ABSTRACT
Dried egg white is extensively used as a food ingredient due to its unique functional properties and extensive shelf life. This study investigated the effect of drying methods and storage conditions on the color and gelling properties of dried egg white. Egg white was dried with two drying methods; freeze drying and hot-air drying, then stored at 25 and 40°C for 4 months. The result showed that the color of hot-air-dried egg white, especially after storage at 40°C, was darker yellow than for freeze-dried egg whites. The gelling properties of both samples were altered during storage, however, substantial changes in the gelling properties were found in the gel made from stored hot-air-dried egg white. A decrease in the enthalpy of protein denaturation that indicated a partially unfolded protein conformation, an increase in exposed sulfhydryl and a decrease in the total sulfhydryl contents were found, especially in stored samples of hot-air-dried egg whites. The sodium dodecyl sulfate-polyacrylamide gel electrophoresis pattern of proteins in the stored samples showed protein aggregation, and this was related to the increased glass transition temperature. This study revealed that drying egg white using hot-air drying and storage especially at high temperature induced changes in their color and protein conformation. These have contributed to protein aggregation which affected dried egg white’s gel properties.
Introduction
Hen egg white (HEW) protein has unique characteristics, such as gel formation, water holding capacity (WHC), foaming, and emulsifying properties, and is extensively used in many food applications.[Citation1] Currently, instead of liquid eggs, dried-egg products are being widely used in many foods, such as bakery products. This is due to not only the nutritional and functional properties of dried egg products, but also several other advantages including storage, transportation, uniformity, and microbiological safety.[Citation2] Moreover, the drying process can also reduce the problems of oversupply and lack of egg stock. Thailand has a problem with the fluctuation of egg production that sometimes excess the supply but sometimes lack of egg stock. These problems greatly impact on the both consumer and egg producer. Thus, the dehydration of eggs is one method which can solve these problems because it can extend the egg’s shelf life and can preserve the egg for consumption throughout the year.
Drying can be carried out using many methods including hot-air drying and freeze drying. Freeze drying is based on dehydration by sublimation of water from the frozen product. Due to the absence of liquid water and the low temperature required for the process, most deterioration and microbiological reactions are retarded, which result in a freeze-dried product of high quality.[Citation3] Despite the high quality of the product, freeze drying has always been recognized as an expensive process for manufacturing a dehydrated product. A cheaper drying process—hot-air drying—is commonly used in the drying process. Hot-air-dried products can have an extended shelf life, but, unfortunately, the quality of the dried product is usually reduced compared to the original foodstuff[Citation4] as a result of the high temperature used during the drying process.
The quality of a dehydrated product depends not only on the drying methods, but also on the storage conditions. There are reports on the effect of storage conditions on the physical and functional properties of dried egg white. Kato et al.[Citation5] reported that the foaming power, foaming stability, emulsifying properties, and gel strength of spray-dried egg white were enhanced by increasing the sample’s incubated heating time in a dry-heated state (sample storage in a hot room under controlled temperature and moisture conditions). Van der Plancken et al.,[Citation6] who studied the effect of moisture content during dry-heating at 80°C on the physicochemical and functional properties including the foaming properties of freeze-dried egg white (FDEW), found that the moisture content is an important parameter in the dry-heating of egg white proteins. The foaming ability of the dried egg white increased at longer dry-heating times and the foaming ability improved more rapidly with a high moisture content of the sample. Rao et al.[Citation7] determined the influence of water activity (aw) on the storage quality of a commercial spray dried hydrolyzed HEW powder. They reported that the color of the hydrolyzed HEW changed after storing the sample at 45°C for 1 month and several structural changes occurred at aw from 0.43 to 0.79 including agglomeration, stickiness, and collapse. Rao and Labuza[Citation8] studied the effect of moisture content on the physicochemical properties of commercial HEW powder. The result showed that when the samples were stored at 0.85 aw, the dried samples agglomerated slightly which resulted in an increase in the hardness value of the sample. Rao et al.[Citation9] studied the moisture-induced quality changes of HEW proteins in a protein/water model system. The result showed the effect of moisture content on both the L* and fluorescence intensity values occurred as a function of storage time at 45°C due to the Maillard reaction. Moreover, Katekhong and Charoenrein[Citation10] reported that storage of FDEW at high temperature and relative humidity levels induced protein conformation changes which had an effect on the gelling properties of FDEW during storage.
