ABSTRACT
This study focused on the inactivation of bacterial growth using a mixture containing Salmonella enteritidis, Staphylococcus aureus, Bacillus cereus, and Escherichia coli. This bacterial mixture was added to chicken eggshells and subsequently targeted for elimination using two types of silver nanoparticle (Ag-NP) delivery systems: a composite film (named cover) and a colloidal spray. Both systems were applied to the eggshells’ packaging at concentrations of 500, 1000, and 2000 ppm over 28 days. A General Linear Model (GLM), repeated measurement-Analysis of Variance (ANOVA) procedure was used to analyse the effect of time and concentration of the Ag-NP materials on the inactivation of bacteria. The maximum reduction of bacterial growth resulted from the “Spray 2000 ppm”, for which the value of the bacteria reached a minimum (0.93 ± 0.42) on day 7, and was calculated to be undetected on days 14 and 28, followed by Spray 1000 ppm. In other words, increasing the concentration of the Ag-NP in both coatings, either spray or cover, significantly decreased the number of bacteria colonies on the eggshells. However, the effect of packaging with different concentrations of nanocomposites and the length of the study (number of days) did not show statistically significant results.
Introduction
Since 1960, the global production of animal proteins has increased more than three times, and the production of eggs has increased nearly by four times.[Citation1] Increasing worldwide population and demands of consumers have played a significant part in the growing need for animal proteins, particularly for eggs. Eggs have been touted as healthy foods, following the focus on public health in many countries.[Citation1] Eggs are composed of approximately 9.5% eggshell, 63.0% albumen, and 27.5% yolk.[Citation2]
The earth’s crust contains approximately 0.1 ppm silver ions, while soil contains approximately 0.3 ppm of silver ions.[Citation3] The Greek historian Herodotus recorded that Cyrus the Great, King of Persia (559–530 BC), had water drawn from a watercourse and “boiled it and many four-wheeled carriages drawn by mules carried it in silver containers following the king, wherever he had a visit at any time”.[Citation4] To date, silver nanoparticles (Ag-NPs) have been widely used in commercial materials, and they are increasingly used in public health and consumer products, including household disinfectant sprays, antimicrobial coatings for medical devices, and sterilization of the air and the floor.[Citation4,Citation5] The antimicrobial activity of NP food packaging is a principal feature of such metal-based nanocomposites, including Ag-NP composites, which are formed by mixing polymer films with organic or inorganic matter in particular concentrations.[Citation6–Citation8] Nanotechnology including nano film[Citation8] or nano spray[Citation4] provides a lightweight material with stronger packaging barriers that protect food quality during transportation, prolongs the freshness of fruits and vegetables during storage, and preserves meats or poultry from pathogens[Citation9] Moreover, Ahari et al.[Citation10] found a significant effect of Ag-NPs on saffron’s shelf life in commercialized conventional packaging. Similarly, Hajimirzababa et al.[Citation11] confirmed the effect of colloidal Ag-NP spray on the inactivation of bacteria on flooring.
