ABSTRACT
In this study, effects of NaCl concentrations (0.0, 0.2, 0.4, 0.6, 0.8, and 1.0 M) and pH (3.0, 5.0, 7.0, and 8.0) on the physico-chemical and functional properties of potato protein concentrates extracted from potato fruit juice (PFJ) using ammonium sulfate precipitation (ASP) and isoelectric precipitation (IEP) were investigated. The total polyphenol content of potato protein concentrates extracted by IEP (IEPP) was 2.5 times of that of potato protein concentrates extracted by ASP (ASPP), which resulted in the color of IEPP darker than that of ASPP. More protein fractions (74, 62, and 35 kDa) were found in IEPP compared to ASPP. ASPP exhibited higher solubility than IEPP. The emulsifying properties of ASPP and IEPP increased along with the increasing NaCl concentration. ASPP and IEPP showed the lowest emulsifying properties and foaming ability at pH 5.0. While the foaming stability of ASPP and IEPP achieved the maximum and minimum at pH 5.0, respectively. Two endothermic peaks were observed in ASPP at pH 3.0, 8.0, and higher NaCl concentration (≥0.6 M), while more than two endothermic peaks were observed in IEPP at all NaCl concentrations (0.2–1.0 M) and pH values (3.0, 5.0, 7.0, and 8.0). The glutamic acid concentration of ASPP and aspartic acid concentration of IEPP were the highest, while histidine was the lowest according to the amino acid profile of both ASPP and IEPP. In terms of an essential amino acid index with respect to a reference protein of FAO/WHO, the nutritional values of IEPP were higher than those of ASPP.
Introduction
Plant proteins have high nutrition value and bioavailability with low fat and caloric content. Besides, plant proteins possess good functional activities such as antioxidant, antidiabetic, and antifat activities with low price. Due to the above reasons, there is an increasing demand for the use of plant proteins as an alternative to animal proteins. Potato proteins include three major classes: patatin (up to 40%), protease inhibitors (~50%), and other proteins (~10%)[Citation1]. Compared to proteins from other vegetable and cereal sources, potato proteins are of great potential as food ingredients because of the higher nutritional quality[Citation2], the ability to regulate serum cholesterol levels[Citation3] and reduce food intake by increasing the circulation of cholecystokinin levels[Citation4]. Moreover, potato proteins have satisfactory functional properties such as good solubility, significant foaming, and emulsifying properties[Citation1,Citation5]. Therefore, the potato protein concentrates can be an interesting commodity potentially usable not only in the feed industry as well as in biotechnological applications, but also in the food industry, e.g. using as a fining agent for wine, a foaming agent instead of egg white protein, and an emulsifier to stabilize the oil–water system[Citation6–Citation12].
Potato fruit juice (PFJ) is an aqueous by-product of the potato starch industry. PFJ contains most of the tuber protein released after grinding of potato tubers in a rasping machine. One thousand kilograms of potatoes release 5–12 mCitation3 of PFJ[Citation5]. PFJ contains 2–5% dry matter, of which one third is protein, peptides, and amino acids/amines[Citation13]. Therefore, lots of research studies have focused on the extraction of potato proteins from PFJ.
Functional properties of food proteins that are of importance in food processing include solubility, foaming, and emulsifying properties[Citation14]. It is reported that the variation in the functional properties of proteins is associated with their structure[Citation14,Citation15]. Proteins with a native structure is essential in food processing because of their satisfactory functional properties[Citation15]. Studies conducted on the functional properties of potato proteins extracted using different techniques in the last few years have shown that their functional properties can vary[Citation1,Citation11,Citation12]. Hence, selection of the appropriate technology and conditions for protein extraction with a native structure is essential in food processing. To recover protein from PFJ without affecting their functional properties is challenging because of the aqueous nature of PFJ and its complex composition. The most common system of protein recovery from industrial PFJ includes heat coagulation and acid precipitation. Although thermal/acidic precipitation results in a high yield of protein recovery, it often leads to complete loss of the protein functionality, which limits their application only to animal feed and low-quality fertilizers[Citation16]. Lots of recovery techniques have been explored for the recovery of native protein isolation, including salt precipitation[Citation6], organic solvent precipitation[Citation6,Citation17], carboxymethyl cellulose (CMC) complexation[Citation7], chromatographic techniques[Citation8,Citation9], ultrafiltration,[Citation10] and the combination of the methods above.
Isoelectric precipitation (IEP) has been previously described as a common and traditional technique for recovery of proteins due to its easy operating, high recovery rate and suitable for large-scale production. Ammonium sulfate precipitation (ASP) is one of the most promising techniques for isolation of high yield of non-denatured proteins with high purity. There have been some reports on the potato protein concentrates extracted from PFJ, but limited information is available on the comparative study of the physico-chemical, functional and nutritional properties of potato protein concentrates extracted by ASP and IEP.
Therefore, this study seeks to compare the physico-chemical and functional properties of potato protein concentrates extracted by ASP and IEP. Besides, the amino acid composition and the effect of different NaCl concentrations and pH values on the physico-chemical and functional properties including solubility, emulsifying properties, foaming properties and thermal properties of the potato protein concentrates extracted by ASP and IEP will also be investigated in order to provide some theoretical basis for the application of potato protein concentrates.
Materials and methods
Materials
Fresh potatoes (cultivar: Atlantic) were kindly provided by the institute of Vegetables and Flowers, Chinese Academy of Agricultural Science. Coomassie Brilliant Blue G-250, ColorBurst Electrophoresis Marker and Folin–Ciocalteu reagent purchased from Sigma Chemical Co. (St-Louis, MO). All the other chemical reagents for chemical analysis were of analytical grade purchased from Sinopharm Chemical Teagent Beijing Co., Ltd.