In the literature, there are negligible reports available on the comparison of FDEW and hot-air-dried egg white (HDEW) on the properties of dried egg white. Therefore, the aim of this study was to compare the freeze drying and hot-air drying process in term of the physical and gelling properties of the dried products. Furthermore, this study was done on the storage stability of FDEW and HDEW as a function of temperature and time. The results of this study can be utilized to produce and maintain good quality dried egg whites during storage.
Materials and methods
Materials
Fresh eggs (hen, Betagro Layer) were purchased from Luang Suwan Vajokkasikij Farm, Kasetsart University, Thailand. They were selected to be homogeneous in size (weight of whole egg 65–70 g) and grade which was determined using a Haugh unit (H.U.) micrometer.[Citation11] In this study, eggs with grade AA (H.U. >72) and A (H.U. = 60–71) were chosen.
Preparation of FDEW and HDEW
The egg white was separated from the yolk and stirred using a stirrer (IKA®C-MAG HS 7, Staufen, Germany). The chalazae were removed in order to ensure a uniformity of egg white samples, then the egg white was freeze dried (GAMMA 1-16 LSD, Martin Christ, Germany) and hot-air dried. For hot-air drying, the egg white was spread on a tray and dried (Memmert, ULE500, Germany) at 45°C for 24 h. Freeze-dried and hot-air-dried samples were prepared in duplicate.
Storage of FDEW and HDEW
FDEW and HDEW samples were packed in an aluminum foil bag and stored at 25 and 40°C for 1, 2, and 4 months. The samples were removed at designated time intervals and cooled to room temperature before being analyzed. The experiments were replicated twice.
Color measurement
The colors of the samples were determined using a colorimeter (MiniScan XE, Hunter Lab, USA) to measure the CIE color parameters (L*, a*, and b*). In this system, the coordinate L* indicates lightness on a scale from 0 (black) to 100 (white); a* is + (red) or – (green) and b* is + (yellow) or – (blue). The total color differences (ΔE*) were obtained using Eq. (1), where ΔL*, Δa*, and Δb* define the color differences in the samples before and after storage under the various storage conditions. Measurement of each sample was done in triplicate.
Gel preparation
The gel of dried egg white sample was prepared using the method of Katekhong and Charoenrein.[Citation10] The unstored and the stored FDEW and HDEW samples were dissolved in distilled water with 12.2% solid content (solid content equal to fresh egg white) and adjusted to pH 7.0 using 2 M HCl or 2 M NaOH. The sample solutions were then loaded into a plastic cup (18 g) and heated for 30 min in a water bath (Memmert, WB22, Germany) at 90°C to achieve gelling. The gels were then held 20 h at 4°C before testing their gelling properties of gel hardness and WHC. Fresh egg white was also studied for comparison with FDEW and HDEW samples.
Gelling properties measurement
Gel hardness
The gel samples which had been stored in a plastic cup were measured with a compression test using a texture analyzer (Stable Micro System, TA-XT plus, UK). The samples were compressed with a P/25 cylinder probe at a test speed of 1 mm/s. The hardness was measured by compression at 40% strain. The maximum positive force was recorded and expressed as the hardness (N) of the sample. The measurement of each sample was done in five replicates.
%WHC
The %WHC of the gel was measured using a method adapted from Charoenrein et al.[Citation12] The gel samples were removed from their plastic cups and cut into four uniform pieces (wedge shape; 1.3 cm × 1.3 cm × 1 cm). Next, the gel samples were put in a cylindrical plastic tube with a perforated bottom covered with filter paper (Whatman No. 41). These tubes were then placed in centrifuge tubes and centrifuged at 2000 × g, at 25°C (centrifuge CN-1050, MRC Ltd., Holon, Israel) for 15 min. The cylindrical plastic tube and cover were then removed from the centrifuge tube and the liquid which had separated from the gel was weighed. The %WHC of the gel was determined using Eq. (2). The measurements of each sample were done in triplicate.