In addition, antimicrobial packaging (AP) materials extend the bacterial lag phase and decrease the load of bacteria, which extend the shelf life and maintain the quality of products.[Citation12] Silver ions have a strong inhibitory and bactericidal effect on as many as 16 species of bacteria, such as E. coli.[Citation13,Citation14] The high performance of NPs is due to their high surface areas, which are the main reason that metal NPs, such as Ag-NPs, display rising antimicrobial activity over time.[Citation15] The materials being studied include titanium dioxide, Ag-NPs, cerium oxide, and fullerenes. To date, only a few studies have attempted to explain the effects of Ag-NPs on controlling the bacterial load of eggshells. For example, Farzinpour and Karashi[Citation16] explored the effects of NPs on egg quality, but they concentrated on egg parameters, such as weight, length, width, yolk weight, and eggshell thickness, and microbial analysis was not assessed. Ag-NP sprays could also serve as broad-spectrum fungicides and may also exhibit antiviral properties.[Citation17] Feeding bottles and mug cups developed with NP technology can help protect children with weak immunity from all sources of diseases.[Citation9]
During egg production, microorganisms can contaminate eggs at several stages. Horizontal transmission occurs when eggs are exposed to water or other liquids, especially when there is a difference between the inside and the surface temperature of the eggs. Bacteria then easily penetrate the eggshells.[Citation18] In addition, some reports have confirmed a correlation between eggshell contamination and bacterial shell penetrations.[Citation19,Citation20] In addition, the silver ions released from the surface of Ag-NPs control and cause a microorganism’s death, due to their binding to the bacterial cell’s membrane.[Citation13,Citation14,Citation21] The amount of silver migration was significantly increased by time and temperature in all food simulation solutions. Such research suggested the possible mechanism for this migration phenomenon in two steps. The first step consists of the initial release of the encapsulated Ag-NP, which was placed on the specimens’ surface layers. Then, the subsequent release of Ag-NP took place through a dual-sorption mechanism: diffusion and embedding.[Citation9] Recent microbiological studies have implied that the interaction of Ag+ with thiol groups within the cell membrane is responsible for bacterial inactivation.[Citation22] Accordingly, our study compared the effects of two types of Ag-NPs products—nanocomposite packaging cover and a packaging colloidal spray—on the prohibition of selected bacterial growth: Salmonella enteritidis, Staphylococcus aureus, Bacillus cereus, and Escherichia coli. These bacteria were placed on the surface of the chicken eggshells to assay the effects of Ag-NPs release among the bacterial groups.
Materials and methods
Bacterial suspension, measurement, and egg immersion
A bacterial suspension was made using 106 colony forming units (CFUs) ml−Citation1 of four selected bacteria prepared from Azad Islamic University (Tehran Research and Science Branch): Salmonella enteritidis (WHO-approved), Staphylococcus aureus (ATCC 6538), Bacillus cereus (PTCC 11778), and Escherichia coli (O157:H7) all in the same ratio. The stock solution, maintained at −20°C, was prepared by adding the bacterial working suspension, and a new culture was carried out using a brain–heart infusion (BHI) broth (53286 SIGMA-ALDRICH) at 37°C for 18–20 h, with a phosphate buffered solution (PBS) (Sigma-Aldrich, St. Louis, MO).[Citation23] The solution was kept in a refrigerator until required for the experiment.[Citation18,Citation24] The wavelength of the spectrophotometer (HP-8452, Hewlett-Packard, Palo Alto, CA) was adjusted to 600 nm. Before adding bacteria to the covet, which contained 4 ml of sterile BHI broth, the absorbance was set to zero. Adequate volume of bacterial suspension was transferred to each covet, the absorbance was read, and subsequently set to 0.1.
Serial dilution was performed by adding 1 ml of bacterial suspension kept in the covet to a tube containing 9 ml of sterile 0.1% peptone water. Cultures were performed by adding 100 µl of each dilution to BHI agar plates. Plates were incubated at 35°C for 24 h. After this period, the CFU values of bacteria were counted.[Citation25] Based on spectrophotometer absorbance, adjusted to 0.1, the number of bacteria per ml (density) in the bacterial suspension kept in the covet was calculated.
