ABSTRACT
The effects of dry-curing with low concentrations of salt and sugar on the quality of rainbow trout (Oncorhynchus mykiss) fillets were studied over a 16-day storage period at 4°C. Physicochemical characteristics (total volatile base nitrogen (TVB-N), biogenic amines (BAs), ATP-related compounds and K value), microbial populations (total aerobic counts (TAC), H2S-producing bacteria, Pseudomonas, Aeromonas, and lactic acid bacteria) and sensory (SA) indicators were analyzed. Fish samples were subjected to the following treatments: untreated (control), dry-cured with 1.3% salt (T1), and dry-cured with 1.3% salt that also contained 0.9% sugar (T2). SA indicated that the sensory quality of the control, T1, and T2 was unacceptable after 8, 12, and 12 days of storage, respectively. Moreover, the cadaverine concentration of treated samples was significantly lower (P < 0.05) than the control after storing for 8 days. T1 and T2 were significantly higher (P < 0.05) than the control in inosine 5-monophosphate (IMP) content from the 2th to 10th day. Cadaverine, putrescine, hypoxanthine, and TAC were correlated significantly (P < 0.01) with SA, and these seemed to be reliable indicators of fish quality. The results indicated that curing treatments extended the shelf life of rainbow trout fillets, which could be exploited by processors.
Introduction
Rainbow trout (Oncorhynchus mykiss) is a cold-water fish species with high nutritional value, and it is a highly desirable commodity by consumers.[Citation1] The quality of rainbow trout during storage has long been a major concern to processors and consumers. Like other freshwater fish species, rainbow trout is usually more perishable than other muscle foods, because of its high moisture level, rich nutrient content, and high susceptibility to microbial activity.[Citation2,Citation3]
In recent years, numerous methods have been applied to enhance fish quality and to extend shelf life, such as high hydrostatic pressure,[Citation4] vacuum package,[Citation3] smoking,[Citation5] edible film coatings,[Citation6] and others. However, salting, which is a traditional method, is often used for preserving fish.[Citation7–Citation10] Salt can extend the shelf life of fish products by reducing water activity, controlling the reproduction of microbes, and enhancing functional properties of fish protein.[Citation11] Salt also enhances product texture and flavor. Considerable research has focused on the effect of different salt concentrations on fish quality during storage.[Citation3,Citation12–Citation14] Although salt has many advantages, the proteins may denature if the balance of protein-protein and protein-water interactions is altered at high salt concentrations.[Citation15]
In addition to salt, sugar can be also added to maintain food moisture and to promote good flavor. Moreover, sugar helps to stabilize fish myofibril protein and to contribute to the gel strength of fish gelatin.[Citation16] Some scholars have conducted research on the effect of sugar on the storage of fish. For example, experiments have included 1.8% sucrose and 1.8% salt in silver carp (Hypophthalmichthys molitrix),[Citation8] and 1% sugar in lightly salted grass carp (Ctenopharyngodon idellus).[Citation17]
Biogenic amines are basic nitrogenous compounds that are usually found at the end of storage. They are associated closely with the safety and quality of fish foods. Therefore, biogenic amines are often used to evaluate the quality of fish. Generally, the categories and contents of biogenic amines can be influenced by food composition, microbial communities, processing, and storage conditions such as temperature, storage time, and packaging.[Citation12,Citation18,Citation19] During the storage of rainbow trout, adenosine triphosphate (ATP) and its related compounds (5-diphosphate (ADP), adenosine 5-monophosphate (AMP), IMP, hypoxanthine riboside (HxR), and hypoxanthine (Hx)) have been correlated closely with the freshness of fish flesh. Because of the presence of endogenous and microbial enzymes, ATP degrades gradually according to the following process: ATP→AMP→ADP→IMP→HxR→Hx→uric acid.[Citation20] IMP is the main source of good flavor in fish flesh, but Hx leads to the deterioration of taste. Meanwhile, K value (calculated as: [(HxR+Hx) /(ATP+ADP+AMP+IMP+HxR+Hx)] × 100) is an important index to evaluate the freshness of fish.[Citation21] Therefore, we can measure the changes in ATP-related compounds to evaluate the sensory quality of fish.
In the last few years, with the variations in dietary habits, people have had a tendency to purchase convenient, high-quality, and safe food, such as fish fillets, which are seasoned with salt and sugar. Prepared fish foods are considered to be an attractive and promising alternative for traditional processing fish products. However, there is less information on how low concentrations of salt and sugar affect the changes in quality of rainbow trout. The reports on the changes in biogenic amines and ATP related compounds for lightly dry-cured fish fillets are also insufficient. Therefore, it is relevant to study the effects of low concentrations of salt and sugar on the storage of rainbow trout.
This study aimed to investigate the effects of low concentrations of salt and sugar on the changes in quality of rainbow trout fillets. To accomplish this, the physicochemical indicators (total volatile base nitrogen (TVB-N), biogenic amines (BAs), ATP-related compounds and K value), microbial load (total aerobic counts (TAC), H2S-producing bacteria, Pseudomonas and Aeromonas, lactic acid bacteria) and sensory attributes of fish fillets stored at 4°C were evaluated.
