ABSTRACT
Partitioning behaviors of trypsin from the spleen of albacore tuna (Thunnus alalunga) in an aqueous two-phase system (ATPS) were investigated. Partitioning behaviors of proteins were influenced by polyethylene glycol (PEG) molecular mass and concentration, types and concentration of salts, NaCl concentration, and temperature. Trypsin was preferentially partitioned into the PEG-rich top phase. The best ATPS conditions for trypsin partitioning from albacore tuna spleen were 15% PEG 4000–15% NaH2PO4 at 40°C without the addition of NaCl, which increased the purity by 5.54-fold with the recovered activity of 71.92%. Based on SDS-PAGE, the enzyme after ATPS separation was near homogeneity and the result of SDS-substrate gel electrophoresis revealed that the band intensity of enzyme in ATPS fraction increased, indicating the enhanced specific activity of splenic extract. The study further investigated the effect of fractionated trypsin on the hydrolysis of Pacific white shrimp (Litopenaeus vannamei) shells. Electrophoretic study revealed that trypsin after ATPS separation was a potential enzyme for extraction of carotenoprotein from Pacific white shrimp shell waste. Therefore, ATPS was an effective method for purification and recovery of trypsin from the spleen of albacore tuna and it could be used as an alternative cheap proteinase for the extraction of carotenoprotein from shrimp shells.
Introduction
Albacore tuna (Thunnus alalunga) has a high commercial value and served as an important raw material for canned tuna in Thailand.[Citation1] During canned tuna processing, large quantities of viscera are generated and are known to be a rich source of digestive enzymes, especially proteinases.[Citation2] High proteinase activity of fish viscera enzymes may be interesting for many industrial applications, e.g. in the detergent, food, pharmaceutical, leather, and silk industries.[Citation1,Citation3] A variety of digestive proteolytic enzymes have been isolated from the internal organs of fish. One of the main serine proteinases detected in fish viscera is trypsin.[Citation4] Based on our previous study, albacore tuna spleen contained high proteolytic activity, which was identified as trypsin-like serine proteinase.[Citation5]
Trypsin (EC 3.4.21.4) is a serine endopeptidase that specifically hydrolyzes peptide bonds at the carboxyl terminus of lysine and arginine residues.[Citation6] It plays a major role in biological processes, including digestion and activation of zymogens of chymotrypsin and other enzymes.[Citation7] Trypsins have been used increasingly because they have shown higher catalytic activities over a wide range of pH and temperature conditions at relatively low concentrations.[Citation8] Fish trypsin has been used for preparation of protein hydrolysate[Citation2] and extraction of collagen[Citation3] and gelatin.[Citation3] Recently, bluefish trypsin has been successfully used for the extraction of carotenoprotein from crustacean processing discards.[Citation9]
Hence, an increasing large number of highly pure trypsins are needed due to their wide range of industrial and scientific uses. Trypsins have been purified and characterized from the viscera of several fish species such as bigeye snapper (Priacanthus macracanthus),[Citation10] Pacific cod (Gadus macrocephalus), and saffron cod (Eleginus gracilis).[Citation11] However, traditional methods for trypsin purification involve several steps, including ammonium sulfate precipitation, chromatography, dialysis, and filtration, which increase the cost of the processes and reduce the yield.[Citation6] Thus, it is desirable to develop new methods that improve or replace totally or partially any stages of the current purification procedures.
Recently, the efficient and economical downstream processes for the partitioning and purification of biomolecules that give high yield and high purity of the product have been demanded by industries. Partitioning in an aqueous two-phase system (ATPS) has shown to be efficient for separating and purifying proteins.[Citation12] ATPS is formed when combinations of hydrophilic solutes (polymer or polymer and certain salts) display incompatibility in aqueous solution above critical concentrations.[Citation13] ATPS has been applied for the partitioning and recovery of various proteases such as bromelain,[Citation14] trypsin,[Citation15] and alkaline proteinase.[Citation16] ATPS offers many advantages: a biocompatible environment, low interfacial tension, low energy, ease of scale-up, and continuous operations.[Citation17] Moreover, ATPS can remove contaminants, such as nucleic acids and undesirable proteins. Therefore, the aims of this study were to investigate the feasibility of utilizing ATPS for purification and recovery of albacore tuna spleen trypsin and to study the impact of fractionated trypsin from albacore tuna spleen on the hydrolysis of Pacific white shrimp shells.
Materials and methods
Chemicals
Polyethylene glycol (PEG) 1000, 4000, Nα-benzoyl-DL-arginine-p-nitroanilide hydrochloride (BAPNA), β-mercaptoethanol (βME), N,N,N′,N′-tetramethyl ethylenediamine (TEMED), wide range molecular weight markers, and Coomassie Brilliant Blue R-250 were purchased from Sigma Chemical Co. (St. Louis, MO, USA.). Tris(hydroxymethyl)aminomethane and sodium dodecyl sulfate (SDS) were obtained from Merck (Darmstadt, Germany). The salts and other chemicals with the analytical grade were procured from Merck (Darmstadt, Germany).