Preparation of potato protein concentrates
Preparation of potato fruit juice
Potato fruit juice (PFJ) was prepared according to the method reported by Van Koningsveld et al.[Citation18] with slight modification. Briefly, peeled potatoes were washed thoroughly with water and cut into pieces which were immediately dipped into a 20 mg/mL solution of sodium bisulfite to prevent enzymatic browning. The potato pieces were ground in a domestic type juice extractor. The resulting turbid juice was allowed to settle for 15 min. Then, the liquid was decanted and centrifuged (15 min, 3000 g, 10°C) and the supernatant filtered through a medium speed quantitative filter paper with the pore diameter of 30 ~ 50 mm (Hangzhou Special Paper Co., Ltd, China). The resulting clear yellowish filtrate which had a pH of 5.7–6.0, was known to be comparable to industrial PFJ.
Preparation of potato protein concentrates
Ammonium sulfate precipitation (ASP) was performed according to the method reported by Waglay et al.[Citation19] with slight modification. Briefly, potato protein concentrate extracted by ASP (ASPP) was prepared from PFJ by adding ammonium sulfate ((NH4)2SO4) to 60% saturation. After incubation for one hour at 4°C, the suspension was centrifuged at 3000 g and 4°C for 30 min. The resulting precipitate was washed twice with half the starting volume of 50 mM sodium phosphate buffer (pH 7.0) that contained (NH4)2SO4 up to 60% saturation. Subsequently, the precipitate was suspended in distilled water and ultrafiltrated through regenerated cellulose filters with a molecular weight cut-off (MWCO) of 10 kDa. The retentate protein solution was freeze-dried and stored at −20°C for further use.
Isoelectric precipitation (IEP) was performed by adjusting the pH of PFJ to 4.0 with 1.0 M hydrochloric acid (HCl). Suspensions were then centrifuged for 40 min at 3000 g and 4°C. The recovered precipitation was suspended in water and the pH of the suspension was adjusted to 8.0 with 1 M sodium hydroxide (NaOH) to dissolve the potato protein[Citation20,Citation21]. The alkaline solution was centrifuged for 40 min at 3000 g and 4°C, the supernatant was collected and ultrafiltrated through regenerated cellulose filters with a MWCO of 10 kDa at ice-bath. The retentate protein solution was freeze-dried and stored at −20°C for further use.
Composition of potato protein concentrates
The composition of IEPP and ASPP was determined according to the methods as follows: Moisture content of was determined by drying the samples in an oven at 105°C overnight till the weight of the sample constant[Citation22]. Crude protein content was determined by the manual Kjeldah method, and the total nitrogen was converted to protein by using a factor of 6.25[Citation23]. Crude fat was determined by the Soxhlet extraction method[Citation22]. The ash content was determined by putting the sample after carbonization to muffle for 8 h at 550°C[Citation22]. The starch content was determined using a Total Starch Assay Kit[Citation22].
Sodium dodecyl sulfate–polyacrylamide gel electrophoresis (SDS–PAGE)
SDS–PAGE was performed according to Laemmli[Citation24]. A 40 μL sample solution was mixed with 10 μL of sample buffer. 5% and 12% acrylamide was used in the stacking and separation gels, respectively. Reduction of disulfide bonds was performed by adding 2-mercaptoethanol (5%, v/v) and heating at 100°C for 3 min. The electrophoresis was conducted at a constant current of 20 mA. The protein bands were visualized by Coomassie Brilliant Blue staining. The molecular mass of proteins was estimated using ColorBurst Electrophoresis Marker. Gel Imaging System Fluor Chem FC2 (Alpha, USA) was used to analyze the SDS–PAGE graph.
Color measurements
The visual appearance of ASPP and IEPP was measured using chroma meter CR-400 (Konica Minolta, Japan). Differences in color were expressed by means of the L*, a*, and b* values of the CIELab color space. The L* values range from 0 to +100, and represent black and white, respectively. The negative and positive a* values represent green and red, respectively. The negative and positive b* values represent blue and yellow, respectively.
Total polyphenol content
The total polyphenol content of IEPP and ASPP was determined according to the Folin–Ciocalteu method described by Velioglu et al.[Citation25] with slight modification. The samples were vigorously mixed with 20 times its equivalent volume of 80% ethanol. The mixture was extracted by ultrasonic wave at 50°C with the power of 59 Hz for 30 min. The extract was then centrifuged at 7000 g for 15 min, and the clear supernatants were obtained. 1.0 mL of 0.2 N Folin–Ciocalteu reagent was added to 500 μL of the supernatant, followed by the addition of 2.0 mL of 10% (w/v) sodium carbonate after about 30 min at 30°C. The mixture was incubated at 30°C for 30 min and the absorbance was measured at 736 nm using a UV spectrophotometer (UV-1101 Techcomp, China). 80% methanol and 1% HCl in place of protein sample was used as blank. 3-caffeoylquinic acid (3-CAE) standards of 0.05–0.3 mg/mL were used for the calibration curve (RCitation2 = 0.996). The total polyphenol content of the protein sample was expressed as mg 3-caffeoylquinic acid equivalent (CAE) per gram dry weight (mg CAE/g DW). The experiment was conducted in triplicate.
Functional properties
Solubility
Samples were solubilized in deionized water and the pH was adjusted from 1.0 to 10.0 with 0.1 M HCl and 0.1 M NaOH; deionized water was added to reach the exact concentration of 10 mg/mL. Samples were then centrifuged for 15 min at 3000 g until clear supernatants were obtained. The protein content of the obtained supernatants was analyzed in duplicate according to Lowry et al.[Citation26].
