ABSTRACT
Phytate content, bioavailability and myo-inositol phosphates profile were analysed in pseudocereals (i.e., white and coloured quinoa, buckwheat) subjected to thermal processing and solid-state fermentation with Rhizopus oligosporus. Fair buckwheat groats were characterised by the highest level of phytate (18.3 µmol/g), with 54% share of InsP6 fraction. Processing (cooking, roasting, tempe-type fermentation) resulted in the significant decrease in phytate content, its bioavailability and the share of higher phosphorylated forms of myo-inositol (InsP6-5) in the profile of all the samples. Fermentation of cereals resulted in generation of high amounts of inositol triphosphates, particularly in the case of buckwheat (32% share in the whole profile). The path of dephosphorylation, identified after fermentation of quinoa seeds including intermediate products – Ins(1,2,4,5,6)P5 and Ins(1,2,6)P3 – confirmed that the dominant phytase activity could be attributed to the presence of microbial 3-phytases from R. oligosporous.
Introduction
Pseudocereals buckwheat (Fagopyrum esculentum, Polygonaceae) and quinoa (Chenopodium quinoa Willd., Chenopodiaceae) have recently gained strong interest as functional food components because of their nutritional characteristic: a high level of gluten-free protein (16–18%), advantageous amino acid profile with high contents of lysine and tryptophan, minerals, as well as bioactive (e.g., antioxidant) properties[Citation1,Citation2]. Buckwheat is cultivated worldwide (Europe, Asia, United States and Canada) and processed mainly to flour and roasted groats. However, fair (unroasted) groats are characterised by more favourable composition than roasted ones[Citation3]. The year 2013 was announced by FAO as the International Year of Quinoa; during last decade, the area of this crop cultivation nearly tripled in such countries as Bolivia, Paraguay and Ecuador, making these pseudocereal a real alternative for wheat, oat or rye[Citation4]. Quinoa flour was used as an additive to conventional ingredients in breadmaking due to health benefits especially in gluten-free diet or in producing noodles, pasta or sweet biscuits[Citation5–Citation7]. However, some factors such as the occurrence of saponins (quinoa), protease inhibitors and the high contents of phytates reduce the application of pseudocereals as food components.
Phytic acid (1,2,3,4,5,6-myo-inositol hexakisphosphate) and its salts are the main storage forms of phosphorus in plants. This compound is present in significant amounts in whole grain products, especially from pseudocereals[Citation8]. Phytate is hardly digested in human gastrointestinal tract and with higher phosphorylated derivatives (InsP5) form stronger complexes with minerals, which negatively influences the absorption of these elements from digestive tract[Citation9]. This is very important, especially in the case of quinoa seeds, which are characterised by a relatively high level of phytates and potentially high amounts of micro- and macrominerals. Moreover, results of some research show that the potential anticancerogenic activity of phytic acid relates in fact to the effect of its mixture with lower inositol phosphates[Citation10]. Thus, the determination of inositol phosphates profile in pseudocereals can be considered important, especially the assessment of the ratio between InsP6-5 and lower phytate forms, as well as the analysis of inositol tri- and tetraphosphates which have potential physiological function in cell signalling processes (especially Ins(1,2,3)P3 and Ins(1,4,5)P4). Phytate dephosphorylation may occur by means of thermal hydrolysis, e.g., during the cooking, or as a result of enzymatic treatments[Citation11,Citation12]. Phytases are produced by plants (endogenic enzymes active during soaking and sprouting of seeds)[Citation13], bacteria and filamentous fungi[Citation14].
Solid-state fermentation of legume seeds and cereal grains with Rhizopus oligosporus (tempe process) and Aspergillus oryzae (koji process) is commonly applied, especially in Asia, to improve the nutritional characteristics of foods[Citation15–Citation18]. As proven by our earlier research, tempe-type products of advantageous bioactive, nutritional and sensory properties may also be successfully obtained from quinoa seeds and fair buckwheat groats[Citation19,Citation20].