Protein denaturation measurement
The denaturation enthalpy (∆H) of fresh egg white, FDEW, and HDEW proteins were measured using a method modified from Thirathumthavorn and Charoenrein[Citation13] and analyzed using a differential scanning calorimeter (DSC; Pyris-1, Perkin Elmer, Norwalk, CT, USA). The unstored and stored samples (1.6–2 mg dry basis) were transferred into stainless steel pans and mixed with distilled water to give a final concentration of 12.2% solid content (solid content equal to fresh egg white) and then hermetically sealed. An empty stainless steel pan was used as a reference. Each sample was heated from 20 to 120°C at a rate of 10°C/min to measure the enthalpy of egg white protein denaturation. The measurements were performed in duplicate.
Exposed and total sulfhydryl (SH) group measurement
Measurement of SH groups was performed using Ellman’s reagent[Citation14] (5,5´-dithiobis 2-nitrobenzoic acid; DTNB) The content of exposed and total SH groups was measured in duplicate using a method modified from Van der Plancken et al.[Citation15] The determination of exposed SH groups, 5 mL of unstored and stored dried egg white sample (4 months) solutions in distilled water (5% w/v dry basis) was added with 5 mL Tris-glycine buffer (0.1 M Tris, 0.1 M glycine and 4 mM ethylenediamine-tetraacetic acid disodium (EDTA), pH 8.0). After a 15 min reaction time with 0.1 mL Ellman’s reagent (4 mg/mL DTNB in Tris-glycine buffer) at ambient temperature, the reaction mixture was centrifuged at 19,900 ×g, 4°C for 15 min. The absorbance of the supernatant was measured at 412 nm. For the total SH group determination, the samples solutions in distilled water (5% w/v dry basis) were diluted 30 times, then, 5 mL of each sample solution was added with 5 mL of 5% SDS in Tris-Glycine buffer and 0.1 mL Ellman’s reagent. The reaction mixture was kept at 40°C in a water bath (Memmert, WB22, Germany) for 15 min to allow the protein to unfold and all SH groups to be accessible to DTNB. Finally, the SH determination was continued as described in the exposed SH group procedure. The SH contents were calculated using a molar extinction coefficient of 13,600 M−Citation1cm−Citation1.[Citation15]
Gel electrophoresis
Sodium dodecyl sulfate-polyacrylamide gel electrophoresis (SDS-PAGE) in the presence and absence of 2-mercaptoethanol (2-ME) was carried out according to the method of Laemmli[Citation16] using 12% acrylamide separating gel and 4% stacking gel. Samples were mixed with a buffer. After 4 min of boiling, the sample solutions were centrifuged at 2700 × g for 10 min. Next, 10 µL of each sample solution, containing 20 µg of protein was loaded into separate wells. Electrophoresis was performed at a constant voltage (150 V) for 45 min using a Bio-Rad Mini-Protean II cell (Bio-Rad Laboratories; Hercules, CA, USA). After electrophoresis, the gel sheets were stained with a staining solution for 30 min and destained for 2.5 h. The molecular weight (MW) of each band was determined using MW markers (Precision plus, proteinTM, All-blue Standards #161-0373, Bio-Rad, UK).
Glass transition temperature (Tg)
The Tg value was determined using a DSC (Pyris-1, Perkin Elmer, Norwalk, CT, USA). Each 5 mg of unstored and stored samples (4 months) were transferred into DSC pans and immediately sealed. An empty pan was used as a reference. Triplicate samples were cooled from 25 to –60°C at a rate of 20°C/min, held for 2 min at –60°C and subsequently heated to 160°C at a rate of 5°C/min. The Tg values were taken from the midpoint of the heat capacity (Cp) change over the glass transition.
Statistical analysis
The experiments were done in duplicate. The data were subjected to analysis of variance and mean comparisons were carried out using Duncan’s new multiple range test. Statistical analyses were performed using the Statistical Package for Social Science (SPSS 12.0 for Windows, SPSS Inc., Chicago, IL, USA).