Materials and methods
Raw materials and treatment
Forty-six rainbow trout were purchased from an aquatic product wholesale market in Beijing, China, in August 2015, and transported immediately to the laboratory alive. The average weight and length of fish were 815.75 ± 90.65 g and 41.07 ± 1.07 cm, respectively. The content of crude protein, crude fat, water, and total ash of fish were 17.66 ± 1.47%, 9.42 ± 1.05%, 32.02 ± 0.63%, 1.30 ± 0.01%, respectively. Fish were euthanized by a blow to the head, scaled, gutted, filleted (each fish was divided into four pieces), and washed. After washing, fish fillets were left to drain on sterile, stainless steel wire mesh for 3 min and then prepared for dry-curing. Prior to starting this study, a preliminary experiment with different curing concentrations was performed and tested for consumer acceptability. After the taste test, it was decided that desirable taste was obtained with two concentrations (1.3% salt and 1.3% salt + 0.9% sugar).
Rainbow trout fillets were separated randomly into three groups. The untreated group was used as the control. The treated groups were dry cured with 1.3% salt (T1) and dry-cured with 1.3% salt + 0.9% sugar (T2). The addition of salt and sugar was calculated as the percentage of weight of the drained fillets. Ordinary commercial, food grade salt (China National Industry Corporation, Beijing, China) and sugar (Beijing Shijideqing Company, Beijing, China) were used for dry-curing. All samples were packed in sealed, polyvinyl chloride bags and stored at 4 ± 1°C in refrigerators until needed for analysis. We selected three fillets randomly for each group, and we took white dorsal muscle for analysis every two days (0, 2, 4, 6, 8, 10, 12, 14, and 16 days).
Sensory assessment
Assessment of the raw fish was performed by methodology recommended by Ojagh et al.[Citation6] with slight modifications (). Seven trained panelists scored the fillets for appearance, odor, texture, and morphology from 0 to 5, where 5 represented the best quality and 0 represented the lowest quality. Scores of separate attributes of the fish were summed to give an overall sensory score. A total score of 20 points was considered very fresh, and a value of 12 was seen as the borderline for acceptability.
Table 1. Standards of sensory assessment on rainbow trout fillets.
Total volatile base nitrogen
Rainbow trout fillets (10 g) were homogenized with 100 mL distilled water and stirred for 30 min, the mixture was centrifuged (3600×g, 3min), and the supernatant was collected for further use. The TVB-N was measured following the method of Song et al.[Citation22]
Microbiological analysis
Total aerobic counts (TACs) were determined following the method of Song et al.[Citation22] A 25-g sample of fish flesh was obtained aseptically and homogenized with 225 mL sterilised 0.9% physiological saline for 1 min. The homogenized samples were diluted (1:10, V/V) serially, 1 mL of serial dilution was plated onto a plate count agar, and the plates were incubated at 30°C for 72 h. Samples were measured for Pseudomonas on Pseudomonas CFC selective agar at 20°C for 2 days and for Aeromonas on Aeromonas medium base at 30°C for 2 days.[Citation18] Iron agar and MRS agar were used for H2S-producing bacteria and lactic acid bacteria, respectively. All counts were performed in duplicate.
Nucleotide degradation products
The extraction for ATP and its degradation products were based on the method described by Li et al.[Citation23] The obtained extractions were filtered through a 0.22 μm membrane filter. ATP and its related compounds were analyzed by high-performance liquid chromatography (HPLC) (LC-10AT, Shimadzu, Kyoto, Japan), which was equipped with an SPD-10AV detector (UV/Vis detector) and a COSMOSIL 5C18-PAQ column (4.6ID × 250 mm) (Nacalai Tesque, Inc., Kyoto, Japan). The mobile phase was 0.05 mol/L phosphate buffer (pH 6.8). The sample (20 μL) was injected at a flow rate of 1 mL/min, and the detection wavelength of the peak was 254 nm. The individual amounts of ATP and its related compounds were identified and quantified by comparing the retention time and spectra based on the commercially standard ATP, ADP, AMP, IMP, HxR, and Hx. K value was defined by the following equation:[Citation21]
Biogenic amines
The preparation and derivatization of BAs were performed according to the method of Zhang et al.[Citation18], with some modifications. The extractions were filtered through a 0.22 μm membrane filter and analyzed by using HPLC (LC-10AT, Shimadzu, Kyoto, Japan), which was equipped with a SPD-10AV detector (UV/Vis detector) and a COSMOSIL 5C18-PAQ column (4.6ID×250mm) (Nacalai Tesque, Inc., Kyoto, Japan). The flow rate was 1mL/min, and the column temperature was 30°C. Injection volume was 50 μL, and the peak was detected at 254 nm. Ammonium acetate (0.1M; solvent A) and acetonitrile (solvent B) were used as mobile phases. Chromatographic separation was carried out using a gradient elution as follows: 0 min, 50% B; 25 min, 90% B; 35 min, 90% B; 45 min, 50% B.