Fish sample preparation
Internal organs from albacore tuna (Thunnus alalunga) were obtained from Tropical Canning (Thailand) Public Co. Ltd., Hat Yai, Songkhla. Those samples (5 kg) were packed in polyethylene bags, kept in ice and transported to the Department of Food Science and Technology, Thaksin University, Phatthalung, within 2 h. Pooled internal organs were then excised and separated into individual organs. Only spleen was collected, immediately frozen, and stored at −20°C until use.
Preparation of spleen extracts
Frozen spleens were thawed using running water (26°C–28°C) until the core temperature reached −2°C to 0°C. The samples were cut into pieces with a thickness of 1–1.5 cm. Samples were homogenized in 3 vol. of acetone at −20°C for 30 min according to the method of Klomklao et al.[Citation7] The homogenate was filtered in vacuo on Whatman No. 4 filter paper. The residue obtained was then homogenized in 2 vol. of acetone at −20°C for 30 min, and then the residue was air-dried at room temperature until dry and free of acetone odor.
To prepare the splenic extract, the spleen powder was suspended in 50 mM sodium phosphate buffer, pH 7.0 containing 1.25 M NaCl and 2.0% Brij 35 at a ratio of 1:9 (w/v) and stirred continuously at 4°C for 30 min. The suspension was centrifuged for 30 min at 4°C at 5000×g using a Sorvall Model RC-B plus centrifuge (Newtown, CT, USA) to remove the tissue debris. The supernatant was collected and referred to as “splenic extract”.
Preparation of an aqueous two-phase system
ATPS was prepared in 15-ml centrifuge tubes by adding the appropriate amount of PEG, salts and splenic extract according to the method of Klomklao et al.[Citation18]
Effect of salts on partitioning of trypsin in splenic extracts
To study the effect of salts on trypsin partitioning from albacore tuna splenic extract using ATPS, different salts including NaH2PO4, K2HPO4, MgSO4, Na3C6H5O7 and (NH4)2SO4 at different concentrations (10%, 15%, and 20%, w/w) were mixed with 20% PEG1000 in aqueous system. One gram of splenic extract was added into the system. Distilled water was used to adjust the system to obtain the final weight of 5 g. The mixtures were mixed continuously for 5 min using a Vortex mixer (Vortex-genie2, G-560E, USA). Phase separation was achieved by centrifugation for 5 min at 5000×g. The top phase was carefully separated using a Pasteur pipette and the interface of each tube was discarded. Volumes of the separated phases, top and bottom phases, were measured. Aliquots from each phase were taken for enzyme assay and protein determination. The specific trypsin activity in the aqueous two-phase system was defined as:
The purification factor as:
where SAe is the SA of each phase and SAi is the initial SA of the splenic extract. The partition coefficient of protein concentration was defined as:
where CT and CB are concentrations of protein in top and bottom phase, respectively. The partition coefficient of trypsin was defined as:
where ET and EB are activity of trypsin in top and bottom phase, respectively. The volume ratio as
where VT and VB are top and bottom phase volume, respectively. The trypsin activity recovery yield was defined as:
where AT is the total trypsin activity in top phase and Ai is the initial trypsin activity of splenic extract. Based on purity and recovery yield, the appropriate salt in ATPS rendering the most effective partitioning was selected for further study.
Effect of molecular weight and concentration of PEG on partitioning of trypsin in splenic extracts
To study the effect of the concentrations (10%, 15%, 20%, and 25%, w/w) of PEG1000 and PEG4000 on trypsin partitioning in albacore tuna splenic extract, NaH2PO4 at a level of 15% was used in the system. Partitioning was performed as previously described. Based on purity and recovery yield, the ATPS rendering the most effective partitioning was chosen for study the effect of temperature on trypsin partitioning.
Effect of temperature on partitioning of trypsin in splenic extracts
The effect of temperature on the ATPS consisting of 15% PEG4000 and 15% NaH2PO4 was evaluated. One gram of albacore tuna splenic extract was added into the system. The mixtures were incubated at different temperatures (room temperature, 30°C, 40°C, 50°C, and 60°C) for 10 min in a temperature-controlled water-bath (Memmert, Schwabach, Germany). Partitioning was performed as previously described. The system showing the highest purity and recovery yield was selected for study the effect of NaCl on trypsin partitioning.
Effect of NaCl on partitioning of trypsin in splenic extracts
The phase system containing 15% PEG4000 and 15% NaH2PO4 at 40°C was chosen for study on the effect of NaCl on trypsin partitioning. Adjustment of salt content in the system was made by addition of NaCl (solid form) into the system to obtain concentrations of 0%, 2%, 4%, and 6% (w/w). Partitioning was performed as previously described. The ATPS rendering the most effective partitioning was chosen. Phase with high specific activity was dialyzed against 10 volumes of 50 mM Tris-HCl, pH 7.5 for 18 h with three changes of buffer in the first 3 h and five changes in the last 15 h. After dialysis, the samples were determined for trypsin activity and total protein content. Yield, SA, and PF were calculated. ATPS fraction with the highest purity and yield was used for hydrolysis study.