Emulsifying properties
Emulsifying properties were studied according to Pearce and Kinsella[Citation27] and Cameron et al.[Citation28] with some modification. 2.5 mL of corn oil was added to 7.5 mL of protein solution (1%, w/w). Effects of pH on emulsifying properties were evaluated by prepared 7.5 mL of protein solutions in 0.05 M phosphate buffer (disodium hydrogen phosphate–citric acid) with different pH (3.0, 5.0, 7.0, and 8.0). To study effects of salt on emulsifying, 7.5 mL of protein solutions were prepared in different salt solutions (0.0, 0.2, 0.4, 0.6, 0.8, and 1.0 M NaCl concentration). Mixtures were homogenized at 10000 rpm for 1 min with a mechanical blender (FJ-200). An aliquot of 20 μL of the freshly prepared emulsion was taken from the bottom of the test tube immediately after homogenization and diluted with 5 mL of 0.1% SDS solution. After vortexing, the absorbance of the dilution was measured at 500 nm. Emulsifying activity index (EAI) and emulsifying stability index (ESI) were calculated as described in Pearce and Kinsella[Citation27]. All analysis was conducted in triplicate.
Foaming properties
To produce the foam, 0.5 g of the samples were placed into a 15 mL centrifuge tube with 10 mL of different NaCl concentration solutions (0.0, 0.2, 0.4, 0.6, 0.8, and 1.0 M) or 0.05 M disodium hydrogen phosphate–citric acid buffer (pH 3.0, 5.0, 7.0, and 8.0) to study effects of salt (NaCl) and pH on foaming. The resulting solutions were whipped with a mechanical blender (FJ-200) for 2 min at 8000 rpm. The formed foam was set for certain minutes. Foaming ability and foaming stability were calculated as the following equations:
where V1 (mL) is the volume of the foam formed through homogeneous, and Vt (mL) is the volume of the foam after certain minutes, therein t = 30, 60, 90 min.
Differential scanning calorimetry (DSC)
The thermal properties of protein concentrates were determined by differential scanning calorimetry (DSC) (Q200 TA Instruments, New Castle, DE, USA) according to the procedure of Meng and Ma[Citation29] with some modification. Approximately 2.0 mg of protein concentrates were mixed with 10 μL of 0.05 M phosphate buffer (pH 3.0, 5.0, 7.0 and 8.0) or different NaCl concentration solutions (0.0 0.2, 0.4, 0.6, 0.8 and 1.0 M) and then sealed in aluminum pans. The pans were scanned from 20°C to 95°C at a rate of 5°C/min. A sealed empty pan was used as a reference. The peak denaturation temperature (Tp) and enthalpies of denaturation (ΔH) were recorded. Each sample was repeated three times and data were presented as means ± SD.
Determination of amino acid composition
Amino acid composition was determined after hydrolysis of ASPP and IEPP using 6 M HCl in a microwave oven (acid hydrolysis for 40 min at 150°C; oxidative hydrolysis for 40 min at 140°C) followed by separation of the amino acids by ion-exchange chromatography (column AminoPac PA1, Dionex, ThermoScientific, Sunnyvale, CA, USA) on HPLC system (Dionex DX-600, Dionex, ThermoScientific, Sunnyvale, CA, USA) using ninhydrin post-column derivatization. Initial oxidation to methionine sulfone and cysteic acid was carried out with a performic acid/phenol mixture before hydrolysis for determination of tuber protein quality. Two different standards, amino acid composition appropriate for an adult human and whole egg protein[Citation30] were used for EAAI estimation (EAAIadult and EAAIegg, respectively). The recommended levels of exogenous amino acids were as follows: Val 5 g/100 g pro, Met+ Cys 3.5 g/100 g pro, Ile 4 g/100 g pro, Leu 7 g/100 g pro, Phe + Tyr 6 g/100 g pro, Lys 5.5 g/100 g pro, and Trp 1 g/100 g pro for adult human standard; Val 6.6 g/100 g pro, Met+ Cys 5.7 g/100 g pro, Ile 5.4 g/100 g pro, Leu 8.6 g/100 g pro, Phe + Tyr 9.3 g/100 g pro, and Lys 7.0 g/100 g pro for whole egg protein standard. EAAI was calculated according to the method of Oser[Citation31].
where n is the number of participating amino acids and ns is the number of corresponding amino acids in standard.
Data analysis
Statistical analyses were performed using the Statistical Analysis System version 9.2 software (SAS Institute Inc., Cary, NC, USA). A significance level of p<0.05 was considered statistically significant.
Results and discussion
Proximate composition
shows the proximate composition of IEPP and ASPP. IEPP contained 85.80% (w/w) total protein, which was slightly higher than ASPP (83.20%, w/w). The starch and fat contents of ASPP were a little higher than those of IEPP. Since the largest mass of the potato proteins have a isoelectric point (pI) at acidic pH, precipitation of potato proteins at low pH has frequently been described using various acids[Citation17,Citation32]. However, for IEPP, after proteins were precipitated by adding acid until the pI (pH 2.5–5.0), the precipitated proteins were then resolved in alkaline (pH 8.0–10.0) and the insoluble matter was removed by centrifugation. Different from isoelectric precipitation, the hydrophobic interactions responsible for precipitating proteins out of solution when using (NH4)2SO4 is achieved with its dissociation to its ionic substances, which results in an increase at the protein’s surface. This surface tension leads to a decrease in hydrogen bonding between the water molecules and the protein thereby promoting precipitation[Citation19]. Therefore, the difference of proximate composition between ASPP and IEPP might be due to the different processing conditions and precipitation mechanisms.
Table 1. The proximate composition of potato protein (g/100 g sample).