Egg study design
A total of 192 freshly laid chicken eggs were randomly purchased from a layer management system in Tehran, Iran. The eggs were sterilized using a rinse of 70% ethanol and subsequently exposed to ultraviolet (UV) light for 3–4 min. Ultraviolet intensities reached a maximum of 14 mW/cm following the method of Coufal et al.[Citation26] Next, the eggs were randomly distributed among eight groups in triplicate. Group 1 formed the negative control as the untreated group. Group 2 formed the positive control, where the eggs were immersed only in the bacterial suspension but not given any treatment. Groups 3–5 were immersed in the bacterial suspension and then treated with an NP colloidal spray in one of the three concentrations, 500, 1000, and 2000 ppm sprayed over 5 cm away from the eggs. The spray, which was prepared in collaboration with Veterinary Section, Pars Nano Nasb, Tehran, Iran, based on the melt mixing method of Potschke et al.[Citation27] used a sol-gel process that stabilized the suspension of colloidal solid particles within a liquid, and it was applied to the egg containers. Finally, groups 6–8 were also immersed in the bacterial suspension and subsequently were packaged with a silver nanocomposite film (named cover and prepared by veterinary section, Pars Nano Nasb, Tehran, Iran), containing the same concentrations of Ag-NPs as the colloidal spray. The polymer/silver nanocomposite film was made using the melt-mixing method. All the eggs were kept at 25°C for 28 days. The eggs of groups 2–8 were immersed in the bacterial suspension and bacterial samples of the eggshells were taken at days 0, 7, 14, and 28, after treating the eggshells of the positive groups[Citation3–Citation8] with Ag-NP spray or the Ag-NP cover, as previously discussed.
Bacterial sampling and counting
During the laboratory testing of the control and treated eggs, each egg was placed in a sterile zip bag and 30 ml sterile distilled water was added, and the bag was shaken. Next, 1 ml of the solution within the bag, containing the probable selected microorganisms, was sampled. A total count was initially prepared using serial dilution. Next, 1 ml of each dilution was cultivated using the pour plate method on a count plate agar at 37°C for 24 h. A plate containing 30–300 colonies was appropriated for counting. The number of colonies was multiplied by the dilution ratio to calculate the number of bacteria in 1 ml of the sample, and the resulting number was multiplied by 30 (30 ml sterile distilled water) to calculate the whole number of the bacteria on the eggshell.[Citation23]
Scanning Electron Microscope (SEM)
The presence of Ag-NPs within the packaging material was confirmed using a SEM (Vega, Tescan, Philadelphia, PA, USA) in which the reflected light determined the size of NPs invisible to the eye. Master batches of the constituent particles were dissolved in a solvent, acetonitrile 1v/1v,[Citation28] to make a water-based solution. These ingredients, dissolved in the acetonitrile, were inserted into two metal legs inside a device called a sputter coater. The Ag-NPs were sputter-coated with gold prior to the SEM examination. A particle-size range of 1–100 nm proved that the increased shelf life was due to the NPs formed inside the packaging materials.[Citation29–Citation31]
Titration test
Titrino (Metrohm, Herisan, Switzerland) was used to test the number of Ag-NPs attached to the packaging composite cover and the egg pack treated with the Ag-NP colloidal spray. Through the test carried out for the Ag-NPs, the whole number of the particles was tested after one month in order to determine whether the number of particles decreased or remained the same as the initial number.[Citation32]
To perform the test, each of the three different concentrations of the silver nanocomposite packaging was cut into small pieces (10 × 10 mm), individually, and the pieces were then added to a suspension of 80% sulphuric acid. That solution was poured into the Titrino device to titrate the number of silver particles. This device contained a silver chloride electrode, and the packing cover Ag-NP entered the chain without being used. Therefore, it was expected that the number of Ag-NPs in the beginning of the film usage would be equal to their numbers after performing the test, with no release of the NPs. Silver received a positive charge, and the silver chloride electrode created a positive charge and absorbed the silver particles inside the packing film.[Citation33]
Statistical analysis
All bacterial counts were expressed as log10 colony-forming units per gram (CFU/g−Citation1). The mean log10 values were calculated on the assumption of a normal log distribution. The effects of the nanocomposite cover and colloidal sprays on different days (time × nanoparticles) were analysed using the General Linear Model (GLM), repeated measurement-Analysis of Variance (ANOVA) procedure, using SPSS 18 software (version 18; SPSS Inc., Chicago, USA). The greater and lower values of, respectively, Pillai’s Trace and Wilks’ Lambda showed the effectiveness of the independent factor on bacteria inactivation, while the data were analysed at the level of α = .05. Nonparametric analysis of NP releasing scores was conducted using the Kruskal–Wallis test to determine if there were significant differences between multiple groups, followed by a pairwise comparison, using the Mann–Whitney test.