Enzyme assay and protein determination
Trypsin activity was measured by the method of Klomklao et al.[Citation6] using BAPNA as a substrate. To initiate the reaction, 200 μl of splenic extract were added into reaction mixtures containing 800 μL of 0.5 mM BAPNA in 0.1 M glycine–NaOH buffer, pH 9.5 and 200 μL of distilled water (pre-warmed to 55°C). The mixture was incubated at 55°C for precisely 15 min. The enzymatic reaction was terminated by adding 900 μl of 30% (v/v) acetic acid. The reaction mixture was centrifuged at 8000×g for 3 min at room temperature (Microlitre Centrifuge, Hettich Zentrifugen, Germany). Activity of trypsin was measured by the absorbance at 410 nm due to p-nitroaniline released. One unit of trypsin activity was defined as the amount causing an increase of 0.01 in absorbance per minute. Protein concentration was measured by the method of Bradford[Citation19] using bovine serum albumin as a standard.
Characterization of recovered trypsin
Sodium dodecyl sulfate polyacrylamide gel electrophoresis (SDS-PAGE) and substrate-gel electrophoresis
SDS-PAGE of splenic extract and their selected ATPS fractions was performed according to the method of Laemmli.[Citation20] Protein solutions were mixed at 1:1 (v/v) ratio with the SDS-PAGE sample buffer (0.125 M Tris-HCl (pH 6.8), 4% SDS, 20% glycerol and 10% βME) and boiled for 3 min. The samples (20 μg) were loaded on the gel made of 4% stacking and 12.5% separating gels and subjected to electrophoresis at a constant current of 15 mA per gel using a Mini-Protean II cell apparatus (Bio-Rad, Hercules, CA, USA). After electrophoresis, the gels were stained with 0.2% Coomassie Brilliant Blue R-250 in 45% methanol and 10% acetic acid and destained with 30% methanol and 10% acetic acid.
Substrate-gel electrophoresis was performed after electrophoresis. The gels were immersed in 100 mL of 50 mM Tris-HCl buffer, pH 7.5, containing 2% (w/v) casein for 1 h at 0°C with a gentle agitation. Thereafter, the gels were transferred to 2% (w/v) casein in 50 mM glycine–NaOH buffer. The mixture was incubated at pH 9.5 (55°C) for 15 min with continuous agitation. The gels were rinsed with distilled water and then stained with 0.05% Coomassie Blue R-250 in 15% methanol and 5% acetic acid. Thereafter, gels were destained in 30% methanol and 10% acetic acid. Proteolytic activity was indicated by the development of a clear zone on a blue background.
Hydrolysis of Pacific white shrimp shells using fractionated trypsin
Pacific white shrimp shells (Litopenaeus vannamei) were obtained from Sea Wealth Frozen Food Co., Ltd., Songkhla. Samples were packed in polyethylene bags, kept in ice with a solid/ice ratio of 1:2 (w/v) and transported to the Department of Food Science and Technology, Thaksin University, Phatthalung within 2 h. The samples were then ground to obtain the particle size of 1.0–2.0 mm.
Ground shrimp shells (5 g) were blended with three volumes of 0.1 M glycine–NaOH buffer, pH 9.5 using a Waring blender. The mixture was added with 0, 5, 10, 15, 20, 25, and 30 units of fractionated trypsin from the top phase of the ATPS (15% NaH2PO4–15% PEG4000, 40°C). The mixtures were shaken continuously at 55°C for 30 min. A control study was performed in the same manner without incubating the mixture at 55°C and addition of the enzyme. The reaction was terminated by adding solution containing 2% SDS, 8M urea, and 2% βME. The mixture was further incubated at 85°C for 1 h to solubilize total proteins. The solution was centrifuged at 5000×g for 10 min at room temperature (Sorvall Model RC-B Plus centrifuge, Newtown, CT, USA) to remove the debris. The supernatant was then subjected to SDS-PAGE analysis.
Statistical analysis
A completely randomized design was used throughout this study. All data were subjected to analysis of variance (ANOVA) and differences between means were evaluated by Duncan’s multiple range test.[Citation21] Statistical analysis was performed using the Statistical Package for Social Science (SPSS for Windows, SPSS Inc., Chicago, IL, USA).
Results and discussion
Use of ATPS for partitioning of trypsin
Effect of salts with different types and concentrations on partitioning of trypsin in ATPS
Salts are frequently used in ATPS to improve partitioning target molecules between the phase.[Citation22] The partitioning of trypsin from albacore tuna spleen was carried out in several biphasis systems of 20% PEG1000 containing different salts, including NaH2PO4, K2HPO4, MgSO4, Na3C6H5O7, and (NH4)2SO4 at various concentrations (). After phase separation, two phases were obtained, PEG-rich top phase and salt-rich lower phase. However, no phase separation was observed in the system containing 10% NaH2PO4 and 10% MgSO4 for partitioning of trypsin from albacore tuna spleen. This indicates that phase separation will occur if the concentrations of polymers or salts are above a certain critical value of the bimodal curve.[Citation22]
Table 1. Effect of phase composition in PEG1000–salt ATPS on partitioning of trypsin from albacore tuna spleen.