Statistical analysis
All measurements were run in triplicate (except microbiological analysis, which was carried out in duplicate). The least significant difference (LSD) procedure was used to test differences between means (significance was defined at P < 0.05) using SAS software (2008, SAS Institute Inc., Cary, NC), and analysis of variance was performed. Correlation coefficients were obtained by Pearson’s regression analysis.
Results and discussion
Sensory assessment
The sensory score of all groups showed a remarkable decline as storage time progressed (). During the first four days, there was no significant difference between the control and the treated samples (P > 0.05). Thereafter, sensory scores decreased sharply, and the score of the control was significantly lower (P < 0.05) than T1 and T2. The sensory score for T2 was also significantly higher (P < 0.05) than T1 after 6 days. Compared with the standard, the control (sensory score: 10.4) reached the unacceptable limit score on the 8th day, whereas T1 (sensory score: 15.3) and T2 (sensory score: 15.9) still maintained relatively good quality after eight days. After being stored for 12 days, quality of T1 (sensory score: 9.6) and T2 (sensory score: 11.5) became unacceptable. Similarly, Zhang et al.[Citation13] reported that the observed shelf life of common carp (Cyprinus carpio) was nine days for the untreated samples, 12 days for the samples treated with 2.0% salt, and 12 days for the samples treated with 2.0% salt + 1.0% sugar. The delay in degradation of T1 and T2 fillets in sensory characteristics may be attributed to the high osmotic pressure of salt and sugar, which inhibited enzyme activity and microbial growth in the fish flesh. T2 exhibited a slower rate of degradation than T1, which was possibly due to the addition of sugar; this addition had a further inhibitory effect on deterioration of sensory quality and gave the fish flesh a more pleasant taste.
Figure 1. Changes in sensory assessment (SA), total volatile base nitrogen (TVB-N), and total aerobic counts (TAC) in rainbow trout fillets that were treated with low concentrations of salt and sugar during storage at 4°C (A: SA; B: TVB-N; C: TAC; Control: untreated; T1: dry-cured with 1.3% salt; T2: dry-cured with 1.3% salt + 0.9% sugar).
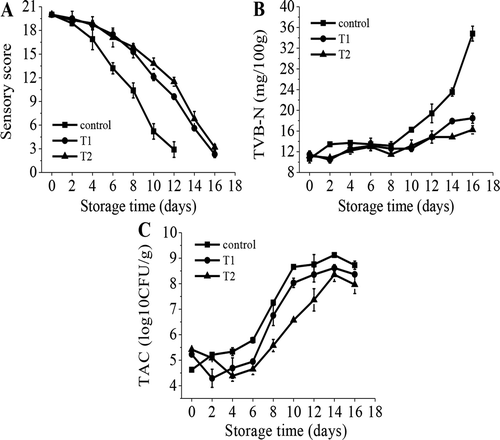
Total volatile base nitrogen
The chemical spoilage of fish fillets during storage is evaluated usually by measuring the changes in the level of TVB-N. Initial TVB-N values were not obviously different among the samples, and they ranged from 10.64 to 11.48 mg/100g (), which was similar to what was found for rainbow trout by Shen et al.[Citation20] At the beginning, the TVB-N exhibited moderate growth with subtle fluctuation. The increase in TVB-N may have been due to autolytic degradation of nucleotides and the release of free amino acids.[Citation24] The fluctuation was probably due to the reproduction of acid-forming bacteria, which can produce organic acid or CO2 to neutralize volatile basic nitrogenous compounds. Up to the 8th day, TVB-N of the control, T1, and T2 increased by 2.52, 1.12, and 0.28 mg/100g, respectively, compared with the initial value. After the 8th day, TVB-N grew rapidly, and the value of treated samples was significantly lower (P < 0.05) than the control. However, in most cases, the difference between T1 and T2 was not significant (P > 0.05). Thus, the salt was more effective at delaying the growth of TVB-N, but this effect was not obvious with sugar. On the 16th day, the concentrations of the control, T1, and T2 were 34.81, 18.48, and 16.24 mg/100g, respectively. According to Feng et al.[Citation25] and Hughes et al.[Citation26] the maximal permissible level of TVB-N is 25-35 mg/100g, and our control had almost reached this point by the 14th day, while T1 and T2 were still below this limit at day 16. Moreover, TVB-N of sugar treatment was lower than the control and T1, which may have been because sugar has an additional inhibitory effect on the increase of TVB-N. Moreover, sugar is a source of energy that facilitates the growth of certain spoilage bacteria that generate organic acid and CO2.[Citation17]
Microbial analysis
The initial values of TAC in T1 and T2 were 5.62 and 5.42 log CFU/g, respectively, which were higher than the control (4.22 log CFU/g) (); this is probably due to the curing process, which introduced microorganisms. Duman and Ozpolat[Citation27] reported very similar initial counts (4.2 log CFU/g) for TAC in controls for shibuta (Barbus grypus). However, a different initial value was reported by Shen et al.[Citation20] (3.86 log CFU/g) and Krizek et al.[Citation28] (4.99 log CFU/g) for rainbow trout and common carp, respectively. In general, water condition, temperature, and many other factors can result in a different initial microbial load in freshwater fishes.[Citation28] In the control, TAC showed a slow increase during the first four days, grew sharply thereafter, and decreased on the 16th day. TAC of T1 and T2 decreased within the first 2 days and 4 days, respectively, and then they showed trends similar to the control. TAC values of T1 were significantly lower than the control before the 10th day (P < 0.05), and T2 was significantly lower (P < 0.05) than the control and T1 before the 12th day. The reduction in the bacteria count on the 16th day may have been due to the competition between microbes and the reduction of nutrients in fish flesh. T1 and T2 reached their maximum level of 8.61 and 8.35 log CFU/g on the 14th day, respectively, which was significantly (P < 0.05) lower than the control (9.12 log CFU/g); however, there was no significant (P > 0.05) difference between T1 and T2. A comparison with the proposed limits (7 log CFU/g)[Citation29] for freshwater and marine fish showed that fillets maintained relatively good quality up to 6, 8, and 10 days for the control, T1, and T2, respectively.