SDS–PAGE
As can be seen from the SDS–PAGE analysis (), IEPP and ASPP showed significant difference (lane 1 and 2). Apart from some major bands of 129, 87, 43, 39, 24, 20, and 16 kDa at which the lane of ASPP and IEPP were both contained, some of minor bands were absent at lane of ASPP, such as the protein bands of 74, 62, and 35 kDa. In addition, IEPP presented more gradient of undefined bands than ASPP. SDS–PAGE analysis in the presence of β-mercaptoethanol showed that the protein band of 129 kDa was dissociated at ASPP (lane 4), while some new bands emerged (such as 14 kDa), which indicating the existence of aggregated polymers under the non-reductive conditions. Potato proteins include three major classes: patatin with molecular weight (MW) of 39–45 kDa, protease inhibitors with MW of 5–25 kDa and other proteins (all identified potato proteins other than patatin and protese inhibitors)[Citation1,Citation33]. Therefore, protein bands with MW of 43 and 39 kDa corresponded to different isoforms of patatin, protein bands with MW of 24, 20, 16, and 14 kDa corresponded to different protease inhibitors, protein bands with MW of 87 and 129 kDa corresponded to other proteins.
Figure 1. SDS–PAGE patterns of ASPP and IEPP. Lanes 1–5 were marker, ASPP, IEPP, ASPP in present of β-mercaptoethanol, and IEPP in present of β-mercaptoethanol, respectively.
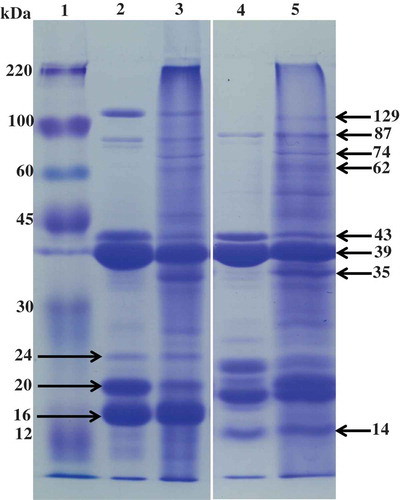
Not only the difference existed in the fractions of IEPP and ASPP, but also presented in the content of different fractions. The patatin and protease inhibitors were higher in ASPP than IEPP, especially the protein with MW 20 kDa (one kind of protease inhibitors). This could be attributed that using (NH4)2SO4 resulted mainly in the precipitation of resoluble patatin, while the group of protease inhibitors was eliminated during precipitation. These results were consistent with Waglay et al.,[Citation19] which found a higher relative protein proportion of patatin and the proteins with MW 15–20 kDa in (NH4)2SO4 precipitation (36.5% and 20.8%, respectively) than acidic precipitation (11.1% and 15.3%, respectively).
Color measurement and total polyphenol content
The color intensities (L*, a*, b*) of ASPP and IEPP are shown in . ASPP appeared brighter than IEPP for the L* value of ASPP (60.20 ± 0.02) was higher than that of IEPP (56.60 ± 0.01). The a* and b* value of IEPP was lower than ASPP, suggesting that ASPP is more reddish and yellow dish than IEPP. The total polyphenol content of IEPP was 2.5 times of that of ASPP. PFJ contains several non-protein components, of which the polyphenol compounds, mainly chlorogenic acid and caffeic acid[Citation34]. Strætkvern and Schwarz[Citation8] and Løkra et al.[Citation9] suggested that colored pigments in potato and fruits commonly arise from the oxidation of polyphenols and the low content of polyphenols contribute to high brightness values. Therefore, the lower content of total polyphenol in ASPP resulted in the brighter color than IEPP.
Table 2. Color values and total polyphenol content of ASPP and IEPP.
Functional properties
Protein solubility
To provide useful information toward effective utilization of potato proteins in various food applications, the solubility of the concentrates was investigated at pH ranging from 2.0 to 10.0 and NaCl concentration ranging from 0.0 to 1.0 M (). Overall, the solubility of ASPP is higher than IEPP in the range of pH and NaCl concentrations. shows that along with the increasing concentration of NaCl (from 0.0 to 1.0 M), the protein solubility of ASPP increased from 56.13% to 75.69% whereas the protein solubility of IEPP increased from 50.51% to 63.07% and fell to 58.07%. As can be seen from , the lowest solubility of ASPP and IEPP was observed at pH 4.0 (32.28% and 7.01% respectively), and the solubility increased quickly when pH > 6.0. Increasing the pH to 7.0 resulted in an increase in the protein solubility of ASPP and IEPP (from 32.28% and 7.01% at pH 4.0–81.64% and 69.52% at pH 7.0, respectively) significantly.
Figure 2. Effect of NaCl concentration (M) (A) and pH (B) on the solubility (%) of ASPP and IEPP. Values in the same graph with the different letter are significantly different (p < 0.05).