For all ATPS studied, the trypsin was partitioned mainly in the PEG-rich top phase, principally those with hydrophobic properties.[Citation23] Therefore, the trypsin recovery from the opposite phase (lower phase) was relatively low. The SA, PF, and yield of trypsin obtained in PEG1000-salt systems depended on the types of salt used. ATPS containing 15% NaH2PO4 showed the highest yield (85.96%) and fraction obtained had a high SA of 86.28 units/mg protein and a PF of 1.79-fold. It was noted that a phase system containing 20% PEG1000 and 20% (NH4)2SO4 gave the highest SA (106.98 units/mg protein) and PF (2.22-fold). However, the yield was generally lower than that of the system containing NaH2PO4. Amongst all systems used, the systems containing PEG1000–Na3C6H5O7 rendered the lowest yield.
The distribution of the protein and trypsin in ATPS is investigated by Kp and KE, respectively (). From the results, Kp and KE were found to vary with type and concentration of salts used. In general, Kp and KE increased with increasing salt concentration (). This phenomenon can be explained by the salting out effect by which both trypsin and impurity proteins were more easily partitioned to the PEG-rich top phase. The shift of trypsin as well as the non-desired proteins in albacore tuna splenic extract to the top phase when raising the salt concentration is probably due to the similar hydrophobic properties on their surface.[Citation24] Generally, lower Kp indicates a shift of contaminant proteins, nucleic acid and other undesirable components to the lower phase.[Citation22] When a single component must be extracted from a mixture, phase system compositions are often manipulated in such a manner that the component partitions into one of the phases, while the other components of the mixture partition into the other phase.[Citation23] Johansson[Citation25] reported that the partition of a protein is influenced by the presence of salts. This effect increases with the net charge of the protein. The presence of salt may affect the partitioning in two ways: (1) the weakening or strengthening of the interactions, or (2) the interaction between ionized groups with the opposite net charge of proteins. It has been reported that molecular weight, shape, surface hydrophobicity and specific binding sites of biological materials also influence the partition profiles.[Citation26]
VR was decreased when the concentration of salt increased. Enhancement of salt quantity provided the higher proportion of salt rich bottom phase leading to practically reduced VR.[Citation27] Rawdkuen et al.[Citation22] reported that the phase volume ratio (VR) of PEG1000-salt systems on partitioning of proteinase from Calotropis procera latex was generally decreased when salt concentration increased. The same result was also found in the extraction of bromelain from pineapple peel (Nang Lae cultv.) using aqueous two phase system (ATPS).[Citation27]
The effect of salt concentration on the recovery of trypsin from albacore tuna spleen was also investigated. The trypsin activity decreased with increasing salt concentration leading to a poor yield recovery (). This result can be rationalized by considering the negative effect of using high concentration of salt. Salts at high concentration possibly competed with enzyme in water binding, resulting in stronger protein–protein interaction, which was probably associated with precipitation.[Citation6] Hence, the type of salt and concentration used were critical factors for recovery and partitioning of trypsin from albacore tuna spleen in ATPS. Nalinanon et al.[Citation1] found that the presence of high concentrations of salts in the reaction medium greatly decreased the yield and the selectivity of the proteinase from albacore tuna stomach. Activity of trypsin from hybrid catfish viscera decreased with increasing salt concentration led to a poor yield recovery.[Citation6] Pan et al.[Citation28] also reported that increasing NaH2PO4 concentration resulted in a less activity recovery and a poorer specific activity. In view of industrial application, the highest recovery yield was attractive for further studies. Therefore, the system containing 15% NaH2PO4 was chosen for further study on the effect of PEG concentration on trypsin partitioning.
Effect of PEG with different MW and concentration on partitioning of trypsin in ATPS
shows trypsin partitioning in splenic extract from albacore tuna using ATPS containing PEG with varying molecular weights and concentrations in the presence of 15% NaH2PO4. All trypsin partitioned into the top phase in ATPS containing both PEG1000 and 4000 (KE > 1). However, the system containing PEG4000 showed higher KE than those with PEG1000. When the same PEG was used, KE increased when increasing PEG concentration. This was a result from the reduction of trypsin partitioning to the salt-rich bottom phase. For the Kp value, at the same PEG concentration, KP decreased with increasing PEG molecular weight. The lowest Kp (0.77–0.78) was found in ATPS consist of 20% PEG4000–15% NaH2PO4 and 15% PEG4000–15% NaH2PO4. The lowest Kp indicates a shift of contaminant proteins, nucleic acids, and other undesirable components to the lower phase. The results suggested that the differences in partition parameters can be attributed to the effect of molecular weight and concentration of PEG. VR of the system ranged from 1.14 to 1.61. Most of the systems yielded VR higher than 1.0, indicating that the volume fraction was distributed in the top phase rather than in the bottom phase.