Results
Values of Pillai’s Trace (0.92) and Wilks’ Lambda (0.08) for the groups over time showed a significance of 99%, while those for Pillai’s Trace and Wilks’ Lambda time × nanoparticle group were 1.38 and 0.04, respectively, which confirmed the significant effects of the variables together (p < 0.05). The estimated marginal mean log10 values of CFU/g for the time × nanoparticle groups are tabulated in . As shown, the marginal mean log10 values of CFU/g for the negative control on days 0, 7, 14, and 28 were, respectively, 0.0 ± 0.13, 0.0 ± 0.42, 0.0 ± 0.67, and 0.0 ± 0.64, and the lack of bacteria on the eggshells was easily observed. The lower and upper bounds at a 95% confidence interval (CI) included zero, along with the groups with NP spray 1000 ppm on day 28 (0.99 ± 0.64), and NP spray 2000 ppm on days 14 (0.0 ± 0.67) and 28 (0.0 ± 0.64). The estimated marginal mean values, log10 of CFU/g, on the other sampling days did not include zero for the other groups at a 95% CI. As illustrated in , the maximum and minimum mean values for the group “Spray 500 ppm” were 4.75 ± 0.13 and 3.38 ± 0.64 on days 0 and 28, respectively. Those of the group “Cover 500 ppm” showed 4.75 ± 0.13 on day 0 and 4.65 ± 0.67 on day 14, and increased surprisingly to 4.74 ± 0.64 on day 28. The maximum and minimum CFU values for the groups with nanoparticle cover at 1000 ppm were, respectively, 4.75 ± 0.13 and 4.00 ± 0.64 on days 0 and 28, while at the same time 2000 ppm CFU values were 4.75 ± 0.13 and 2.70 ± 0.64.
Table 1. Estimated marginal means of log10 values of colony-forming units per gram (n = 9).
As shown in and , the maximum reduction of the bacterial growth was attributed to the group “Spray 2000 ppm” in which the CFU for bacteria reached a minimum of 0.93 ± 0.42 on day 7 and was calculated to be 0 on days 14 and 28. The second-most reduction in bacterial growth was achieved by the group “Spray 1000 ppm,” where the CFUs for the bacteria on days 7, 14, and 28 reached 3.65 ± 0.42, 1.95 ± 0.67, and 0.99 ± 0.64, respectively. Clearly, the CFU values did not equal 0, even at the end of the study. The third group in which the bacterial growth was reduced the most was “Cover 2000 ppm.”
Figure 1. The effect of nanoparticles on the estimated marginal (log) means on different days of the study among the eight groups using the Titrino method (χCitation2 = 4.08, p = .573).
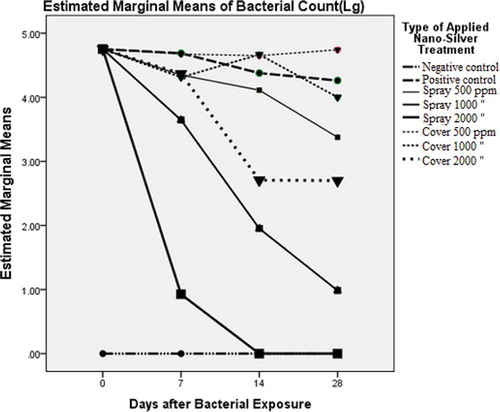
As shown in , the sizes of the NPs identified under 15 kV and 5–10 kX magnifications were all equal to 0.08–0.12 µm, which implied a mean size value of 80–120 nm for the NPs in the packaging. The accumulation of dark points in the figures could be due to the insolubility of the constituent particles in the solvent, acetonitrile (). According to the results of the Kruskal–Wallis test, there was no significant difference between the silver values of composite cover and colloidal sprays among the eight groups, using the Titrino method (χCitation2 = 4.08, p = .573).