H2S-producing bacteria, Pseudomonas and Aeromonas were the dominant spoilage bacteria in the control samples (). H2S-producing bacteria and Pseudomonas were also reported as the specific spoilage organisms in common carp.[Citation18] They exhibited an increasing trend and maintained a higher level than lactic acid bacteria. The value of H2S-producing bacteria, Pseudomonas, and Aeromonas, reached 8.14, 7.81, 8.00 log CFU/g on the 12th day, respectively. Lactic acid bacteria exceeded 7 log CFU/g only slightly after being stored for 12 days. Most of the time, the value of the four kinds of spoilage bacteria for T1 was significantly lower (P < 0.05) than that of the control. This phenomenon was probably due to the dehydration effect of salt,[Citation28] which affected the normal metabolism of bacteria, and it even resulted in its mortality. The value of H2S-producing bacteria for T2 was significantly lower (P < 0.05) than the other two groups after 8 days’ storage, which may have been because the sugar increased the osmotic pressure further, and it inhibited the growth of H2S-producing bacteria more strongly. However, the level of lactic acid bacteria was significantly higher (P < 0.05) than the control and T1; after 8 days, the values of the control and T2 were significantly higher (P < 0.05) than the T1. These results indicated that salt can inhibit the growth of lactic acid bacteria, and sugar facilitated the growth of it; the facilitation of growth of lactic acid bacteria by sugar surpassed the inhibition effect of salt in T2 samples.
Table 2. Changes in microbial flora (log CFU/g) of rainbow trout fillets treated with low concentration of salt and sugar during storage at 4°C.
Products of nucleotide degradation and K value
IMP is the delicate flavor compounds in fish fillets, and it is linked strongly to the acceptable level of fish freshness. However, IMP decreased as time went on (), which was different from the result (increased first, and then decreased) for white shrimp (Litopenaeus vannamei).[Citation30] Alasalvar et al.[Citation31] pointed out that the conversion of ATP to IMP is usually completed within 1–2 days in fish, and this is presumed to be a totally autolytic process. Therefore, it probably had completed the accumulation when we measured the initial value of IMP. The concentrations of IMP in all groups decreased sharply, and then they showed a slow reduction during the remainder of the storage time. Compared with the 0th day, the IMP content for the control decreased by 7.86 μmol/g, but T1 and T2 only decreased by 5.57 and 4.73 μmol/g up to the 6th day, respectively. T1 and T2 decreased rapidly until the 12th day and decreased by 8.29 and 7.91 μmol/g, respectively. IMP levels of the treated samples were significantly higher (P < 0.05) than the control from the 2nd to the 10th day. All the results indicated that curing treatments can delay the breakdown of IMP and maintain a pleasant flavor.
Figure 2. Changes in inosine monophosphate (IMP), inosine (HxR), hypoxantine (Hx), and K value in rainbow trout fillets that were treated with low concentrations of salt and sugar during storage at 4°C (A: IMP; B: HxR; C: Hx; D: K value; Control: untreated; T1: dry-cured with 1.3% salt; T2: dry-cured with 1.3% salt + 0.9% sugar).
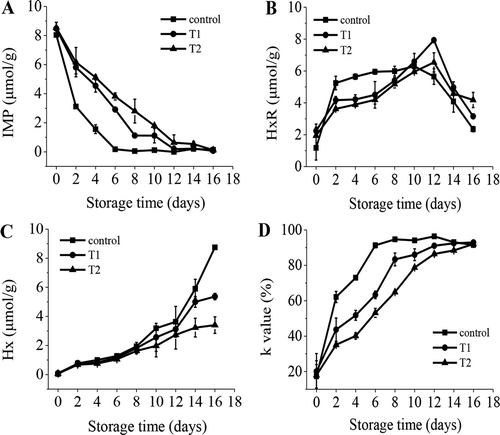
The HxR concentration increased initially and then decreased during the storage period (). From day 2 to day 8, HxR of the control was significantly higher (P < 0.05) than T1 and T2. This phenomenon indicated that the degradation of IMP of the control was more rapid than that of T1 and T2 and that the curing treatment had an obvious delay effect. Then, the control reached its peak level of 6.30 μmol/g on the 10th day, whereas T1 and T2 increased to their peak values of 7.93 and 6.54 μmol/g on the 12th day, respectively. The HxR values of the control, T1, and T2 were 2.35, 3.15, and 4.18 μmol/g, respectively, on the 16th day (P > 0.05).