Table 2. Effect of PEG molecular mass and concentration in PEG–NaH2PO4 ATPS on partitioning of trypsin from albacore tuna spleen.
From the results, PEG 4000 is more suitable for trypsin partitioning in splenic extract than PEG1000 as indicated by the higher SA and PF. However, PEG1000 exhibited slightly higher activity recovery. These results were in agreement with Klomklao et al.,[Citation6] who found that systems containing PEG4000 gave a higher SA of trypsin from hybrid catfish viscera than those containing PEG1000. Nevertheless, it is well known from studies of protein purification that the partition coefficients of proteins usually increase as the molecular weight of the PEG decreased.[Citation22] This partitioning behavior is governed by a combination of factors such as the exclusion effect of the polymer from the protein domain and the forces involved in the polymer–polymer interaction. Reh et al.[Citation23] reported that most proteins were partitioned to the top phase in phase systems with low molecular weight PEG. Consequently, factors other than the partition coefficients of the enzymes must play significant roles in the selective partitioning of the enzyme. Protein partitioning behavior was shown to be sensitive to surface hydrophobicity.[Citation22] A high hydrophobic property of a biomolecule is a factor that favors the partition equilibrium displacement to the PEG-rich phase.[Citation22] Hence, most trypsin from albacore tuna spleen partitioned in the top phase when PEG4000 was used might be due to its high hydrophobic characteristic. ATPS of 15% PEG4000–15% NaH2PO4 gave the highest PF (3.15-fold) with 80.44% yield for trypsin partitioning in splenic extract from albacore tuna. This phase system exhibited the highest PF with appropriate the recovery yield for splenic extract was chosen for further study.
Effect of temperature on partitioning of trypsin in ATPS
Temperature is an important parameter that affects the enzyme configuration and overall stability.[Citation29] shows the effect of temperature on partitioning behavior of trypsin from albacore tuna spleen using 15% PEG4000 and 15% NaH2PO4. The PF increased with an increase in the temperature and reached an optimum at 40°C. At 40°C, the highest PF (5.48-fold) of trypsin was obtained. The result was in accordance with Show et al.,[Citation30] who reported that PF of lipase from Burkholderia cepacia was increased when increasing the system temperature. Nevertheless, a decrease in PF and yield at higher temperatures (above 40°C) was found. From the results, it was suggested that temperature is an important factor in trypsin partitioning. Low temperature can cause inactivation of biomolecule.[Citation30] However, an extremely high temperature would denature the enzyme. Moreover, as the temperature increases, the PEG structure becomes more extended and as a result its preferential interaction with the protein decreases thereby decreasing the partition coefficient.[Citation29]
Figure 1. Effect of temperature on the purification factor (a) and the recovery (b) of trypsin partitioning in 15% PEG4000–15% NaH2PO4. Bars represented the standard deviation from triplicate determinations. Different letters within the same parameter indicate the significant differences (P < 0.05).
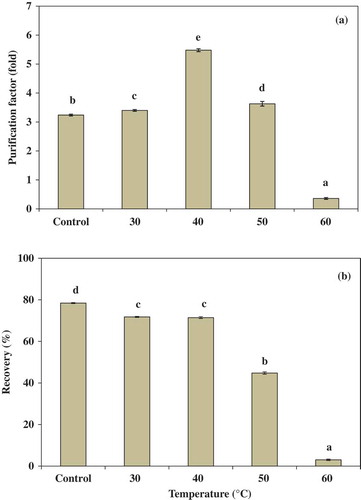
Effect of NaCl on partitioning of trypsin in ATPS
Partitioning of trypsin from albacore tuna spleen in the presence of NaCl was also studied in the system of 15% PEG4000–15% NaH2PO4 at 40°C (). In general, it is well known that NaCl is able to modify the enzyme partition in favor of one of the phases.[Citation31] Addition of NaCl to the ATPS results in an increase in the hydrophobic difference due to generation of an electrical potential difference between two phases.[Citation32] The result showed that purification factor (PF) and yield decreased with increasing NaCl concentration (). The PF of trypsin decreased from 5.54 (no NaCl addition) to 2.10 (6%, w/w NaCl). The recovery yield of trypsin decreased from 71.92 (no NaCl addition) to 47.55 (6%, w/w NaCl). Occurrence of this effect may be due to a decrease in protein solubility as a result of the addition of NaCl. Salts can change the structure of water, which acts with hydrophilic enzymes to modify the interaction between the water structures.[Citation33] Higher concentration of NaCl shows a significantly negative effect on partitioning and yield of the enzyme, probably due to protein denaturation and precipitation of this salt.[Citation34] These results were in agreement with Ketnawa et al.[Citation34] who studied the effect of NaCl concentration on partitioning of alkaline protease from Giant catfish (Pangasianodon gigas) viscera by aqueous two phase system. The results showed that the partition coefficient of the enzyme was significantly decreased from 15.34 (no NaCl addition) to 10.67 (7%, w/w NaCl).