Results and discussion
Color
The color values (L*, a*, b*, and ∆E*) of unstored and stored dried egg white samples are shown in . When considering the color of unstored FDEW and HDEW samples, significantly differ (p ≤ 0.05) in the color value of samples was found in the b* value which indicated the yellowness of the sample. The b* value of HDEW was higher than FDEW which was probably due to the occurrence of non-enzymatic browning reaction (Maillard reaction). Egg white contains glucose which is a reducing sugar and an amino group in the egg white protein,[Citation17] thus, the darker yellow in HDEW might have been due to the Maillard reaction during the hot-air drying process. The ΔE* was calculated with respect to the color of unstored sample, the results showed that there was a slight increase in the ΔE* value of FDEW during storage. However, a substantial increases in the ΔE* value was found in HDEW. This result might have been due to the effect of water in the sample. The water content of HDEW was higher than in FDEW (), for this reason, it is possible that the Maillard reactants in the HDEW sample had a higher molecular mobility than that in FDEW which caused a higher browning rate. The ΔE* value of HDEW stored at 40°C was higher than that stored at 25°C which demonstrated that temperature during storage had an influence on the changes in the color of sample. In contrast, the ΔE* values of FDEW stored at 25 and 40°C were not significantly differ (p ≥ 0.05) which might have been due to the low molecular mobility of the Maillard reactants. However, clear changes in the values of L*, a*, b*, and ΔE* of the HDEW samples were found at the beginning of the storage period (storage time 0–2 months). After storage for 2 months, the rate of the color changes was reduced possibly due to glucose, as the limiting reactant, being consumed during the initial storage time.[Citation10]
Table 1. Gelling properties (gel hardness and %WHC), protein denaturation enthalpy (∆H), water content, and midpoint Tg of unstored and stored FDEW and HDEW.
Gelling properties
Gelling properties consisting of gel hardness and %WHC, were determined for gel samples made from unstored and stored FDEW and HDEW as well as from fresh egg white. The moisture contents of the FDEW and HDEW were 3.97 ± 0.33% and 9.34 ± 0.17% (wet basis), respectively. The results () showed that the hardness value and %WHC of the gel samples made from fresh egg white and unstored FDEW and HDEW were not significantly different (p ≥ 0.05) which indicated that the processes of freeze drying and hot-air drying did not affect the gelling properties of the egg white. Nevertheless, the results showed that storage conditions (temperature and time) had an effect on the gelling properties of the stored FDEW and HDEW. The hardness and %WHC of the gel samples made from FDEW after storage at 40°C for 4 months were significantly (p < 0.05) increased when compared to those stored at 25°C. However, storage of FDEW at 25°C for 4 months had no effect on the hardness of the gel samples. Substantial changes in the gelling properties were found in the gel made from the stored HDEW with the hardness and %WHC increasing with the increased storage time and temperature of the dried samples. These results were related to studies by Kato et al.[Citation18] and Mine,[Citation18] who found that the gel strength of dried egg white protein greatly increased when heated in the dry state (sample storage in a hot room under controlled temperature and moisture conditions).
The increase in the hardness value and %WHC of the gel samples might have been due to a partial conformational unfolding of the protein at high temperature related to a decrease in the ∆H of the dried egg white protein after storage as described in the following section. A protein with partially unfolded conformation as a result of high temperature during storage exhibits a greater exposure of its functional groups.[Citation18,Citation19] However, the HDEW samples stored at 40°C for 2 and 4 months were unable to form gel because the samples were insoluble in water due to the loss of protein structure during storage at high temperature[Citation20] and this might have been due to the occurrence of the Maillard reaction during storage.[Citation21] Handa and Kuroda[Citation22] who studied the effects of the Maillard reaction on the functional properties of dried egg white found a decrease in the available lysine and glucose content in the dried egg white with increasing heating storage time which indicated that the heating treatment induced the Maillard reaction in dried egg white. Thao et al.,[Citation23] who studied the Maillard reaction and protein crosslinked in relation to the solubility of milk powders stated that advanced Maillard products can react with amino acid residues to form crosslinked proteins which prevent their dispersion in water.
Protein denaturation enthalpy
The denaturation enthalpy (∆H) is correlated with the ordered secondary structure of a protein. A reduction in enthalpy is an indication of a partial loss of protein structure.[Citation24] The results () showed that the ∆H values of the stored samples of both FDEW and HDEW decreased during storage which indicated a partial unfolding of protein conformation. These results were related to changes in gelling properties of FDEW and HDEW, as described previously, increases in hardness value and %WHC of the gel samples might have been due to a partial conformational unfolding of the protein. Our findings were in line with those of Kato et al.,[Citation18] who found a good correlation between an increase in the gel strength of dried egg white and a decrease in the ∆H of dried egg white protein. Furthermore, the ∆H values of the samples were greatly decreased with increasing storage temperature and time. These results demonstrated increased in denaturation of the protein samples, which was might be related to a decrease in solubility of HDEW samples stored at 40 °C for 2 and 4 months. Van der Plancken et al.[Citation6] reported a decrease in ∆H values, an increase in surface hydrophobicity and exposure of SH groups of FDEW during storage in dry-heated stage at 80°C and stated that these changes induced the formation of insoluble aggregates as evidenced by the decrease in protein solubility with increasing dry-heating time and moisture content.