The purpose of the present experiment was to investigate the effect of cooking and subsequent solid-state fermentation with Rhizopus oligosporous on the content of phytates and the profile of lower inositol phosphates, including myo-inositol, in the fair buckwheat groats and quinoa seeds. Another important objective is to test the bioavailability of phytate, which can help to assess the amounts that could be accessible, as the solubility of these compound is dependent on their phosphorylation level. The initial profile of phosphates in food product can determinate their changes during the simulated digestion process. Additionally, the effect of cooking on roasted buckwheat groats was analysed, as this product is popular on the market and thus may be treated as a reference from the practical point of view.
Material and methods
Rhizopus oligosporus ATCC 64063 was grown on potato dextrose agar (PDA) at 24°C for 12 days. The spores were harvested with sterile saline solution (8 g L−1) supplemented with peptone (0.01 g L−1) and Tween 80 (0.1 mL L−1). The suspension was filtered three times through nylon net filters (mesh diameter 11 μm, Millipore, Tullagreen Carrigtwohill, Ireland) in order to remove mycelium fragments. The spore density was obtained by spore counting method in Thoma chamber. The fair and roasted buckwheat groats were obtained from a cereal processing plant Młyn Niedźwiady (Niedźwiady, Poland). The seeds of white, red and black quinoa (Bolivia origin), packaged by Bio Planet s.a., were purchased from a local health-food store in Krakow, Poland.
Cooking procedure
Fair buckwheat groats and quinoa seeds were cooked in tap water (1:3 m/v, acidified to pH 4.5–5 with lactic acid to assure proper conditions for mycelium growth) for 10 min (white quinoa, fair buckwheat groats) or 15 min, respectively. Roasted buckwheat was cooked in tap water (1:3 m/v) until it is ready for consumption (20 min), according to the procedure recommended for culinary processing of commercially available groats.
Fermentation procedure
Cooked fair buckwheat groats and quinoa seeds were dried on the surface with sterile cloth, cooled (<35°C) and mixed thoroughly with R. oligosporus inoculum (104 spores per g). Inoculated material was tightly packed in sterile Petri dishes (diameter 10 cm, six replicates for every treatment) and incubated for 40 h (2 h at 35°C to induce spores germination, followed by 31°C). After 20 h, Petri dishes were turned over in order to ensure uniform mycelium distribution. Fermented material was subjected to 10 min. steaming in order to stop fungal activity. All the obtained samples were lyophilised and stored at 3°C for further analysis. The experimental samples were as follows: fair buckwheat groats – raw, cooked and fermented, roasted buckwheat groats – raw and cooked, white and black quinoa seeds – raw, cooked and fermented. Dry matter was determined with a moisture analyser (WPS 110S, Radwag, Radom, Poland).
In vitro procedure
Bioavailability of phytate was estimated by an in vitro technique according to the method developed by Zyla et al.[Citation21] for poultry and subsequently modified as described in Starzyńska-Janiszewska et al.[Citation22] to simulate the conditions of human gastrointestinal tract. The procedure was performed in triplicates. Half a gram of sample was incubated with 1.7 mg of pepsin (Sigma-Aldrich, Steinheim, Germany; the declared activity was 4750 U/mg) dissolved in 0.1 mol/L HCI at 37°C, pH = 2.0 for 2 h. Next, 2.5 mg pancreatin (Sigma, from porcin pancreas, 8×, United States Pharmacopeia) dissolved in 0.1 mol L−1 of NaHCO3 was added, and the whole sample was incubated in dialysis tubes (Sigma-Aldrich, cellulose membrane 25 mm × 90 mm, MWCO 12000) at 37°C, pH = 7.0 for 4 h. Those incubations simulated the digestion in the stomach and small intestine, respectively. Obtained dialysates were collected for HPLC phytate determination.
Total phytate and inositol phosphates determination
Extraction of inositol phosphates from samples was conducted according to Gambuś and co-workers[Citation23]. 2 g of samples were extracted in 20 ml of 0.5 M hydrochloric acid at room temperature for 2 h. Extracts were centrifuged for 30 min at 4500 r/min and filtered. Low pressure ion exchange liquid chromatography was performed to separate myo-inositol phosphates from the extract. Chromatography columns were filled with 2 g of AG 1 × 8 anion-exchanger (200–400 mesh, chloride form) and conditioned with 10 ml of deionised water. Next, 15 ml of the extract was passed through the column, and the column was rinsed with 10 ml of deionized water. Elution of myo-inositol phosphates was performed by 20 ml of 2 M hydrochloric acid. Eluates were evaporated on a water bath preheated to 40°C and redissolved in 5 ml of deionized water, frozen at −18°C and left for HPLC analysis. The phytate analysed from these samples expressed for 1 g of dry matter was defined as ‘total phytate’.