Figure 2. Effect of NaCl concentration on the purification factor (a) and the recovery, (b) of trypsin partitioning in 15% PEG4000–15% NaH2PO4 at 40°C. Bars represented the standard deviation from triplicate determinations. Different letters within the same parameter indicate the significant differences (P < 0.05).
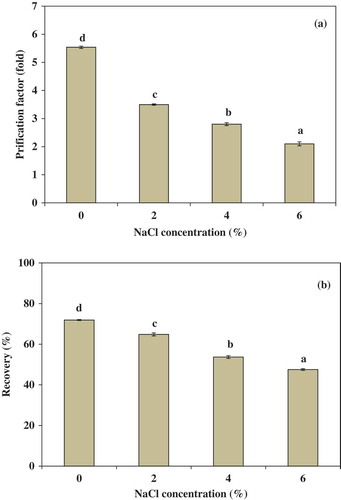
Purification of trypsin from albacore tuna spleen
The purification summary of trypsin from albacore tuna spleen is shown in . The purity of trypsin was increased by 5.54-fold to homogeneity with a recovery of 71.92% by ATPS process using phase composition of 15% PEG4000 and 15% NaH2PO4 at 40°C. Most of the steps of extraction reported for trypsin purification from fish viscera involved several steps, including ammonium sulfate precipitation, size-exclusion and ion-exchange chromatography, hydrophobic interaction chromatography and affinity chromatography.[Citation7,Citation8,Citation10,Citation11] In the view of their properties, these methods are time-consuming and high-cost purification process. Thus, ATPS could be an efficient method for the recovery of trypsin from albacore tuna spleen due to ease and lower cost.
Table 3. Purification of trypsin from albacore tuna spleen using ATPS.
Characterization of recovered trypsin
Protein pattern and activity staining for ATPS fractions
The SDS-PAGE analysis of protein samples from albacore tuna spleen extract and various extraction steps using the ATPS process is shown in . The crude splenic extract contained a variety of proteins with different molecular weights. However, a large number of contaminating proteins were removed after partitioning with ATPS, especially proteins with higher and lower MW. As a result, a higher purity of interested trypsin was obtained. Trypsin obtained by ATPS of 15% PEG4000 and 15% NaH2PO4 at 40°C was near homogenous with estimated molecular mass of 24 kDa. When the proteins or enzymes to be separated differ significantly in their structural properties from others, partitioning can be carried out successfully. The partitioning by ATPS becomes more complicated when those differences are minor.[Citation26]
Figure 3. SDS-PAGE of splenic extract and ATPS fractions from albacore tuna. M, molecular weight standard; lane 1, splenic extract; lane 2, 20% PEG1000–15% NaH2PO4 ATPS fraction; lane 3, 15% PEG4000–15% NaH2PO4 ATPS fraction; lane 4, 15% PEG4000–15% NaH2PO4 at 40°C ATPS fraction.
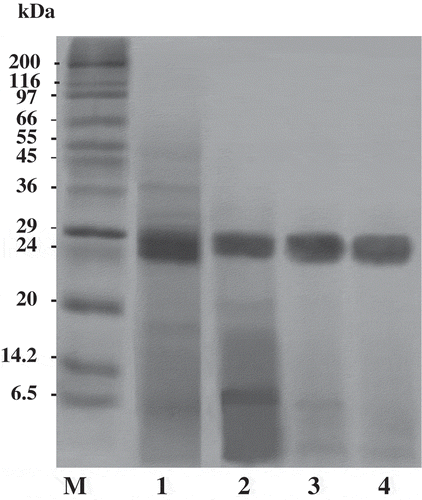
The trypsin in splenic extract from albacore tuna and fraction obtained from ATPS were identified by SDS-substrate polyacrylamide gels (). The apparent MWs of the major trypsin activity bands were estimated to be 22 and 24 kDa for all fractions. However, minor activity bands were found with apparent MW of 31 and 34 kDa. The band intensity slightly increased after the ATPS process. The biggest trypsin activity band was found in the 15% PEG4000–15% NaH2PO4 at 40°C. The results suggested the higher specific activity of trypsin loaded into the gel. Fuchise et al.[Citation11] reported that the molecular weight of trypsin from Pacific cod (Gadus macrocephalus) was 24 kDa. Bezerra et al.[Citation35] found that trypsin from pyloric caeca of tambaqui (Colossoma macropomum) was 38.5 kDa. Recently, Poonsin et al.[Citation5] reported that the major proteinases in albacore tuna spleen with MW of 22, 24, 31, and 34 kDa are trypsin or trypsin-like enzyme. From the results, ATPS was demonstrated to be efficient and inexpensive method of purification of albacore tuna spleen trypsin.