Discussion
One of the recent strategies to improve the production, distribution, hygiene, and processing of foods is the use of nanotechnology in food industries.[Citation34] Antimicrobial studies proved the impact of NPs on bacteria reduction in contact with the Ag-NP constituents of packaging from a few minutes to 24 h incubation,[Citation15] which was in line with our study, and showed that remarkable bacteriostatic and bactericidal activities occurred after spraying with Ag-NP colloidal spray at 1000 and 2000 ppm on 7 days and on 14 and 28 days, respectively. Unlike the Ag-nanocomposite film (cover), this result showed the Ag-NP colloidal spray could effect both Gram negative and positive bacteria after 7 days up to end of the experiment. The rough structure ( and ) with more visible tiny pores and with more visible and dispersed silver particles on the surface with the potential of trapping humidity incorporated with the mixture of pathogens could result in better efficiency of Ag-NP colloidal spray versus “nanocomposite cover”. These pores can allow the structure to perform channels and to allow the chemicals and pathogens to enter the matrix in contact with nano silver to enhance the antibacteriocidal actuality. Sanchez-Valdes et al.[Citation35] confirmed that the spay method used to apply the silver nanocomposite shows tiny pores on the surface, which could increase the rate of diffusion of the chemical entities, such as water, gas, and silver ions, into the composites and enhance the microbial activity of the silver particles. They showed this effectiveness act with a smaller size of Ag-NPs (7.8–87.3 nm), which was relatively similar to the results obtained in this study (spray method). Egger et al.[Citation36] demonstrated that the silver-silica nanocomposite-containing polystyrene material had significant antibacterial activity against E. coli (<100 CFU/g) rather than S. aureus (1.4 × 1039.0 × 102 CFU/g) after 24 h. Recently, research revealed that highly reactive metal oxide MgO-NPs exhibit excellent biocidal action against Gram-positive and Gram-negative bacteria,[Citation37] and positively charged NPs were particularly crucial in bactericidal activity.[Citation14] The silver nanocomposite cover at 2000 ppm was effective in decreasing bacterial growth, but it did not stop the growth of bacteria entirely when the bacterial count was gradually reduced to 2.70 ± 0.64 log10 CFU/g. Contrary to the obtained titration values of our results, which showed no significant differences among the treatments and controls, implying an insignificant effect on bacterial reduction, Fernandez et al.[Citation21] found that the released silver ions were remarkably effective in decreasing the population of spoilage-related bacteria in cellulose-based absorbent cushions. Accordingly, the lag phases of the bacteria were significantly augmented and microbial loads in the pads remained on average approximately three log10 CFU/g−Citation1 below the control.