Dialysable phytate
The samples of dialysates (35 ml) from the in vitro procedure were evaporated overnight on water bath thermostated at 40°C and redissolved in 15 mL of deionised water. Inositol phosphates were purified on AG1 × 8Cl cartridges according to Duliński et al.[Citation24]. The dialysable phytate is the amount of phytate that passed through pores in dialysis tubes into buffer during the simulated digestion experiment. The obtained results were calculated for 1 gram of the material subjected to the digestion.
Phytate bioavailability
The ‘phytate bioavailability’ was calculated as the ratio between the ‘dialysable phytate’ (phytate which passed through pores in dialysis membrane into the buffer during the in vitro digestion) and ‘total phytate’ (total amount of phytate in material, determined after acidic hydrolysis) and expressed as % (g/g).
HPLC analysis
Profiles of the isomers of myo-inositol phosphates were analysed by the analytical system using high pressure anion exchange chromatography (HPAEC) with postcolumn derivatisation and spectrophotometric detection[Citation25]. Before injection on a chromatographic column, samples were filtrated through a syringe filter (0.45 μm). A reference sample for identification of peaks was prepared by dissolving 2.3 g of sodium phytate in 50 ml of deionised water and adjusting pH to 4.0 by 2 M HCl. The solution was autoclaved for 40 min at 121°C under 1 atm. The elution sequence of different isomers was established according to the work of Blaabjerg et al.[Citation25] – by using appropriate standard solutions – sodium phytate (InsP6), Ins(1,2,4,5,6)P5, Ins(1,4,5,6)P4, Ins(1,3,4,5)P4, Ins(1,4,5)P3, Ins(1,3,4)P3, myo-inositol 2-monophosphate (all purchased from Sigma-Aldrich).
Total myo-inositol determination
Proper amounts of homogenised sample (150 mg) were subjected to acid hydrolysis with 2 ml of 1 M HCl in Duran® glass tubes (16 × 160 mm) for 48 h at 123°C. After cooling the sample, pH was adjusted to 4.8–5.0 and then subjected to HPLC assay according to Duliński et al.[Citation26].
Statistical analysis
Experimental data were subjected to the one-way analysis of variance (ANOVA) to detect significant differences among means and expressed as a mean ± standard error of mean (SEM). Differences among means were checked by the Tukey test at 0.05 using Statistica for Windows, ver. 10 (Statsoft Inc., Tulsa, OK, USA) statistical software.
Results and discussion
Processing of buckwheat
Buckwheat groats processing resulted in the decrease of phytate level in all the samples. As shown in , total phytate content was the highest in the raw material (fair groats), 18.3 µmol/g of dry matter. Cooking resulted in the statistically significant decrease of InsP6 level in the case of both cooked and roasted groats (10.4 and 8.9 µmol/g, respectively). Fermentation procedure of fair groats was more efficient in phytate decomposition than cooking alone. Value measured for tempe (7.0 µmol/g) was lower by 60% than the one of raw groats. This observation is in agreement with data obtained by other authors in studies on seeds or kernels subjected to processing by tempe-type fermentation[Citation27]. However, in our study, roasting followed by cooking was the most successful combination resulting in 68% degradation of phytate, as compared to raw fair groats (5.9 µmol/g vs 18.3 µmol/g).
Table 1. Amount of total and dialysable phytate and profile of inositol phosphates in buckwheat groats subjected to hydrothermal treatment and solid state fermentation with Rhizopus oligosporus.
The results of the analysis of inositol phosphates profile showed that inositol hexakisphosphate was the main fraction (81%) in fair buckwheat samples. Fermented buckwheat contained relatively high share of lower inositol phosphates with 3 phosphate moieties (InsP3) (30.2%), with concomitant reduction of phytate fraction to 51.7% (). When hydrothermal treatment alone was applied, the predominant fraction of lower inositol phosphates was InsP4 (28–38%), especially in the combination of roasting and cooking with only 45.7% InsP6 share in the whole profile ().