Hydrolysis of Pacific white shrimp shells using fractionated trypsin
Protein patterns of hydrolyzed Pacific white shrimp shells with and without fractionated trypsin from albacore tuna spleen are shown in . The apparent molecular weights (MW) of the major protein bands of protein recovered without fractionated trypsin were estimated to be 72, 38, and 21 kDa. The minor bands were observed with apparent MWs of 200, 43, 36, and 35 kDa (). MWs of proteins were similar to carotenoprotein in black tiger shrimp shells (211, 76, 43, and 31 kDa).[Citation9] Cheesman and Zagalsky[Citation36] reported that MW of crustacyanin from lobster (Homarus gammarus) is 36 kDa. Garate et al.[Citation37] also reported that the MWs of crustacyanin of the carapace crayfish Procambarusclarkii (Girard) are 19.2 and 21.4 kDa. After treatment of shrimp shells with fractionated trypsin, the protein band with high MW, especially 200 kDa, was totally disappeared. Furthermore, the band intensity of proteins MW of 72, 38 and 21 were decreased and the coincidental formation of bands with the lower MW was observed. The effect of fractionated trypsin at different levels was also studied. The protein bands with high MW were increasingly removed, when enzyme fraction concentration was increased. For protein patterns of hydrolyzed shrimp shells recovered by incubating the reaction mixture at 55°C for 15 min without the addition of enzyme, the proteins were also hydrolyzed by autolysis process as evidenced by the decrease in band intensity. Therefore, trypsin recovered from albacore tuna spleen using ATPS could be an alternative potential aid for extraction of carotenoprotein from shrimp shell, in which the cost of enzyme could be reduced.
Conclusion
An aqueous two-phase system was demonstrated to be an efficient primary purification step for albacore tuna spleen trypsin. The best condition of ATPS for trypsin partitioning from albacore tuna spleen was the 15% PEG4000–15% NaH2PO4 system at 40°C. Fractionated trypsins were able to hydrolyze Pacific white shrimp shell in a dose-dependent manner. Scaling up two-phase partition could form part of future industrial purification protocols for recovery of albacore tuna spleen trypsin.
Funding
The authors would like to express their appreciation to Thailand Graduate Institute of Science and Technology (SCA-CO-2559-2285-TH) for support. The TRF distinguished research professor grant and Thaksin University were also acknowledged.
Additional information
Funding
References
- Nalinanon, S.; Benjakul, S.; Visessanguan, W.; Kishimura, H. Partitioning of Protease from Stomach of Albacore Tuna (Thunnus alalunga) by Aqueous Two-Phase Systems. Process Biochemistry 2009, 44, 471–476.
- Gildberg, A. Recovery of Proteinases and Protein Hydrolysates from Fish Viscera. Bioresource Technology 1992, 39, 271–276.
- Haard, N.F. A Review of Proteotlytic Enzymes from Marine Organisms and their Application in the Food Industry. Journal of Aquatic Food Product Technology 1992, 1, 17–35.
- Castillo-Yanez, F.J.; Pacheco-Aguilar, R.; Garcia-Carreno, F.L.; Navarrete-Del Toro, M.A. Isolation and Characterization of Trypsin from Pyloric Caeca of Monterey Sardine Sardinops Sagax Caerulea. Comparative Biochemistry and Physiology-Part B: Biochemistry and Molecular Biology 2005, 140, 91–98.
- Poonsin, T.; Sripokar, P.; Benjakul, S.; Simpson, B.K.; Visessangunan, W.; Klomklao, S. Major Trypsin Like-Serine Proteinases from Albacore Tuna (Thunnus alalunga) Spleen: Biochemical Characterization and the Effect of Extraction Media. Journal of Food Biochemistry 2016. In Press.
- Klomklao, S.; Benjakul, S.; Kishimura, H.; Osako, K.; Tanaka, M. Effect of Salts and Polyethylene Glycols on the Partitioning and Recovery of Trypsin from Hybrid Catfish Viscera in Aqueous Two-Phase Systems. Journal of Food Biochemistry 2010, 34, 730–747.
- Klomklao, S.; Benjakul, S.; Visessanguan, W.; Kishimura, H.; Simpson, B.K.; Saeki, H. Trypsins from Yellowfin Tuna (Thunnus albacores) Spleen: Purification and Characterization. Comparative Biochemistry and Physiology-Part B: Biochemistry and Molecular Biology 2006, 144, 47–56.
- Villalba-Villalba, A.G.; Ramírez-Suárez, J.C.; Valenzuela-Soto, E.M.; Sánchez, G.G.; Ruiz, G.C.; Pacheco-Aguilar, R. Trypsin from Viscera of Vermiculated Sailfin Catfish, Pterygoplichthys disjunctivus, Weber, 1991: Its Purification and Characterization. Food Chemistry 2013, 141, 940–945.
- Klomklao, S.; Benjakul, S.; Visessanguan, W.; Kishimura, H.; Simpson, B.K. Extraction of Carotenoprotein from Black Tiger Shrimp with the Aid of Bluefish Trypsin. Journal of Food Biochemistry 2009, 33, 201–217.
- Hau, P.V.; Benjakul, S. Purification and Characterization of Trypsin from Pyloric Caeca of Bigeye Snapper (Pricanthus macracanthus). Journal of Food Biochemistry 2006, 30, 478–495.