Unlike IMP, Hx has a slightly bitter taste and is a contributor to the off-flavor of rainbow trout fillets. In this study, the Hx level increased gradually throughout the entire storage period (), which was consistent with the report by Shi et al.[Citation8] Compared with the initial value, Hx of the control, T1, and T2 increased by 3.59, 3.02, and 2.67 μmol/g, respectively, up to 12th day, and there was no significant differences among the three groups (P > 0.05). Thereafter, the treated samples were significantly lower (P < 0.05) than the control, and T2 was also significantly lower (P < 0.05) than T1. On day 16, the Hx values of the control, T1, and T2 were 8.75, 5.36, and 3.40 μmol/g, respectively. As Alasalvar et al.[Citation31] reported, the breakdown of IMP to Hx is possibly due to the spoilage by both endogenous autolytic enzymes in muscle and microbial enzymes. Therefore, the curing process can inhibit the activity of these two types of enzymes, and the addition of sugar can enhance this effect.
K value is one of the most important freshness indices used for the indication of nucleotide degradation and assessment of chemical spoilage.[Citation32] The initial K values of the control, T1, and T2 were 17.34%, 20.05%, and 17.97%, respectively (), which were higher than what was found in red abalone (Haliotis rufescens) (14%),[Citation26] common Carp (7%),[Citation3] and white shrimp (2.5%).[Citation30] This result might be due to the different species of fish. Changes in K values for different groups showed the same tendency to increase, in which the values grew sharply at first and then increased slowly until the end of storage. The control reached a relatively high K value (91.28%) on the 6th day, while K values for T1 and T2 were still 63.33% and 53.17%, respectively. T1 and T2 reached similarly high values of 91.08% and 92.06% on the 12th and 16th day, respectively. From the 2th day to the 12th day, T1 was significantly lower (P < 0.05) than the control, and T2 was significantly lower (P < 0.05) than T1. By comparing IMP, HxR, Hx, and K values, the lower K values of T1 and T2 were mainly because of the slower degradation of IMP. Furthermore, the delay effect in IMP degradation was probably caused by the curing treatment that changed the living conditions of microorganisms and the activity of enzymes. As Satio et al.[Citation21] described, fish products with K values that are less than 20% are very fresh, between 20% and 50% are moderately fresh, and higher than 70% are not fresh. Under this evaluation criterion, the control, T1, and T2 were very fresh on the 0th day; fish fillets were moderately fresh up to 2 days for the control, 4 days for T1 and T2, and not fresh after 4, 6, and 8 days for the control, T1, and T2, respectively. Based on the K values, fish fillets were unacceptable for the control, T1, and T2 after 4, 6, and 8 days, respectively, while the sensory scores were still in the acceptable range. Song et al.[Citation22] also reported a similar result for bream (Megalobrama amblycephala).
Biogenic amines
Biogenic amines are basic nitrogenous compounds, and they are often used to evaluate fish quality. BAs mainly contain tryptamine (TRM), 2-phenylethylamine (PHE), putrescine (PUT), cadaverine (CAD), histamine (HIM), tyramine (TYM), spermidine (SPD), and spermine (SPM). Overall, the concentrations of the biogenic amines in all samples showed an increasing trend during the storage period, except for PHE and HIM ().
Table 3. Changes in biogenic amines (BAs) concentration (mg/kg) of rainbow trout fillets treated with low concentration of salt and sugar during storage at 4°C.
PHE maintained a fluctuating, high level during the entire period. Ikonic et al.[Citation33] reported a similar result for concentrations of PHE. On the 2nd, 8th, 10th, and 14th day, the concentrations of PHE in treated samples were significantly lower (P < 0.05) than those of the control, which may due to the preservative effect of salt and sugar. HIM is the most dangerous biogenic amine from a toxicological point of view, and it receives high-profile attention. However, in our study, HIM was only detected on the 10th and 16th day for T2 and the control, respectively. Similar results were obtained in grass carp stored at 4°C and in dry-fermented sausage. [Citation33,Citation34] The concentrations of HIM were lower than its permissible limit in foods (10 mg/100 g).[Citation35] According to this standard, HIM in rainbow trout fillets was always at a safe level during the chilled storage period. Ikonic et al.[Citation33] pointed out that the lack of production of HIM might be due to the lack of decarboxylation of amino acids to form HIM in freshwater species. It might be also due to the inhibition of some species of Enterobacteriaceae that could decarboxylate histidine.