Processing of quinoa
Thermal processing of red and white quinoa seeds reduced the phytate level by 38% and 33%, respectively. Further fermentation (40 h) resulted in 72% reduction of phytate in case of white quinoa (6.4 µmol/g), as compared with the raw seeds (16.5 µmol/g). Similar tendency was observed in the coloured quinoa: 70% and 61% reduction for black and red seeds (). Egounlety and Aworth[Citation14] fermented the seeds of soybean and cowpea with Rhizopus sp. starter culture for 36h and observed a lower decrease in phytate content, by 33% and 29%, respectively. This difference may be due to various tempe preparation scheme or different distribution of InsP6 in legumes than in quinoa seeds, where compound is evenly distributed within the endosperm[Citation4].
Table 2. Amount of total and dialysable phytate and profile of inositol phosphates in white and black quinoa seeds subjected to hydrothermal treatment and solid state fermentation with Rhizopus oligosporus.
Iglesias-Puig et al.[Citation6] analysed the whole grain flour from Chenopodium used for bread making, wherein the phytate was determined at 9.28 µmol/g and InsP5 at 0.47 μmol/g. Inositol hexakisphosphate content was comparable to that obtained in our experiment in the case of cooked seeds of white quinoa (10 µmol/g), wherein InsP6 : InsP5 ratio, namely 20:1, was similar in both studies. Analysis of the profile of inositol phosphates in the raw seeds of Chenopodium confirms the presence of InsP6 as a dominant fraction (67% for white and 65% for black quinoa). The distribution of inositol fractions in quinoa seeds was characterised by a lower share of compounds with 3–4 phosphate moieties as compared with buckwheat ( and ). Fermentation resulted in a pronounced accumulation of myo-inositol pentaphosphates, especially in white quinoa samples (by 27%) with concomitant reduction of InsP2-1 (16–11% share in whole profile) as compared to the raw seeds (32–24%). A relatively low share of InsP1-2 fraction in relation to hydrothermal processing could be explained by the simultaneous activity of unspecific phosphatases and phytases. This interpretation may be supported by the fact that most microbial or plant phytases only have the capability to degrade phytate molecule to inositol monophosphate[Citation11].
Analysis of the path of the phytate degradation in Chenopodium seeds subjected to fermentation indicates the presence of intermediate products: Ins(1,2,4,5,6)P5, Ins(1,2,5,6)P4 and Ins(1,2,6)P3 as compared to the profile obtained from the raw ones ( and ). This observation demonstrates the specificity of mould enzymes from microorganisms, initially removing orthophosphate from the D-3 position of myo-inositol ring, which are considered to be 3-phytases (EC 3.1.3.8). It should be emphasised that a small amounts of myo-inositol triphosphate with configuration – 1,4,5 were also identified (). Even though the concentration of this compound was rather low, its presence is important as it is proven to exert the positive impact on human health as a potential signalling molecule[Citation28].
Figure 1. Overlayed chromatograms from the HPAEC analysis of myo-inositol phosphates from white quinoa raw and tempe seeds.
Myo-inositol phosphates in house reference standard acquired after thermal hydrolysis of phytic acid (A), white quinoa seeds – raw (B) and fermented (C). Peaks: (1) inorganic phosphate; (2–4) InsP1-2; (5) Ins(1,3,5)P3; (6) Ins(2,4,6)P3; (7) Ins(x,y,z)P3,; (8) Ins(1,2,6)P3; (9) Ins(x,y,z)P3; (10) DL-Ins(1,5,6)P3; (11) DL-Ins(4,5,6)P3; (12) DL-Ins(1,2,4,6)P4; (13) DL-Ins(1,2,3,4)P4; (14) DL-Ins(1,2,4,5)P4; (15) InsP4,; (16) DL-Ins(1,2,5,6)P4; (17) Ins(2,4,5,6)P4; (18) Ins(1,4,5,6)P4; (19) Ins(1,2,3,4,6)P5; (20) Ins(1,2,3,4,5)P5; (21) DL-Ins(1,2,4,5,6)P5; (22) Ins(v,w,x,y,z)P5; (23) Ins (1,3,4,5,6)P5, (24) Ins(1,2,3,4,5,6)P6.