Accordingly, the total count of eggshell bacterial load was calculated to be 1.30 ± 0.80 log 10 CFU/g after 21 days without any treatment. In agreement with our results, the CFUs of the eggshells were enumerated to be 0.0 ± 0.67 and 0.0 ± 0.64 log 10 CFU/g after 14 and 28 days, respectively. This finding showed the effectiveness of higher Ag-NP concentrations, such as “Spray 2000 ppm,” which completely inactivated the bacteria from the eggshell on day 14. Nevertheless, the CFU/g for the groups “Spray 1000 ppm” and “Spray 500 ppm” were calculated to be 0.99 ± 0.64 and 3.38 ± 0.64, respectively, which suggests that “Spray 1000 ppm” can relatively reduce the bacterial load from the eggshell on day 28, but the “colloidal Spray 500 ppm” did not decrease the bacterial load. Spray disinfectants containing Ag-NPs might be more effective at killing bacteria than those made with larger, conventional silver particles, and this bactericidal effectiveness is due to the higher surface area-to-volume ratios of the smaller particles.[Citation4]
Our results showed that there were significant bactericidal effects from the Ag-NP spray at 1000 and 2000 ppm. In accordance with the results at a temperature of 25°C, Theron et al.[Citation38] showed no significant growth of E. coli. Sondi and Salopek-Sondi[Citation14] showed that when Ag-NPs were introduced to agar plates, they could completely inhibit E. coli growth, but when they were applied using a liquid medium, even at high concentrations, the NPs only caused a delay in the growth of E. coli. SEM results in this study implied the size of 80–120 nm as the mean value of Ag-NPs in the egg packaging. This was in agreement with our previous findings,[Citation39] which confirmed that increasing the food life-span using Ag-NP covers occurred by decreasing the size of the bactericidal materials to sizes smaller than 100 nm, by which their properties follow the quantum physics rules. In total, different dilutions of Ag-NP coatings, using either spray or cover methods, functionally decreased the bacterial CFUs, but the results of this study imply the ineffectuality of the silver nanocomposites at 500 ppm and 1000 ppm in inactivating or reducing the bacterial count on the eggshells. On the other hand, the silver nanocomposites at 2000 ppm were effective in diminishing bacterial growth, but they did not stop the growth of bacteria entirely. In other words, increasing the concentration of Ag-NP coatings, using either spray or cover, created a significant decrease in the number of bacteria colonies on the eggshells. However, the effects of packaging at various concentrations of nanocomposites and the number of days within the study showed no statistically significant results. Finally, the colloidal Ag-NP spray, particularly at concentrations of 2000 ppm, was safer and more effective in inactivating bacteria on the eggshells through day 28, than the silver nanocomposite cover.
Acknowledgments
We would like to thank both the head and the experts of the Razi Complex Laboratory of Science and Research Branch of the Islamic Azad University of Tehran, Iran, for their lab assistance and help with an earlier draft of our study.
References
- Speedy, A.W. Global Production and Consumption of Animal Source Foods. Journal of Nutrition 2003, 133(11), 4048S–4053S.
- Kovacs-Nolan, J.; Phillips, M.; Mine, Y. Advances in the Value of Eggs and Egg Components for Human Health. Journal of Agricultural and Food Chemistry 2005, 53(22), 8421–8431.
- Nanomaterials EPA is Assessing. The United States Environmental Protection Agency 2013 [cited 2015 January (13] accessed Jan 13, 2015) [Internet].
- USEPA. Nanomaterial Case Study: N l Sil i Nanoscale Silver in Disinfectant Spray. EPA: USA, 2012; 423 p.
- Xiu, Z.-M.; Zhang, Q.-B.; Puppala, H.L.; Colvin, V.L.; Alvarez, P.J.J. Negligible Particle-Specific Antibacterial Activity of Silver Nanoparticles. Nano Letter 2012, 12(8), 4271–4275.
- Alexandre, M.; Dubois, P. Polymer-layered Silicate Nanocomposites: Preparation, Properties and Uses of a New Class of Materials. Materials Science & Engineering 2000, 28(1), 1–63.
- De Azeredo, H.M.C. Nanocomposites for Food Packaging Applications. Food Research International 2009, 42(9), 1240–1253.
- Chaudhry, Q.; Scotter, M.; Blackburn, J.; Ross, B.; Boxall, A.; Castle, L.; Aitken, R.; Watkins, R. Applications and Implications of Nanotechnologies for the Food Sector. Food Additives & Contaminants 2008, 25(3), 241–258.
- Bumbudsanpharoke, N.; Ko, S. Nano Food Packaging: An Overview of Market, Migration Research, and Safety Regulations. Journal of Food Science 2015, 80(5), R910–R923.
- Ahari, H.; Anvar, A.A.; Shokri, A.; Bayat, M.; Talakesh, F.; Sadeghi, M.; Rahmannia, H. Survey of Shelf Life Effect on Iranian Saffron with Nano Packaging Snp 103.3 for Microbial Properties and Nano Particle Release. Journal of Chemical Physics 2012, 9(4), 793–802.