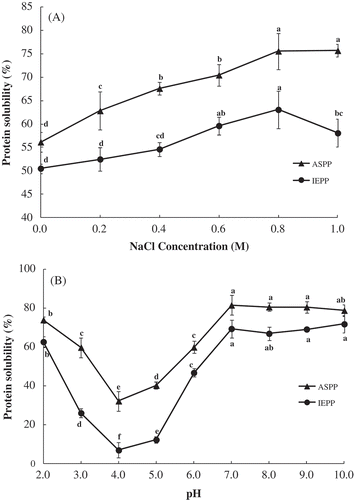
Studies conducted by other researchers also showed the solubility of protein isolates from potato to be lowest at pH 4–6 and highest at pH 8–9[Citation19,Citation32]. The little difference in pI could be due to the conformational changes, cross-linking, and changes in the net charge of proteins. However, the significant lower solubility of IEPP than ASPP could be due to the heavier loss of physico-chemical properties of IEPP. High ionic strength of (NH4)2SO4 can preserve the conformation of the patatin and protease inhibitors[Citation18], resulting in higher protein solubility of ASPP than IEPP. In addition, the polyphenols are considered to be responsible for a decrease in protein solubility during protein recovery. Polyphenols may interact covalently or non-covalently with proteins and both ways can lead to precipitation of proteins[Citation35]. The higher polyphenol contents in IEPP could partially explained the lower protein solubility of IEPP than that of ASPP. What’s more, IEPP tends to salting out at high NaCl concentration (1.0 M), which could be attributed to the increase of the ionic strength increased the binding strength between polyphenols and proteins. The aggregation of polyphenols and proteins could decrease the protein solubility significantly[Citation36].
Emulsifying properties
Emulsifying properties of food proteins can be described by the emulsifying activity (EAI) and emulsifying stability (ESI) indices. shows the effect of pH and NaCl on EAI and ESI. As the NaCl concentration increased, the EAI and ESI of ASPP and IEPP decreased first, then started to increase ( and ). Protein concentrates from sweet potato also presented similar trend[Citation37]. This could be attributed to the low concentration of salt ions reduce the electrostatic repulsion forces that help to form and maintain emulsion, while along with the increasing concentration of salt ions the electrostatic repulsion forces decreases[Citation37].
Figure 3. Effect of NaCl concentration (M) and pH on the emulsifying properties of ASPP and IEPP. Effect of NaCl concentration (M) (A) and pH (B) on the EAI (mCitation2/g). Effect of NaCl concentration (M) (C) and pH (D) on the ESI (mCitation2/g). Values in the same graph with the different letter are significantly different (p < 0.05).
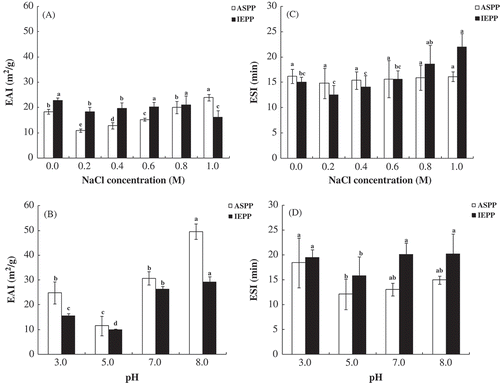
and show that ASPP and IEPP stabilized emulsions presented higher EAI at neutral and alkaline pH (7.0 and 8.0) than that at the proximity of its pI (pH 3.0 and 5.0). This could be attributed solely to the electrostatic effect. The surface charge concentration of protein molecules is the lowest at their pI and hence maintaining the pH of the protein dispersion at about their pI favors the aggregation of protein molecules due to the absence of the repulsive electrostatic forces[Citation1,Citation38]. It is worth noting that the ESI of IEPP was similar to or higher than ASPP, indicating that a certain degree of denaturation of potato protein contribute to stabilizing emulsions.
Foaming properties
The ability to form and stabilize foams is considered an important functional property of food proteins, which is exploited in several food products. The effect of salt concentration and pH on the ability and stability of foam whipped from ASPP and IEPP are presented in and . For ASPP, the foaming ability was increased from 75.2% to 200.0% with increasing NaCl concentration (). While for IEPP, the foaming ability was increased from 57.0% to 122.0% when NaCl concentration increased from 0.0 to 0.8 M (). As can be seen from , the foaming stability of ASPP and IEPP reached the maximum at 1.0 M and 0.8 M NaCl concentration 90 min after homogenization, respectively, indicating that the foams produced were more stable as the concentration of added salt (NaCl) increased. Especially at 1.0 M NaCl concentration, there was no decrease in the foaming stability for both ASPP and IEPP along with the time extension. Addition of NaCl improved foaming ability and stability could be due to the higher protein solubility of vegetable proteins in salt solutions[Citation39].
Table 3. Effect of NaCl concentration (M) (A) and pH (B) on the foaming stability of ASPP and IEPP.
Table 4. Amimo acid composition and nutritional value of ASPP and IEPP.
Figure 4. Effect of NaCl concentration (M) (A) and pH (B) on the foaming ability (%) of ASPP and IEPP. Values in the same graph with the different letter are significantly different (p< 0.05).
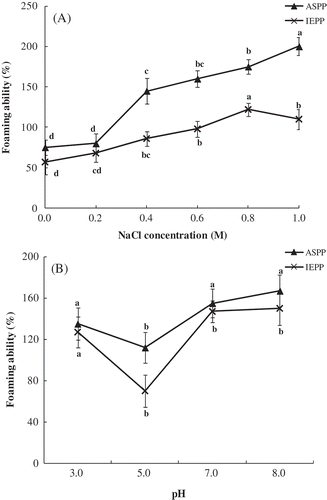
Foaming properties of ASPP and IEPP were pH-dependent ( and ). ASPP and IEPP presented the highest foaming ability at pH 8.0 and the lowest foaming ability at pH 5.0. The improved foaming abilities at the pH far away from pI could be explained by the higher solubility of the potato protein. According to , the foaming stability of ASPP was quite different from IEPP. ASPP presented the highest foaming stability along with the time extension (from 30 to 90 min after homogenization) and achieved maximum 90 min after homogenization at pH 5.0. While for IEPP, the foaming stability achieved minimum at pH 5.0. Foaming stability of some proteins is expected to be markedly improved in the neighborhood of their pI, where the decrease in electrostatic repulsion promotes the formation of a network structure in the protein film. However, some proteins tend to precipitate in the neighborhood of the pI and hence stability is decreased considerably[Citation11]. Therefore, the discrepancy of foaming stability between ASPP and IEPP could be explained like this: at pH near pI (pH 5.0), the solubility of IEPP was too low to stabilize the foam, while that of ASPP was higher and the decrease of electrostatic repulsion facilitates the stability of the foams.