Exposed and total SH group contents
Ovalbumin, the major protein in egg white is the only egg white protein that contains four free SH groups, most of which exist in the interior of the molecule in the native state.[Citation25] Heat induced denaturation of ovalbumin results in exposure of these SH groups, accompanied by a decrease in the total SH content due to the oxidation of SH groups to disulfide bonds.[Citation26] illustrates the changes in the exposed and total SH contents of FDEW and HDEW during storage. There was no significant different (p ≥ 0.05) between the exposed SH contents of unstored FDEW and HDEW and also the total SH contents. After the FDEW and HDEW samples had been stored, the degree of exposed SH groups were significantly (p < 0.05) increased, especially at high storage temperatures (40°C). In this study, the SH content of HDEW stored at 40°C for 4 months could not be determined because the sample lost its protein structure as a result of storage at high temperature for a long time which leading to loss of its solubility in distilled water. These results suggested that the SH groups in dried egg white protein were gradually exposed to the surface of the proteins during storage as a result of unfolding protein (related to a decrease in ∆H of the sample during storage). Handa and Kuroda[Citation22] stated that this exposure of the SH groups could be contribute to the formation of strong gels with a high %WHC by forming intermolecular disulfide bonds between protein molecules. The results showed a decrease in the total SH content of both stored FDEW and HDEW when compared to unstored sample that indicated the formation of a disulfide bond in the protein samples during storage. Watanabe et al.[Citation27] and Van der Plancken et al.[Citation6] also reported that an increase in the exposed SH and a decrease in the total SH contents were more pronounced with increased dry-heating time. However, comparing the stored FDEW and HDEW, the result showed that the HDEW samples had higher exposed SH and lower total SH contents than the FDEW samples which was probably due to higher water content in the HDEW samples. At higher levels of water content in the samples, the extent of protein denaturation was greater,[Citation6] and the formation of intermolecular disulfide bonds between protein molecules was greatly increased due to the increased mobility of the protein molecules.[Citation28] These results were in agreement with the study of Van der Plancken et al.,[Citation6] who investigated the effect of moisture content during dry-heating at 80°C on physicochemical and functional properties such as the foaming properties of FDEW reported an increase in exposed SH and a decreased in the total SH content with an increased moisture content of the sample. This phenomenon was due to an increase in degree of protein unfolding with increasing moisture content of the samples. These results showed an increase in the exposed SH and a decrease in the total SH contents of dried egg white during storage, could suggest that the formation of disulfide bonds in the dried samples might occur during storage. This could be one cause that involves the changes in gelling properties of the stored dried egg white.
Figure 2. A: Exposed SH and B: Total SH contents of unstored and stored (4 months) FDEW and HDEW. Note: SH contents of HDEW stored at 40°C for 4 months could not be determined because it is not soluble. Data are shown as mean with standard deviation (n = 2). Different letters in each graph are significantly different (p < 0.05).
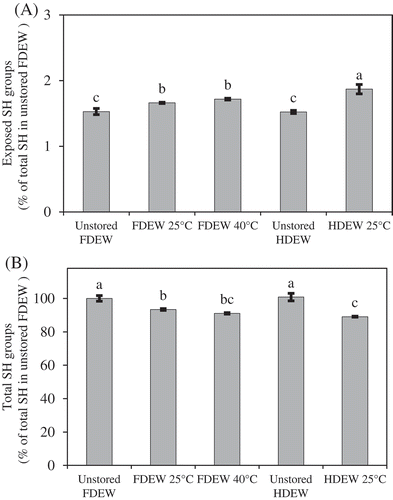
Gel electrophoresis
To better understand the changes in the gelling properties of the stored FDEW and HDEW, the protein pattern of the samples was investigated using gel electrophoresis. The SDS-PAGE patterns of fresh egg white, unstored, and stored dried egg white in the absence and presence of 2-ME are shown in , respectively. The proteins in fresh egg white, FDEW and HDEW solutions clearly separated into major bands of ovalbumin (45 kDa), ovotransferrin (76–80 kDa), and lysozyme (14 kDa). The protein patterns of FDEW remained unchanged throughout storage at 25°C for 2 months (, lanes 3 and 4). After storage of FDEW at 25°C for 4 months (lane 5), proteins with a high MW as a result of the protein aggregation from the slow migration into the SDS-gel were clearly found compared with the unstored FDEW. In the FDEW stored at 40°C, some of the aggregates were found after 1 month of storage (lane 6) and these aggregates were more pronounced after a longer storage time (lanes 7 and 8), as evidenced by their increased intensity in top of gel. This result indicated that the protein molecules in the stored sample could change and form aggregations, especially when under storage conditions at high temperature over a long time.