- Hajimirzababa, H.; Montazer, M.; Rahimi, M.K. A Survey on the Antimicrobial Effect of Nano Silver-Nylon Flooring. Medical Journal of the Islamic Azad University 2011, 21(2),101–107 (In Farsi).
- Suppakul, P.; Miltz, J.; Sonneveld, K.; Bigger, S.W. Active Packaging Technologies with an Emphasis on Antimicrobial Packaging and Its Applications. Journal of Food Science 2003, 68(2), 408–420.
- Feng, Q.; Wu, J.; Chen, G.; Cui, F.; Kim, T.; Kim, J. A Mechanistic Study of the Antibacterial Effect of Silver Ions on Escherichia coli and Staphylococcus aureus. Journal of Biomedical Materials Research 2000, 52(4), 662–668.
- Sondi, I.; Salopek-Sondi, B. Silver Nanoparticles as Antimicrobial Agent: A Case Study on E. coli as a Model for Gram-Negative Bacteria. Journal of Colloid and Interface Science 2004, 275(1), 177–182.
- Damm, C.; Neumann, M.; Münstedt, H. Properties of Nanosilver Coatings on Polymethyl Methacrylate. Soft Mater 2005, 3(2–3), 71–88.
- Farzinpour, A.; Karashi, N. In Advanced Nanomaterials and Nanotechnology: The Effects of Nano-Silver on Egg Quality Traits in Laying Japanese Quail, Springer Proceedings in Physics, vol. 143, 2013, pp. 311–319.
- Sun, R.W.-Y.; Chen, R.; Chung, N.P.-Y.; Ho, C.-M.; Lin, C.-L.S.; Che, C.-M. Silver Nanoparticles Fabricated in Hepes Buffer Exhibit Cytoprotective Activities Toward Hiv-1 Infected Cells. Chemical Communications 2005, 40, 5059–5061..
- De Reu, K.; Grijspeerdt, K.; Messens, W.; Heyndrickx, M.; Uyttendaele, M.; Debevere, J.; Herman, L. Eggshell Factors Influencing Eggshell Penetration and Whole Egg Contamination by Different Bacteria, including Salmonella enteritidis. International Journal of Food Microbiology 2006, 112(3), 253–260.
- Sauter, E.; Petersen, C. The Effect of Egg Shell Quality on Penetration by Various Salmonellae. Poultry Science 1974, 53(6), 2159–2162.
- Nascimento, V.; Solomon, S. The Transfer of Bacteria (Salmonella enteritidis) across the Eggshell Wall of Eggs Classified as “Poor” Quality. Animal Technology 1991, 42, 157–165.
- Fernández, A.; Picouet, P.; Lloret, E. Cellulose-silver Nanoparticle Hybrid Materials to Control Spoilage-Related Microflora in Absorbent Pads Located in Trays of Fresh-Cut Melon. International Journal of Food Microbiology 2010, 142(1), 222–228.
- Liau, S.; Read, D.; Pugh, W.; Furr, J.; Russell, A. Interaction of Silver Nitrate with Readily Identifiable Groups: Relationship to the Antibacterial Action of Silver Ions. Letters in Applied Microbiology 1997, 25(4), 279–283.
- De Reu, K.; Messens, W.; Heyndrickx, M.; Rodenburg, T.; Uyttendaele, M.; Herman, L. Bacterial Contamination of Table Eggs and the Influence of Housing Systems. World’s Poultry Science Journal 2008, 64(01), 5–19.
- Karimi, M.; Mehrabian, S.; Rafiei Tabatabaei, R.; Samiai, B. A Study on Microbial Properties of Mechanically Deboned Chicken Meat in Meat Plan of Tehran. Journal of Food Technology and Nutrition 2010, 73(27), 52–58.
