carboxymethyl Cellulose Films. Bioelectrochemistry 2003, 61, 29–38.
- Imran, M.; El-Fahmy, S.; Revol-Junelles, A.M.; Desobry, S. Cellulose Derivative Based Active Coatings: Effects of Nisin and Plasticizer On Physico-Chemical and Antimicrobial Properties of Hydroxypropyl Methylcellulose Films. Carbohydrate Polymer 2010, 81, 219–225.
- Saha, S.; Tomaro-Duchesneau, C.; Daoud, J.T.; Tabrizian, M.; Prakash, S. Novel Probiotic Dissolvable Carboxymethyl Cellulose Films As Oral Health Biotherapeutics: In Vitro Preparation and Characterization. Expert Opinion on Drug Delivery 2013, 10, 1471–1482.
- Kvien, I.; Tanem, B.S.; Oksman, K. Characterization of Cellulose Whiskers and Their Nanocomposites by Atomic Force and Electron Microscopy. Biomacromolecules 2005, 6, 3160–3165.
- Elazzouzi-Hafraoui, S.; Nishiyama, Y.; Putaux, J.L.; Heux, L.; Dubreuil, F.; Rochas, C. The Shape and Size Distribution of Crystalline Nanoparticles Prepared by Acid Hydrolysis of Native Cellulose. Biomacromolecules 2007, 9, 57–65.
- Iwamoto, S.; Kai, W.; Isogai, A.; Iwata, T. Elastic Modulus of Single Cellulose Microfibrils from Tunicate Measured by Atomic Force Microscopy. Biomacromolecules 2009, 10, 2571–2576.
- Moseley, R.; Walker, M.; Waddington, R.J.; Chen, W.Y.J. Comparison of the Antioxidant Properties of Wound Dressing Materials–Carboxymethylcellulose, Hyaluronan Benzyl Ester and Hyaluronan, Towards Polymorphonuclear Leukocyte-Derived Reactive Oxygen Species. Biomaterials 2003, 24, 1549–1557.
- Singh, R.P.; Chidambara Murthy, K.N.; Jayaprakasha, G.K. Studies on the Antioxidant Activity of Pomegranate (Punica Granatum) Peel and Seed Extracts Using in Vitro Models. Journal of Agricultural and Food Chemistry 2002, 50, 81–86.
- Ray, P.D.; Huang, B.W.; Tsuji, Y. Reactive Oxygen Species (ROS) Homeostasis and Redox Regulation in Cellular Signaling. Cellular Signalling 2012, 24, 981–990.