PUT and CAD are the main biogenic amines in fish, and they are used as indicators of flesh freshness or microbial spoilage. The PUT concentration in all samples grew slowly but fluctuated before the 6th day; PUT then showed an obvious increase until the 16th day, which was similar to the results of Katikou et al.[Citation36] and Rezaei et al.[Citation37] After being stored for 10 days, the PUT concentration of the treated samples was significantly lower (P < 0.05) than the control. During the entire storage period, PUT increased by 6.21, 3.58, 0.49 mg/kg for the control, T1, and T2, respectively. CAD showed a trend similar to PUT during the entire storage period. After the 8th day, the concentration of the control increased by 1.91 mg/kg, while there was little change in T1 and T2. In treated samples, CAD was often lower than the control, and this difference was significant (P < 0.05) from the 8th day to the 16th day. However, except the 10th day, there was no significant (P < 0.05) difference between T1 and T2 during the entire storage. The increase in these two biogenic amines was possibly the result of some special spoilage bacteria. Bover-Cid et al.[Citation38] noticed that the occurrence of putrescine and cadaverine in meat products was related to ornithine- and lysine-decarboxylase activity of Enterobacteriaceae. Curing treatment may have reduced the formation of PUT and CAD by inhibiting the metabolic activity of these bacteria. However, there was no evident impact of sugar on CAD. Similarly, sugar did not have an obvious impact on SPD and SPM in slightly fermented sausages.[Citation38] On the contrary, in dry-cured grass carp, the addition of sucrose decreased the concentration of these two BAs significantly (P < 0.05).[Citation39] Therefore, the effect of sugar on the level of biogenic amines in fish fillets was unclear. Further investigation needs to be done to determine how sugar affects the formation of these two BAs. Moreover, the concentrations of TRM, TYM, SPD, and SPM increased with fluctuations during the entire storage period in all fish samples. But the differences among the control, T1, and T2 were not obvious.
Relationships among SA, TAC, TVB-N, IMP, Hx, K-value, CAD, and PUT
The correlation coefficients for sensory assessment (SA), TAC, TVB-N, IMP, Hx, K-value, CAD, and PUT of rainbow trout fillets stored at 4°C are presented in . In our study, TVB-N was correlated significantly (P < 0.05) with CAD and PUT in the three treatments. SA was also correlated significantly (P < 0.01) with CAD and PUT, except for the PUT in T1. Hx was also correlated significantly (P < 0.01) with SA and TAC in all samples, which indicated that the increase in Hx induced the decrease in sensory quality and had a close relationship with the microbial load. Zhang et al.[Citation18] reported a similar result for common carp when stored at 20°C and 0°C. Moreover, SA was correlated significantly with K-value (P < 0.05) and TAC (P < 0.01) in all groups. Therefore, CAD, PUT, Hx, K-value, and TAC can be used as indicators to evaluate the sensory quality and to predict the shelf life of rainbow trout.
Table 4. Regression correlation coefficients among sensory assessment, microbial flora, TVB-N, K-value, biogenic amines in rainbow trout fillets stored at 4°C.
Conclusion
In conclusion, dry-curing with 1.3% salt and 1.3% salt + 0.9% sugar can delay the physicochemical and microbial changes, maintain sensory quality, and extend the shelf life of rainbow trout stored at 4 ± 1°C. In addition, sugar had an additional delay effect on the decrease of SA and the growth of TAC, H2S-producing bacteria, Hx, and K-value. Therefore, sugar can be added to rainbow trout to improve quality during storage. Thus, the treatment with low salt and sugar is an effective preservation method, and it is suitable for application in fish processing.
Funding
This study was supported by Beijing Natural Science Foundation (award no. 6152017), National Natural Science Foundation of China (award no. 31471683), and National Science and Technology Ministry of China (award no. 2015BAD17B03).
Additional information
Funding
References
- Cakli, S.; Kilinc, B.; Dincer, T.; Tolasa, S. Comparison of the Shelf Lifes of Map and Vacuum Packaged Hot Smoked Rainbow Trout (Onchoryncus mykiss). European Food Research and Technology 2007, 224(1), 19–26.
- Chytiri, S.; Chouliara, I.; Savvaidis, I.N.; Kontominas, M.G. Microbiological, Chemical and Sensory Assessment of Iced Whole and Filleted Aquacultured Rainbow Trout. Food Microbiology 2004, 21(2), 157–165.
- Zhang, Y.; Li, Q.; Li, D.; Liu, X.; Luo, Y. Changes in the Microbial Communities of Air-Packaged and Vacuum-Packaged Common Carp (Cyprinus carpio) Stored at 4 Degrees C. Food Microbiology 2015, 52, 197–204.
- Reyes, J.E.; Tabilo-Munizaga, G.; Perez-Won, M.; Maluenda, D.; Roco, T. Effect of High Hydrostatic Pressure (HHP) Treatments on Microbiological Shelf-Life of Chilled Chilean Jack Mackerel (Trachurus murphyi). Innovative Food Science & Emerging Technologies 2015, 29, 107–112.