Figure 3. SDS-PAGE patterns of the protein samples: A: SDS-PAGE in the absence of 2-ME; B: SDS-PAGE in the presence of 2-ME; fresh egg white (lane 1), unstored FDEW (lane 2), FDEW storage at 25°C for 1, 2, and 4 months (lanes 3, 4, and 5), FDEW storage at 40°C for 1, 2, and 4 months (lanes 6, 7, and 8), unstored HDEW (lane 9), HDEW storage at 25°C for 1, 2, and 4 months (lanes 10, 11, and 12), and HDEW storage at 40°C for 1, 2, and 4 months (lanes 13, 14, and 15).
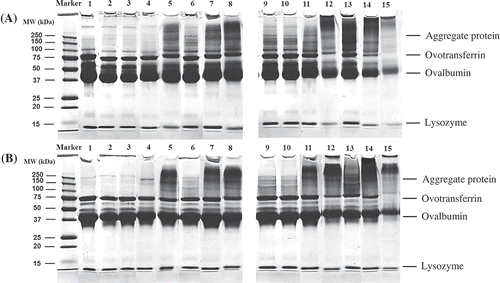
The HDEW, protein pattern was slightly different from that of FDEW, as some high MW proteins were found in the unstored sample (lane 9) which were probably due to the formation of Maillard reaction products during the drying process. Aggregation of protein was also found in HDEW stored at 25°C for 1 month (lane 10) and this gradually increased after storage for 2 and 4 months (lanes 11 and 12). The highest intensity of the aggregated protein band was observed in the protein pattern for HDEW storage at 40°C for 1 month (lane 13). Nevertheless, with increasing storage time (2 and 4 months, lanes 14 and 15, respectively), these protein bands faded (particularly at 4 months) which was probably due to a decrease in protein solubility after storage of the samples at high temperatures for a long time. This result was consistent with the observation of Van der Plancken et al.,[Citation6] who showed that the protein solubility of dried egg white progressively decreased with increased dry-heating time.
When considering the intensity of the aggregated protein band in FDEW and HDEW, the SDS-PAGE results clearly showed that the intensity of the aggregated protein band of HDEW was higher than that of FDEW which suggested that the protein aggregation during storage occurred more in HDEW than in FDEW sample. These results revealed that the water content in a sample is an important factor that can accelerate the protein aggregation in samples.
The aggregation phenomenon in such samples was thought to be due to the disulfide formation between the protein molecules during storage. Therefore, the SDS-PAGE pattern in the presence of 2-ME () which is a reducing agent which cleaves disulfide bonds between SH groups was investigated. The protein pattern of the samples in the presence of 2-ME showed a slight decrease in the proportion of aggregated protein; however, some of the aggregates remained undissociated. As some of the aggregates could not be completely dissociated in the present of 2-ME, therefore, the type of binding cannot be attributed solely to the formation of disulfide bonds during storage, tight interactions such as hydrophobic interactions between egg white proteins and a covalent bonds through Maillard reaction might contributed to the formation of the protein aggregates during sample storage. Other researchers, Kato et al.,[Citation5] Van der Plancken et al.,[Citation6] and Xu et al.[Citation29] who studied the changes in the properties of dry-heated egg white also suggested that the type of binding in the aggregate protein is likely to be hydrophobic interaction and disulfide bond formation between egg white proteins. However, Handa and Kuroda,[Citation22] who studied on the effect of the Maillard reaction on the functional properties of the dried egg white, reported that the covalent bonds through Maillard reaction other than disulfide bond were involved in the aggregate formation of the protein. Therefore, it is possible that hydrophobic interactions and Maillard reaction other than disulfide bonding contributed to the formation of the firm gel.