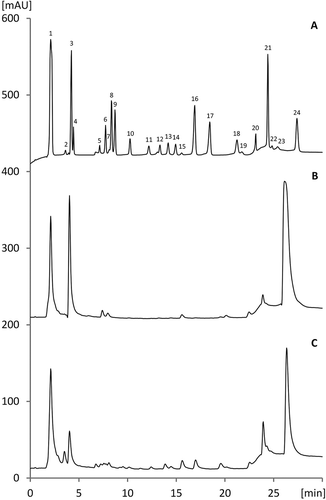
In vitro digestion model
Studies in humans showed that most of the dietary phytate is degraded during the digestion in the stomach and small intestine when the diet is rich – as could be expected in our study – in plant or microbial food phytases[Citation29]. In this experiment, we try to estimate if the process of InsP6 hydrolysis initiated during food processing will be continued after passage throughout the simulated intestine. The amount of phytate released into dialysate divided by its total content expressed in percentages was used as a bioavailability indicator. Cooking and tempe-type processing of buckwheat groats resulted in the decrease of this parameter to 27% from the level of 58% determined for cooked groats. These results were inconsistent with our previous study where bioavailability in buckwheat samples was between 69 and 45%; however, it can be explained by the additional purification procedure of inositol phosphates from the dialysates and different detection system applied. Among the quinoa seeds, bioavailability of phytate in previously fermented samples also decreased after in vitro digestion, especially in the case of white variety, to 15% (40 hour fermentation), as compared to the control (39%). In the coloured quinoa samples subjected to solid state fermentation, the similar tendency was found, with the 44% phytate bioavailability of the raw seeds and 33% or 31% for the fermented black and red ones, respectively. The in vitro bioavailability of phytate from the analysed pseudocereals was lowered as a result of fermentation, as compared to cooked and raw samples of respective material (white, red, black quinoa seeds, fair buckwheat groats). The obtained results suggest that, during the simulated digestion of previously fermented with Rhizopus strain samples, phytates present in the material could undergo further degradation by the mould phytases, being active in the material despite thermal treatments (cooking and steaming). Fungal phytases can show high thermal stability, as described by Simon and Igbasan[Citation30] and Azeke at al.[Citation31] Moreover, it is proven that thermal stability of fungal phytases can be even higher when they are included in food matrix, as compared to the highly purified form[Citation11]. The action of fungal phytases during the simulated digestion is possible, as they can show resistance to proteolytic activity during the gastrointestinal passage[Citation30].
The decrease in phytate bioavailability was accompanied by an increase of total myo-inositol contents in Chenopodium seeds. Analysis of the total concentration of myo-inositol (InsP0) in quinoa seeds fermented by 40 hours showed an 22% (white seeds) and 40% (black seeds) increase as compared to the substrate (32.2 µmol/g) (). The similar phenomenon was observed for the black quinoa, significant increase in sample subjected to solid state fermentation by 40hours (35.6 µmol/g), as compared to the control (25.2 µmol/g).
Conclusions
Hydrothermal processing and solid state fermentation of pseudocereals with R. oligosporus reduced content of antinutritional phytate, generated profile of lower inositol phosphates with a dominant share of inositol penta- and tetraphosphates (buckwheat) and increased levels of bioactive myo-inositol (quinoa). Observations from the in vitro model confirm that phytate once partially degraded through processing of seeds – especially in fermented quinoa samples – was further hydrolysed, probably by active food microbial or plant phytases, during simulated intestinal digestion. Concluding, the fermentation with R. oligosporus reduces the antinutritional properties of the important component of the raw product – phytate – resulting in the improvement of the food quality.
Acknowledgements
The authors would like to thank the Ministry of Science and Higher Education of Poland for financing the national grant number DS 3700/WTŻ/2015. The authors report no conflicts of interest. The authors alone are responsible for the content and writing of the paper.
References
- Handoyo, T.; Maeda, T.; Urisu, A.; Adachi, T.; Morita, N. Hypoallergenic Buckwheat Flour Preparation by Rhizopus oligosporus and Its Application to Soba Noodle. Food Research International 2006, 39, 598–605.