Thermal properties
Thermal properties of ASPP and IEPP for the peak denaturation temperature (Tp) and enthalpy of denaturation (ΔH) can be determined directly by DSC. The effect of pH and NaCl concentration on the thermal properties of ASPP and IEPP are presented in . The major endothermic peaks with Tp of 55–75°C are consistent with previous reports[Citation18]. The endothermic peak of ASPP appeared significantly different from IEPP. ASPP showed two endothermic peaks at pH 3.0, 8.0, and higher NaCl concentration (≥0.6 M), while IEPP showed at least two endothermic peaks at all NaCl concentration and pH range. The enthalpy of denaturation (ΔH) of ASPP was higher than IEPP (data were not provided), suggesting that the denaturation of ASPP absorbed more heat than IEPP. As can be seen from and , on the increasing NaCl concentration, an obvious increase of Tp of ASPP and IEPP took place (from 68.94°C to 69.44°C and 67.34°C to 68.27°C, respectively), while the enthalpy of denaturation (ΔH) increased significantly from 8.19 J/g to 13.63 J/g and 1.17 J/g to 3.02 J/g, respectively (data was not shown). This result suggests that the NaCl solution (<1.0 M concentrations) could stabilize the potato protein conformation. The stabilizing effect of NaCl solution was attributed to the induction of alteration of water structure around the protein, which enhanced the hydration of the protein molecules[Citation40]. Similar results were also reported on red bean globulin by Meng and Ma[Citation23].
Figure 5. Effect of NaCl concentration (M) (A) and pH (B) on the endothermic peak of ASPP. Effect of NaCl concentration (M) (C) and pH (D) on the endothermic peak of IEPP. Arrows point to the heat absorption peak.
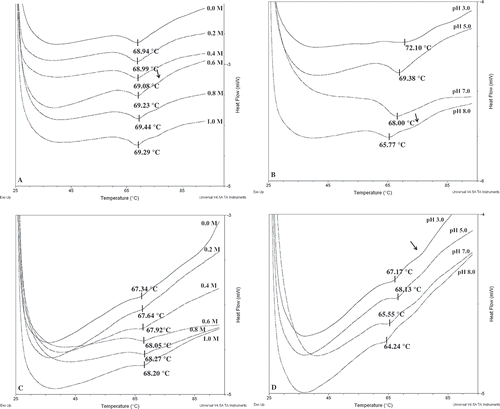
ASPP and IEPP displayed highest ΔH (13.16 J/g and 1.05 J/g, respectively) at pH 5.0, suggesting more heat required for complete denaturation. Similar observations on the effect of pH have been made on other plant proteins such as fababean protein and oat globulin[Citation41,Citation42]. Most proteins are stable over a specific pH range, normally near the pI, when the repulsive forces are quite low and therefore the proteins remain in a native state[Citation43].
Amino acid composition
shows the amino acid composition of ASPP and IEPP. 17 amino acids were determined in potato proteins, including 10 of essential amino acids and 7 of non-essential amino acids. As can be seen from , high levels of aspartic acid, glutamic acid, leucine, lysine, phenylalanine, valine, and threonine were observed (5–12%), which play important roles in protein quality. Lysine is often presented as the first and threonine as the second limiting amino acid in wheat, barley and rye[Citation44]. Therefore, ASPP and IEPP could be recommended as a supplement to cereal proteins. Alanine, serine, proline, arginine, isoleucine, tyrosine, and glycine presented in lower but significant levels (4–8%). Cysteine, methionine, and histidine stated small share in ASPP and IEPP (<3%). The concentration of glutamic acid and aspartic acid was the highest for IEPP and ASPP, respectively, while histidine was the lowest for both. The differences between the results we observed and previous works[Citation6,Citation45] could be attributed to the research materials and potato species.
The mean nutritional value of ASPP and IEPP was calculated as essential amino acid index (EAAI) related to whole-egg and adult standard (EAAIegg and EAAIadult). Although the essential amino acid/amino acid ratio (EAA/AA) of ASPP (41.69%) was a little lower than IEPP (42.91%), the EAAIegg and EAAIadult of ASPP were significantly higher than IEPP, indicating the better nutritional value of ASPP than IEPP.
Conclusion
Protein concentrates with acceptable functional and physico-chemical properties and nutritional quality might be extracted from industrial potato fruit juice using ASP and IEP. At pH close to pI, ASPP and IEPP showed very different behavior compared to other pH values, such as the lowest solubility, emulsifying ability, foaming ability and the highest denaturation temperature. Along with the increasing NaCl concentration, the solubility, emulsifying properties, foaming properties and denaturation temperature all increased obviously. However, not only some functional and physico-chemical properties of ASPP were more preference than IEPP, but also the nutritional value of ASPP was better than IEPP according to the amino acid composition. This comprehensive comparison study will help with the improvement of the extraction of minimally-modified proteins from PFJ and contribute to the conversion of this by-product into value-added ingredients. While on the basis of different physico-chemical, functional, and nutritional value of ASPP and IEPP, further research studies are needed to apply ASPP or IEPP into food processing.
Acknowledgments
The authors are grateful to the Institute of Vegetables and Flowers, Chinese Academy of Agricultural Science for providing the fresh potatoes (cultivar: Atlantic).