Glass transition temperature (Tg)
It is well-established that the Tg of a polymer is related to its MW. The Tg results in showed a significantly (p < 0.05) increase in Tg value of the stored FDEW and HDEW comparing to that of unstored samples. In addition, the Tg value of the samples stored at high temperature (40°C) were higher than that stored at low temperature (25°C). Roos[Citation30] stated that Tg values increased with increasing sample MW. Thus, this result suggests that storage the dried samples at high temperature induced changes in protein structure to aggregation more than at low temperature. These Tg results supported that during storage dried samples, the protein were partially unfolded conformation and these partially unfolded proteins might contribute to forming protein aggregates resulted in increasing in MW of the protein as previously described.
Conclusion
The present study demonstrated that a dramatically changes in the color was found in HDEW during storage. The process of freeze drying and hot-air drying did not affect the gelling properties of egg white. However, the gelling properties of dried egg white were changed especially when the samples were stored at high temperature for a long time. This study showed that storage of dried egg white at high temperature for a long time induced protein conformation changes. These have contributed to protein aggregation which affected their gelling properties. Moreover, gel made from stored HDEW was greatly increased in gel hardness and %WHC than that of stored FDEW. These indicated that water content in the sample after drying (higher water content in HDEW than FDEW) is an important parameter which accelerated changes in the properties of dried egg white.
Funding
This research was supported by the joint funding between Thailand Research Fund and Kasetsart University through The Royal Golden Jubilee Ph.D. Program (Grant No. PHD/0171/2554).
Additional information
Funding
References
- Ahmed, J.; Ramaswamy, H.S.; Alli, I.; Raghavan, V.G.S. Protein Denaturation, Rheology, and Gelation Characteristics of Radio-Frequency Heated Egg White Dispersions. International Journal of Food Properties 2007, 10, 145–161.
- Bergquist, D.H. Egg Dehydration. In Egg Science and Technology; Stadelman, W.J.; Cotterill O.J.; Eds.; Food Product Press: New York, NY, 1995; 335–376 p.
- Rawson, A.; Tiwari, B.K.; Tuohy, M.G.; O’Donnell, C.P.; Brunton, N. Effect of Ultrasound and Blanching Pretreatments on Polyacetylene and Carotenoid Content of Hot air and Freeze Dried Carrot Discs. Ultrasonics Sonochemistry 2011, 18, 1172–1179.
- Ratti, C. Hot Air and Freeze-Drying of High-Value Foods: A Review. Journal of Food Engineering 2001, 49, 311–319.
- Kato, A.; Ibrahim, H.R.; Watanabe, H.; Honma, K.; Kobayashi K. New Approach to Improve the Gelling and Surface Functional Properties of Dried Egg White by Heating in Dry State. Journal of Agricultural and Food Chemistry 1989, 37, 433–437.
- Van der Plancken, I.; Van Loey, A.; Hendrickx, M.E. Effect of Moisture Content During Dry-Heating on Selected Physicochemical and Functional Properties of Dried Egg White. Journal of Agricultural and Food Chemistry 2007, 55, 127–135.
- Rao, Q.; Rocca-Smith, J.R.; Schoenfuss, T.C.; Labuza, T.P. Accelerated Shelf-Life Testing of Quality Loss for a Commercial Hydrolysed Hen Egg White Powder. Food Chemistry 2012, 135, 464–472.
- Rao, Q.; Labuza, T.P. Effect of Moisture Content on Selected Physicochemical Properties of Two Commercial Hen Egg White Powders. Food Chemistry 2012, 132, 373–384.
- Rao, Q.; Rocca-Smith, J.R.; Labuza, T.P. Moisture-Induced Quality Changes of Hen Egg White Proteins in a Protein/Water Model. Journal of Agricultural and Food Chemistry 2012, 60, 10625–10633.
- Katekhong, W; Charoenrein, S. Changes in Physical and Gelling Properties of Freeze-Dried Egg White As a Result of Temperature and Relative Humidity. Journal of the Science of Food and Agriculture 2016, 96, 4423–4431.
- The U.S. Department of Agriculture (USDA). Egg-Grading Manual. In Agricultural Handbook No.75; Agricultural Marketing Service: Washington, DC, 2000; 34–35 p.