- Sarker, S.D.; Nahar, L.; Kumarasamy, Y. Microtitre Plate-Based Antibacterial Assay Incorporating Resazurin as an Indicator of Cell Growth, and Its Application in the In Vitro Antibacterial Screening of Phytochemicals. Methods 2007, 42(4), 321–324.
- Coufal, C.D.; Chavez, C.; Knape, K.D.; Carey, J.B. Evaluation of a Method of Ultraviolet Light Sanitation of Broiler Hatching Eggs. Poultry Science 2003, 82(5), 754–759.
- Pötschke, P.; Bhattacharyya, A.R.; Janke, A.; Pegel, S.; Leonhardt, A.; Täschner, C.; Ritschel, M.; Roth, S.; Hornbostel, B.; Cech, J. Melt Mixing as Method to Disperse Carbon Nanotubes into Thermoplastic Polymers. Fullerenes, Nanotubes and Carbon Nanostructures 2005, 13(S1), 211–224.
- Jeon, H.u.; Lee, D.H.; Choi, D.J.; Kim, M.S.; Kim, J.H.; Jeong, H.M. Characteristics of Rubber/Sodium Montmorillonite Nanocomposites Prepared by a Novel Method. Journal of Macromolecular Science Part B 2007, 46(6), 1151–1163.
- Thakur, V.K.; SinGha, A.S. Biomass-Based Biocomposites; Smithers Rapra Technology: UK, 2013; 410 pp.
- De Moura, M.R.; Mattoso, L.H.; Zucolotto, V. Development of Cellulose-Based Bactericidal Nanocomposites Containing Silver Nanoparticles and Their Use as Active Food Packaging. Journal of Food Engineering 2012, 109(3), 520–524.
- Sharma, V.K.; Yngard, R.A.; Lin, Y. Silver Nanoparticles: Green Synthesis and Their Antimicrobial Activities. Advances in Colloid and Interface Science 2009, 145(1), 83–96.
- Kogure, K.; Akita, H.; Harashima, H. Multifunctional Envelope-Type Nano Device for Non-Viral Gene Delivery: Concept and Application of Programmed Packaging. Journal of Controlled Release 2007, 122(3), 246–251.
- Moaddab, S.; Ahari, H.; Shahbazzadeh, D.; Motallebi, A.A.; Anvar, A.A.; Rahman-Nya, J.; Shokrgozar, M.R. Toxicity Study of Nanosilver (Nanocid) on Osteoblast Cancer Cell Line. International Nano Letters. 2011, 1, 11–16.
- Joseph, T.; Morrison, M. Nanotechnology in Agriculture and Food: A Nanoforum Report. Nanoforum. org., 2006.
- Sánchez‐Valdes, S.; Ortega‐Ortiz, H.; Ramos‐de Valle, L.; Medellín‐Rodríguez, F.; Guedea‐Miranda, R. Mechanical and Antimicrobial Properties of Multilayer Films with a Polyethylene/Silver Nanocomposite Layer. Journal of Applied Polymer Science 2009, 111(2), 953–962.
- Egger, S.; Lehmann, R.P.; Height, M.J.; Loessner, M.J.; Schuppler, M. Antimicrobial Properties of a Novel Silver-Silica Nanocomposite Material. Applied and Environmental Microbiology 2009, 75(9), 2973–2976.
- Stoimenov, P.K.; Klinger, R.L.; Marchin, G.L.; Klabunde, K.J. Metal Oxide Nanoparticles as Bactericidal Agents. Langmuir 2002, 18(17), 6679–6686.
- Theron, H.; Venter, P.; Lues, J. Bacterial Growth on Chicken Eggs in Various Storage Environments. Food Research International 2003, 36(9), 969–975.
- Ahari, H.; Dastmalchi, F.; Ghezelloo, Y.; Paykan, R.; Fotovat, M.; Rahmannya, J. The Application of Silver Nano-Particles to the Reduction of Bacterial Contamination in Poultry and Animal Production. Food-Manufacturing-Efficiency 2008, 2(1), 49–53.