Funding
The author gratefully acknowledges the earmarked fund for the public welfare industry (Agriculture) research project: research and demonstration of key technology system of potato Staple Food (201503001-2), and the agricultural special financial in 2015 of pilot technology research and Demonstration of Potato Staple Products.
Additional information
Funding
References
- Ralet, M.C.; Guéguen, J. Fractionation of Potato Proteins: Solubility, Thermal Coagulation and Emulsifying Properties. LWT-Food Science and Technology 2000, 33(5), 380–387.
- Seo, S.; Karboune, S.; Archelas, A. Production and Characterisation of Potato Patatin-Galactose, Galactooligosaccharides, and Galactan Conjugates of Great Potential as Functional Ingredients. Food chemistry 2014, 158, 480–489.
- Liyanage, R.; Han, K.H.; Watanabe, S.; Shimada, K.I.; Sekikawa, M.; Ohba, K.; Fukushima, M. Potato and Soy Peptide Diets Modulate Lipid Metabolism in Rats. Bioscience, Biotechnology, and Biochemistry 2008, 72(4), 943–950.
- Komarnytsky, S.; Cook, A.; Raskin, I. Potato Protease Inhibitors Inhibit Food Intake and Increase Circulating Cholecystokinin Levels by a Trypsin-Dependent Mechanism. International Journal of Obesity 2011, 35(2), 236–243.
- Wojnowsk, I.; Poznanski, S.; Benarski, W. Processing of Potato Protein Concentrates and their Properties. Journal of Food Science 1982, 47(1), 167–172.
- Bártová, V.; Bárta, J. Chemical Composition and Nutritional Value of Protein Concentrates Isolated from Potato (Solanum tuberosum L.) Fruit Juice by Precipitation with Ethanol or Ferric Chloride. Journal of Agricultural and Food Chemistry 2009, 57(19), 9028–9034.
- Vikelouda, M.; Kiosseoglou, V. The Use of Carboxymethylcellulose to Recover Potato Proteins and Control their Functional Properties. Food hydrocolloids 2004, 18(1), 21–27.
- Strætkvern, K.O.; Schwarz, J.G. Recovery of Native Potato Protein Comparing Expanded Bed Adsorption and Ultrafiltration. Food and Bioprocess Technology 2012, 5(5), 1939–1949.
- Løkra, S.; Schüller, R.B.; Egelandsdal, B.; Engebretsen, B.; Strætkvern, K.O. Comparison of Composition, Enzyme Activity and Selected Functional Properties of Potato Proteins Isolated from Potato Juice with Two Different Expanded Bed Resins. LWT-Food Science and Technology 2009, 42(4), 906–913.
- Chen, Y.; Pan, X.Q.; Zhong, Z.S.; Wang, J. Study on Recovery of Potato Protein from Starch Producing Waste Water by Ultrafitration. Food Research and Development 2010, 9, 014.
- Ralet, M.C.; Guéguen, J. Foaming Properties of Potato Raw Proteins and Isolated Fractions. LWT-Food Science and Technology 2001, 34(4), 266–269.
- Gambuti, A., Rinaldi, A., Romano, R., Manzo, N., Moio, L. Performance of a Protein Extracted from Potatoes for Fining of White Musts. Food Chemistry 2016, 190, 237–243.
- Knorr, D.; Kohler, G.O.; Betschart, A.A. Potato Protein Concentrates: The Influence of Various Methods of Recovery upon Yield, Compositional and Functional Characteristics. Journal of Food Processing and Preservation 1977, 1(3), 235–247.
- Boye, J.I., Aksay, S., Roufik, S., Ribéreau, S., Mondor, M., Farnworth, E., Rajamohamed, S.H. Comparison of the Functional Properties of Pea, Chickpea and Lentil Protein Concentrates Processed using Ultrafiltration and Isoelectric Precipitation Techniques. Food Research International 2010, 43(2), 537–546.
- Damodaran, S. Structure-Function Relationship of Food Proteins. In Protein Functionality in Food Systems; Hettiarachchy, N. S., Ziegler, G. R.; Eds.; CRC Press, 1994; 1–37.
- Miedzianka, J.; Pęksa, A.; Aniołowska, M. Properties of Acetylated Potato Protein Preparations. Food Chemistry 2012, 133(4), 1283–1291.
- Barta, J.; Heřmanová, V.; Diviš, J. Effect of Low-Molecular Additives on Precipitation of Potato Fruit Juice Proteins under Different Temperature Regimes. Journal of Food Process Engineering 2008, 31(4), 533–547.
- Van Koningsveld, G.A.; Gruppen, H.; de Jongh, H.H.; Wijngaards, G.; van Boekel, M.A.; Walstra, P.; Voragen, A.G. Effects of pH and Heat Treatments on the Structure and Solubility of Potato Proteins in Different Preparations. Journal of Agricultural and Food Chemistry 2001, 49(10), 4889–4897.
- Waglay, A.; Karboune, S.; Alli, I. Potato Protein Isolates: Recovery and Characterization of their Properties. Food Chemistry 2014, 142, 373–382.
- Castel, V., Andrich, O., Netto, F.M., Santiago, L.G., Carrara, C.R. Comparison between Isoelectric Precipitation and Ultrafiltration Processes to obtain Amaranth Mantegazzianus Protein Concentrates at Pilot Plant Scale. Journal of Food Engineering 2012, 112(4), 288–295.
- Mu, T.H., Tan, S.S., Xue, Y.L. The Amino Acid Composition, Solubility and Emulsifying Properties of Sweet Potato Protein. Food Chemistry, 2009, 112(4), 1002–1005.
- AOAC. Official Methods of Analysis; 16th ed.; Association of Official Analytical Chemists: Washington, DC, 1995; Methods No. 923.03, 925.09, 960.36, 996.11.