- Abderrahim, F.; Huanatico, E.; Segura, R.; Arribas, S.; Gonzalez, M.C.; Condezo-Hoyos, L. Physical Features, Phenolic Compounds, Betalains and Total Antioxidant Capacity of Coloured Quinoa Seeds (Chenopodium quinoa Willd.) from Peruvian Altiplano. Food Chemistry 2015, 183, 83–90.
- Ahmed, A.; Khalid, N.; Ahmad, A.; Abbasi, N.A.; Latif, M.S.Z.; Randhawa, M.A. Phytochemicals and Biofunctional Properties of Buckwheat: A Review. The Journal of Agricultural Science 2014, 152, 349–369.
- Arendt, E.; Zannini, E. Quinoa. Cereal Grains for the Food and Beverage Industries, Arendt, E.; Zannini, E.; Woodhead Publishing Limited: Cambridge, UK, 2013; 409–438.
- Alvarez-Jubete, L.; Arendt, E.K.; Gallagher, E. Nutritive Value of Pseudocereals and Their Increasing Use as Functional Gluten-Free Ingredients. Trends in Food Science and Technology 2010, 21, 106–113.
- Iglesias-Puig, E.; Monedero, V.; Haros, M. Bread with Whole Quinoa Flour and Bifidobacterial Phytases Increases Dietary Mineral Intake and Bioavailability. LWT – Food Science and Technology 2015, 60, 71–77.
- Rizzello, C.G.; Lorusso, A.; Montemurro, M.; Gobbetti, M. Use of Sourdough Made with Quinoa (Chenopodium quinoa) Flour and Autochthonous Selected Lactic Acid Bacteria for Enhancing the Nutritional, Textural and Sensory Features of White Bread. Food Microbiology 2016, 56, 1–13.
- Kumar, V.; Sinha, A.K.; Makkar, H.P.S.; Becker, K. Dietary Roles of Phytate and Phytase in Human Nutrition: A Review. Food Chemistry 2010, 120, 945–959.
- Lopez, H.W.; Leenhardt, F.; Coudray, C.; Remesy, C. Minerals and Phytic Acid Interactions: Is it a Real Problem for Human Nutrition? International Journal of Food Science and Technology 2002, 37, 727–739.
- Vucenik, I.; Shamsuddin, A.M.S. Cancer Inhibition by Inositol Hexaphosphate (IP6) and Inositol: From Laboratory to Clinic, Journal of Nutrition, 2003, 133, 3778S–3784S.
- Konietzny, U.; Greiner, R. Molecular and Catalytic Properties of Phytate-Degrading Enzymes (Phytases). International Journal of Food Science and Technology 2002, 37, 791–812.
- Ismail, A.; Riaz, M.; Akhtar, S.; Ismail, T.; Ahmad, Z.; Ali, S.W. Improvement of Quality Attributes of Bread by the Application of Phytases from an Indigenous Strain of Aspergillus niger. Pakistan Journal of Agricultural Sciences 2014, 51, 711–718.
- Haros, M.; Bielecka, M.; Honke, J.; Sanz, Y. Myo-Inositol Hexakisphosphate Degradation by Bifidobacterium infantis ATCC 15697. International Journal of Food Microbiology 2007, 117, 76–84.
- Egounlety, M.; Aworh, O.C. Effect of Soaking, Dehulling, Cooking and Fermentation with Rhizopus oligosporus on the Oligosaccharides, Trypsin Inhibitor, Phytic Acid and Tannins of Soybean (Glycine max Merr.), Cowpea (Vigna unguiculata L. Walp) and Groundbean (Macrotyloma geocarpa Ha. Journal of Food Engineering 2003, 56, 249–254.
- Rani, R.; Ghosh, S. Production of Phytase under Solid-State Fermentation using Rhizopus oryzae: Novel Strain Improvement Approach and Studies on Purification and Characterization. Bioresource Technology 2011, 102, 10641–10649.
- Feng, X.M.; Eriksson, A.R.B.; Schnürer, J. Growth of Lactic Acid Bacteria and Rhizopus oligosporus during Barley Tempeh Fermentation. International Journal of Food Microbiology 2005, 104, 249–256.