- Charoenrein, S.; Tatirat, O.; Muadklay, J. Use of Centrifugation–Filtration for Determination of Syneresis in Freeze–Thaw Starch Gels. Carbohydrate Polymers 2008, 73, 143–147.
- Thirathumthavorn, D.; Charoenrein, S. Thermal and Pasting Properties of Acid-Treated Rice Starches. Starch/Stärke 2005, 57, 217–222.
- Beveridge, T.; Toma, S.J.; Nakai, S. Determination of SH- and SS-Groups in Some Food Proteins using Ellman’s Reagent. Journal of Food Science 1974, 39, 49–51.
- Van der Plancken, I.; Van Loey, A.; Hendrickx, M.E. Changes in Sulhydryl Content of Egg White Proteins Due to Heat and Pressure Treatment. Journal of Agricultural and Food Chemistry 2005, 53, 5726–5733.
- Laemmli, U.K. Cleavage of Structural Proteins during the Assembly of the Head of Bacteriophage T4. Nature 1970, 227, 680–685.
- Sugino, H.; Nitoda, T.; Juneja, L.R. General Chemical Composition of Hen Eggs. In Hen Eggs: Basic and Applied Science; Yamamoto, T.; Juneja, L.R.; Hatta, H.; Kim, M.; Eds.; CRC Press: Boca Raton, FL, 1997; 13–24 p.
- Kato, A.; Ibrahim, H.R.; Watanabe, H.; Honma, K.; Kobayashi, K. Structural and Gelling Properties of Dry-Heated Egg White Protein. Journal of Agricultural and Food Chemistry 1990, 38, 32–37.
- Mine, Y. Effect of Dry Heat and Mild Alkaline Treatment on Functional Properties of Egg White Proteins. Journal of Agricultural and Food Chemistry 1997, 45, 2924–2928.
- Campbell, L.; Raikos, V.; Euston, S.R. Modification of Functional Properties of Egg White Proteins. Nahrung/Food 2003, 47, 369–376.
- Sebring, M. Desugarization of Egg Products. In Egg Science and Technology; Stadelman, W.J.; Cotterill, O.J.; Eds.; Food Product Press: New York,NY, 1995; 323–334 p.
- Handa, A.; Kuroda, N. Functional Improvements in Dried Egg White Through the Maillard Reaction. Journal of Agricultural and Food Chemistry 1999, 47, 1845–1850.
- Thao, T.; Bhandari, B.; Holland, J.W.; Deeth, H.C. Maillard Reaction and Protein Cross-Linking in Relation to the Solubility of Milk Powders. Journal of Agricultural and Food Chemistry 2011, 59, 12473–12479.
- Van der Plancken, I.; Van Loey, A.; Hendrickx, M.E. Effect of Heat-Treatment on the Physico-Chemical Properties of Egg White Proteins: A Kinetic Study. Journal of Food Engineering 2006, 75, 316–326.
- Powrie, W.D.; Nakai, S. The Chemistry of Eggs and Egg Products. In Egg Science and Technology; Cotterill. O.J.; Ed. ; AVI Publishing: New York, NY, 1986; 97–139 p.
- Rumbo, M.; Chirdo, F.G.; Fossati, C.A.; Anon, M.C. Analysis of Structure Properties and Immunochemical Reactivity of Heat-Treated Ovalbumin. Journal of Agricultural and Food Chemistry 1996, 44, 3793–3798.
- Watanabe, K.; Xu, J.Q.; Shimoyamada, M. Inhibition Effects of Egg White Dry-Heated at 120°C on Heat Aggregation and Coagulation of Egg White and Characteristics of Dry-Heated Egg White. Journal of Agricultural and Food Chemistry 1999, 47, 4083–4088.
- Zhou, P.; Labuza, T.P. Effect of Water Content on Glass Transition and Protein Aggregation of Whey Protein Powders During Short-Term Storage. Food Biophysics 2007, 2, 108–116.
- Xu, J.Q.; Shimoyamada, M.; Watanabe, K. Heat Aggregation of Dry-Heated Egg White and Its Inhibiting Effect on Heat Coagulation of Fresh Egg White. Journal of Agricultural and Food Chemistry 1998, 46, 3027–3032.
- Roos, Y. Phase Transition in Foods; Academic Press: San Diego, CA, 1995; 367 pp.