- Omar Moreno-Escamilla, J.; de la Rosa, L.A.; Alberto Lopez-Diaz, J.; Rodrigo-Garcia, J.; Alberto Nunez-Gastelum, J.; Alvarez-Parrilla, E. Effect of the Smoking Process and Firewood Type in the Phytochemical Content and Antioxidant Capacity of Red Jalapefio Pepper during Its Transformation to Chipotle Pepper. Food Research International 2015, 76, 654–660.
- Ojagh, S.M.; Rezaei, M.; Razavi, S.H.; Hosseini, S.M.H. Effect of Chitosan Coatings Enriched with Cinnamon Oil on the Quality of Refrigerated Rainbow Trout. Food Chemistry 2010, 120(1), 193–198.
- Yanar, Y.; Celik, M.; Akamca, E. Effects of Brine Concentration on Shelf-Life of Hot-Smoked Tilapia (Oreochromis niloticus) Stored at 4 Degrees C. Food Chemistry 2006, 97(2), 244–247.
- Shi, C.; Cui, J.; Luo, Y.; Zhou, Z. Effect of Lightly Salt and Sucrose on Rigor Mortis Changes in Silver Carp (Hypophthalmichthys molitrix) Stored at 4 Degrees C. International Journal of Food Science and Technology 2014, 49(1), 160–167.
- Lauritzsen, K.; Akse, L.; Johansen, A.; Joensen, S.; Sorensen, N.K.; Olsen, R.L. Physical and Quality Attributes of Salted Cod (Gadus morhua L.) as Affected by the State of Rigor and Freezing Prior to Salting. Food Research International 2004, 37(7), 677–688.
- Martinez, O.; Salmeron, J.; Dolores Guillen, M.; Pin, C.; Casas, C. Physicochemical, Sensorial and Textural Characteristics of Liquid-Smoked Salmon (Salmo salar) as Affected by Salting Treatment and Sugar Addition. International Journal of Food Science and Technology 2012, 47(5), 1086–1096.
- Offer, G.; Trinick, J. On the Mechanism of Water Holding in Meat – The Swelling and Shrinking of Myofibrils. Meat Science 1983, 8(4), 245–281.
- Wang, H.; Luo, Y.; Yin, X.; Wu, H.; Bao, Y.; Hong, H. Effects of Salt Concentration on Biogenic Amine Formation and Quality Changes in Grass Carp (Ctenopharyngodon idellus) Fillets Stored at 4 and 20 Degrees C. Journal of Food Protection 2014, 77(5), 796–804.
- Zhang, Y.; Qin, N.; Luo, Y.; Shen, H. Effects of Different Concentrations of Salt and Sugar on Biogenic Amines and Quality Changes of Carp (Cyprinus carpio) during Chilled Storage. Journal of the Science of Food and Agriculture 2015, 95(6), 1157–1162.
- Rizo, A.; Fuentes, A.; Fernandez-Segovia, I.; Barat, J. M. Smoke-Flavoured Cod Obtained by a New Method using Water Vapour Permeable Bags. Journal of Food Engineering 2016, 179, 19–27.
- Thorarinsdottir, K. A.; Arason, S.; Geirsdottir, M.; Bogason, S.G.; Kristbergsson, K. Changes in Myofibrillar Proteins During Processing of Salted Cod (Gadus morhua) as Determined by Electrophoresis and Differential Scanning Calorimetry. Food Chemistry 2002, 77(3), 377–385.
- Park, J.W.; Lanier, T.C. Combined Effects of Phosphates and Sugar or Polyol on Protein Stabilization of Fish Myofibrils. Journal of Food Science 1987, 52(6), 1509–1513.
- Wang, Z.; Chen, K.; Chen, J.; Fan, H.; Luo, Y. Effect of Sugar on the Changes in Quality of Lightly Salted Grass Carp (Ctenopharyngodon idellus) Fillets under Vacuum Packaging at 4 Degrees C. Journal of Food Protection 2016, 79(3), 468–476.
- Zhang, Y.; Qin, N.; Luo, Y.; Shen, H. Changes in Biogenic Amines and ATP-Related Compounds and Their Relation to Other Quality Changes in Common Carp (Cyprinus carpio var. Jian) Stored at 20 and 0 Degrees C. Journal of Food Protection 2015, 78(9), 1699–1707.
- Shi, C.; Cui, J.; Lu, H.; Shen, H.; Luo, Y. Changes in Biogenic Amines of Silver Carp (Hypophthalmichthys molitrix) Fillets Stored at Different Temperatures and their Relation to Total Volatile Base Nitrogen, Microbiological and Sensory Score. Journal of the Science of Food and Agriculture 2012, 92(15), 3079–3084.
- Shen, S.; Jiang, Y.; Liu, X.; Luo, Y.; Gao, L. Quality Assessment of Rainbow Trout (Oncorhynchus mykiss) Fillets During Super Chilling and Chilled Storage. Journal of Food Science and Technology-Mysore 2015, 52(8), 5204–5211.
- Saito, T.; Arai, K.; Matsuyoshi, M. A New Method for Estimating the Freshness of Fish. Bull Japanese Society Science Fisheries 1959, 24(9), 749–750.