- Van Gelder, W.M.J. Conversion Factor from Nitrogen to Protein for Potato Tuber Protein. Potato Research 1981, 24(4), 423–425.
- Laemmli, U.K. Cleavage of Structural Proteins during the Assembly of the Head of Bacteriophage T4. Nature 1970, 227, 680–685.
- Velioglu, Y.S.; Mazza, G.; Gao, L.; Oomah, B.D. Antioxidant Activity and Total Phenolics in Selected Fruits, Vegetables, and Grain Products. Journal of Agricultural and Food Chemistry 1988, 46(10), 4113–4117.
- Lowry, O.H.; Rosebrough, N.J.; Farr, A.L.; Randall, R.J. Protein Measurement with the Folin Phenol Reagent. The Journal of Biological Chemistry 1951, 193(1), 265–275.
- Pearce, K.N.; Kinsella, J.E. Emulsifying Properties of Proteins: Evaluation of a Turbidimetric Technique. Journal of Agricultural and Food Chemistry 1978, 26(3), 716–723.
- Cameron, D.R.; Weber, M.E.; Idziak, E.S.; Neufeld, R.J.; Cooper, D.G. Determination of Interfacial Areas in Emulsions using Turbidimetric and Droplet Size Data: Correction of the Formula for Emulsifying Activity Index. Journal of Agricultural and Food Chemistry 1991, 39(4), 655–659.
- Meng, G.T.; Ma, C.Y. Thermal Properties of Phaseolus angularis (Red Bean) Globulin. Food Chemistry 2001, 73(4), 453–460.
- Martínez-Villaluenga, C.; Gulewicz, P.; Frias, J.; Gulewicz, K.; Vidal-Valverde, C. Assessment of Protein Fractions of Three Cultivars of Pisum sativum L.: Effect of Germination. European Food Research and Technology 2008, 226(6), 1465–1478.
- Oser, B. L. An Integrated Essential Amino Acid Index for Predicting the Biological Value of Proteins. In Protein and Amino Acid Nutrition; Albanese A. A., Eds.; New York: Academic Press, 1959; 295–311.
- van Koningsveld, G.A.; Gruppen, H.; de Jongh, H.H.J.; Wijngaards, G.; van Boekel, M.A.J.S.; Walstra, P.; Voragen, A.G.J. The Solubility of Potato Proteins from Industrial Potato Fruit Juice as Influenced by pH and Various Additives. Journal of the Science of Food and Agriculture 2002, 82(1), 134–142.
- Pots, A.M.; Gruppen, H.; van Diepenbeek, R.; van der Lee, J.J.; van Boekel, M.A.J.S.; Wijngaards, G.; Voragen, A.G.J. The Effect of Storage of Whole Potatoes of Three Cultivars on the Patatin and Protease Inhibitor Content; A Study Using Capillary Electrophoresis and MALDI‐TOF Mass Spectrometry. Journal of the Science of Food and Agriculture 1999, 79(12), 1557–1564.
- Haslam, E. Practical Polyphenolics: From Structure to Molecular Recognition and Physiological Action; Cambridge University Press: Cambridge, British, 1998.
- Friedman, M.; McDonald, G.M.; Filadelfi-Keszi, M. Potato Glycoalkaloids: Chemistry, Analysis, Safety, and Plant Physiology. Critical Reviews in Plant Sciences 1997, 16(1), 55–132.
- Prigent, S.V.E. Interactions of Phenolic Compounds with Globular Proteins and their Effects on Food-Related Functional Properties; Wageningen Universiteit Press: Netherland, 2005; 132.
- Guo, Q.; Mu, T.H. Emulsifying Properties of Sweet Potato Protein: Effect of Protein Concentration and Oil Volume Fraction. Food Hydrocolloids 2011, 25(1), 98–106.
- Van Koningsveld, G.A.; Walstra, P.; Voragen, A.G.; Kuijpers, I.J.; Van Boekel, M.A.; Gruppen, H. Effects of Protein Composition and Enzymatic Activity on Formation and Properties of Potato Protein Stabilized Emulsions. Journal of Agricultural and Food Chemistry 2006, 54(17), 6419–6427.
- Pant, R.; Tulsiani, D.R.P. Solubility, Amino Acid Composition, and Biological Evaluation of Protein Isolated from Leguminous Seeds. Journal of Agricultural and Food Chemistry 1969, 17(2), 361–366.
- Arakawa, T.; Timasheff, S.N. Preferential Interactions of Proteins with Salts in Concentrated Solutions. Biochemistry 1982, 21(25), 6545–6552.
- Arntfield, S.D.; Murray, E.D. The Influence of Processing Parameters on Food Protein Functionality I. Differential Scanning Calorimetry as an Indicator of Protein Denaturation. Canadian Institute of Food Science and Technology Journal 1981, 14(4), 289–294.
- Harwalkar, V.R.; MA, C.Y. Study of Thermal Properties of oat Globulin by Differential Scanning Calorimetry. Journal of Food Science 1987, 52(2), 394–398.
- Morrissey, P.A.; Mulvihill, D.M.; O’Neill, E.M. Functional Properties of Muscle Proteins. Developments in Food Proteins 1987, 5, 195–256.
- Peksa, A.; Kita, A.; Kułakowska, K.; Aniołowska, M.; Hamouz, K.; Nems, A. The Quality of Protein of Coloured Fleshed Potatoes. Food Chemistry 2013, 141(3), 2960–2966.
- Bártová, V; Bárta, J.; Brabcová, A.; Zdráhal, Z.; Horáčková, V. Amino Acid Composition and Nutritional Value of Four Cultivated South American Potato Species. Journal of Food Composition and Analysis 2015, 40, 78–85.