- Dorđević, T.M.; Šiler-Marinković, S.S.; Dimitrijević-Branković, S.I. Effect of Fermentation on Antioxidant Properties of Some Cereals and Pseudo Cereals. Food Chemistry 2010, 119, 957–963.
- Cai, S.; Gao, F.; Zhang, X.; Wang, O.; Wu, W.; Zhu, S.; Zhang, D.; Zhou, F.; Ji, B. Evaluation of γ- Aminobutyric Acid, Phytate and Antioxidant Activity of Tempeh-Like Fermented Oats (Avena sativa L.) Prepared with Different Filamentous Fungi. Journal of Food Science and Technology 2012, 51, 2544–2551.
- Starzyńska-Janiszewska, A.; Stodolak, B.; Duliński, R.; Mickowska, B. Effect of Solid-State Fermentation Tempe Type on Antioxidant and Nutritional Parameters of Buckwheat Groats as Compared with Hydrothermal Processing. Journal of Food Processing and Preservation 2015. doi:10.1111/jfpp.12607.
- Starzyńska-Janiszewska, A.; Duliński, R.; Stodolak, B.; Mickowska, B.; Wikiera, A. Prolonged Tempe-Type Fermentation in Order to Improve Bioactive Potential and Nutritional Parameters of Quinoa Seeds. Journal of Cereal Science 2016, 71, 116–121.
- Zyla, K.; Ledoux, D.R.; Veum, T.L. Complete Enzymic Dephosphorylation of Corn-Soybean Meal Feed under Simulated Intestinal Conditions of the Turkey. Journal of Agricultural and Food Chemistry 1995, 43, 288–294.
- Starzynska-Janiszewska, A.; Stodolak, B.; Dulinski, R.; Mickowska, B. The Influence of Inoculum Composition on Selected Bioactive and Nutritional Parameters of Grass Pea Tempeh Obtained by Mixed-Culture Fermentation with Rhizopus oligosporus and Aspergillus oryzae Strains. Food Science and Technology International 2012, 18, 113–122.
- Gambuś, H.; Matusz-Mirlak, A.; Duliński, R.; Ziobro, R.; Golachowski, A. The Influence of Extrusion Process on myo-Inositol Phosphate Content and Profile in Snacks Containing Rye Bran. International Journal of Food Sciences and Nutrition 2012, 63, 41–44.
- Duliński, R.; Cielecka, E.K.; Pierzchalska, M.; Żyła, K. Phytases Improve myo-Inositol Bioaccessibility in Rye Bread: A Study using an in vitro Method of Digestion and a Caco-2 Cell Culture Model. Food Technology and Biotechnology 2015, 53, 66–72.
- Blaabjerg, K.; Hansen-Møller, J.; Poulsen, H.D. High-Performance Ion Chromatography Method for Separation and Quantification of Inositol Phosphates in Diets and Digesta. Journal of Chromatography B 2010, 878, 347–354.
- Duliński, R.; Starzyńska-Janiszewska, A.; Stodolak, B.; Żyła, K. Comparison of High-Performance Ion Chromatography Technique with Microbiological Assay of myo-Inositol in Plant Components of Poultry Feeds. Journal of Animal and Feed Sciences 2011, 20, 143–156.
- Abu-Salem, F.M.; Abou-Arab, E.A. Physico-Chemical Properties of Tempeh Produced from Chickpea Seeds. Journal of American Science 2011, 7, 107–118.
- Fisher, S.K.; Novak, J.E.; Agranoff, B.W. Inositol and Higher Inositol Phosphates in Neural Tissues: Homeostasis, Metabolism and Functional Significance. Journal of Neurochemistry 2002, 82, 736–754.
- Sandberg, A.S.; Andersson, H. Effect of Dietary Phytase on the Digestion of Phytate in the Stomach and Small Intestine of Humans. The Journal of Nutrition 1988, 118, 469–473.
- Simon, O.; Igbasan, F. In Vitro Properties of Phytases from Various Microbial Origins. International Journal of Food Sciences and Technology 2002, 37, 813–822.
- Azeke, M.A.; Greiner, R.; Jany, K.-D. Purification and Characterization of Two Intracellular Phytases from the Tempeh Fungus Rhizopus oligosporus. Journal of Food Biochemistry 2011, 35, 213–227.