- Song, Y.; Liu, L.; Shen, H.; You, J.; Luo, Y. Effect of Sodium Alginate-Based Edible Coating Containing Different Anti-Oxidants on Quality and Shelf Life of Refrigerated Bream (Megalobrama amblycephala). Food Control 2011, 22(3–4), 608–615.
- Li, K.; Luo, Y.; Shen, H. Postmortem Changes of Crucian Carp (Carassius auratus) During Storage in Ice. International Journal of Food Properties 2015, 18(1), 205–212.
- Gill, T.A. Objective Analysis of Seafood Quality. Food Reviews International 1990, 6(4), 681–714.
- Feng, L.; Jiang, T.; Wang, Y.; Li, J. Effects of Tea Polyphenol Coating Combined with Ozone Water Washing on the Storage Quality of Black Sea Bream (Sparus macrocephalus). Food Chemistry 2012, 135(4), 2915–2921.
- Hughes, B.H.; Perkins, L.B.; Yang, T.C.; Skonberg, D.I. Impact of Post-Rigor High Pressure Processing on the Physicochemical and Microbial Shelf-Life of Cultured Red Abalone (Haliotis rufescens). Food Chemistry 2016, 194, 487–494.
- Duman, M.; Ozpolat, E. Effects of Water Extract of Propolis on Fresh Shibuta (Barbus grypus) Fillets During Chilled Storage. Food Chemistry 2015, 189, 80–85.
- Krizek, M.; Vacha, F.; Vorlova, L.; Lukasova, J.; Cupakova, S. Biogenic Amines in Vacuum-Packed and Non-Vacuum-Packed Flesh of Carp (Cyprinus carpio) Stored at Different Temperatures. Food Chemistry 2004, 88(2), 185–191.
- International Commission on Microbiological Specifications for Foods (ICMSF). Microorganisms in Foods, 2: Sampling for Microbiological Analysis: Principles and Specific Applications, 2nd ed.; Blackwell Scientific Publications: London, 1986; 181–193.
- Huang, Y.-R.; Zelaya, M.F.G.; Shiau, C.-Y. Changes in Biochemical Compositions and Quality of White Shrimp (Litopenaeus vannamei) During Storage. Journal of Aquatic Food Product Technology 2016, 25(1), 35–45.
- Alasalvar, C.; Taylor, K.D.A.; Oksuz, A.; Shahidi, F.; Alexis, M. Comparison of Freshness Quality of Cultured and Wild Sea Bass (Dicentrarchus labrax). Journal of Food Science 2002, 67(9), 3220–3226.
- Hong, H.; Regenstein. J.M; Luo, Y. The Importance of ATP-Related Compounds for the Freshness and Flavor of Post-Mortem Fish and Shellfish Muscle: A Review. Critical Reviews in Food Science and Nutrition 2015. doi:10.1080/10408398.2014.1001489
- Ikonic, P.; Tasic, T.; Petrovic, L.; Skaljac, S.; Jokanovic, M.; Mandic, A.; Ikonic, B. Proteolysis and Biogenic Amines Formation during the Ripening of Petrovska Klobasa, Traditional Dry-Fermented Sausage from Northern Serbia. Food Control 2013, 30(1), 69–75.
- Qin, N.; Li, D.; Hong, H.; Zhang, Y.; Zhu, B.; Luo, Y. Effects of Different Stunning Methods on the Flesh Quality of Grass Carp (Ctenopharyngodon idellus) Fillets Stored at 4 Degrees C. Food Chemistry 2016, 201, 131–138.
- Kim, M.K.; Mah, J.H.; Hwang, H.J. Biogenic Amine Formation and Bacterial Contribution in Fish, Squid and Shellfish. Food Chemistry 2009, 116(1), 87–95.
- Katikou, P.; Georgantelis, D.; Paleologos, E.K.; Ambrosiadis, I.; Kontominas, M. G. Relation of Biogenic Amines’ Formation with Microbiological and Sensory Attributes in Lactobacillus-Inoculated Vacuum-Packed Rainbow Trout (Oncorhynchus mykiss) Fillets. Journal of Agricultural and Food Chemistry 2006, 54(12), 4277–4283.
- Rezaei, M.; Montazeri, N.; Langrudi, H.E.; Mokhayer, B.; Parviz, M.; Nazarinia, A. The Biogenic Amines and Bacterial Changes of Farmed Rainbow Trout (Oncorhynchus mykiss) Stored in Ice. Food Chemistry 2007, 103(1), 150–154.
- Bover-Cid, S.; Izquierdo-Pulido, M.; Vidal-Carou, M.C. Changes in Biogenic Amine and Polyamine Contents in Slightly Fermented Sausages Manufactured with and without Sugar. Meat Science 2001, 57(2), 215–221.
- Zhang, J.; Liu, Z.; Hu, Y.; Fang, Z.; Chen, J.; Wu, D.; Ye, X. Effect of Sucrose on the Generation of Free Amino Acids and Biogenic Amines in Chinese Traditional Dry-Cured Fish During Processing and Storage. Journal of Food Science and Technology-Mysore 2011, 48(1), 69–75.