- Fuchise, T.; Kishimura, H.; Sekizaki, H.; Nonami, Y.; Kanno, G.; Klomklao, S.; Benjakul, S.; Chun, B.S. Purification and Characteristics of Trypsins From Cold-Zone Fish, Pacific Cod (Gadus macrocephalus) and Saffron Cod (Eleginus gracilis). Food Chemistry 2009, 116, 611–616.
- Huddleston, J.; Veide, A.; Köhler, K.; Flanagan, J.; Enfors, S.O.; Lyddiatt, A. The Molecular Basis of Partitioning in Aqueous Two-Phase Systems. Trends in Biotechnology 1991, 9, 381–388.
- Yang, S.; Huang, Z.; Jiang, Z.; Li, L. Partition and Purification of a Thermostable Xylanase Produced by Paecilomyces thermophila in Solid-State Fermentation using Aqueous Two-Phase Systems. Process Biochemistry 2008, 43, 56–61.
- Babu, B.R.; Rastogi, N.K.; Raghavarao, K.S.M.S. Liquid-Liquid Extraction of Bromelain and Polyphenol Oxidase using Aqueous Two-Phase System. Chemical Engineering and Processing 2008, 47, 83–89.
- Oliveira, L.A.; Sarubbo, L.A.; Porto, A.L.F.; Campos-Takaki, G.M.; Tambourgi, E.B. Partition of Trypsin in Aqueous Two-Phase Systems of Poly(Ethylene Glycol) and Cashew-Nut Tree Gum. Process Biochemistry 2002, 38, 693–699.
- Chouyyok, W.; Wongmongkol, N.; Siwarungson, N.; Prichanont, S. Extraction of Alkaline Protease using an Aqueous Two-Phase System from Cell Free Bacillus subtilis TISTR 25 Fermentation Broth. Process Biochemistry 2005, 40, 3514–3518.
- Rnghavarno, K.S.M.S.; Guinn, M.R.; Todd, P. Recent Developments in Aqueous Two-Phase Extraction in Bioprocessing. Separation and Purification 1998, 27, 1–49.
- Klomklao, S.; Benjakul, S.; Visessanguan, W.; Simpson, B.K.; Kishimura, H. Partitioning and Recovery of Proteinase from Tuna Spleen by Aqueous Two-Phase Systems. Process Biochemistry 2005, 40, 3061–3067.
- Bradford, M.M. A Rapid and Sensitive Method for Quantitation of Microgram Quantities of Protein Utilizing the Principle of Protein Dye Binding. Analytical Biochemistry 1976, 72, 248–254.
- Laemmli, U.K. Cleavage of Structural Proteins during the Assembly of the Head of Bacteriophage T4. Nature 1970, 227, 680–685.
- Steel, R.G.D.; Torrie, J.H. Principles and Procedures of Statistics; McGraw-Hill: New York, 1980; 481 pp.
- Rawdkuen, S.; Pintathong, P.; Chaiwut, P.; Benjakul, S. The Partitioning of Protease From Calotropis Procera Latex by Aqueous Two-Phase Systems and its Hydrolytic Pattern on Muscle Proteins. Food and Bioproducts Processing 2011, 89, 73–80.
- Reh, G.; Nerli, B.; Pico ́, G. Isolation of Alpha-1-Antitrypsin from Human Plasma by Partitioning in Aqueous Biphasic Systems of Polyethyleneglycol-Phosphate. Journal of Chromatography B 2002, 780, 389–396.
- Farruggia, B.; Rigatuso, R.; Capezio, L.; Diez, V.; Pico ́, G. Influence of High Concentration Monovalent Cations on the Protein Partitioning in Polyethyleneglycol 1500-Phosphate Aqueous Two-Phase Systems. Journal of Chromatography B 2004, 809, 301–306.
- Johansson, G. Affinity Partitioning of Proteins using Aqueous Two-Phase Systems. In Protein Purification: Principles, High-Resolution Methods, and Applications; Janson, J.C., Ed.; Wiley-VCH: London, 1998; 443–460.
- Han, J.H.; Lee, C.H. Effects of Salts and Poly(ethylene glycol)-Palmitate on the Partitioning of Proteins and Bacillus subtilis Neutral Protease in Aqueous Two-Phase Systems. Colloids and Surfaces B: Biointerfaces 1997, 9, 109–116.
- Ketnawa, S.; Rawdkuen, S.; Chaiwut, P. Two Phase Partitioning and Collagen Hydrolysis of Bromelain from Pineapple Peel Nang Lae cultivar. Biochemical Engineering Journal 2010, 52, 205–211.
- Pan, I.H.; Yao, H.J.; Li, Y.K. Effective Extraction and Purification of β-xylosidase from Trichoderma koningii Fermentation Culture by Aqueous Two-Phase Partitioning. Enzyme and Microbial Technology 2001, 28, 196–201.
- Saravanan, S.; Rao, J.R.; Nair, B.U.; Ramasami, T. Aqueous Two-Phase Poly(ethylene glycol)-poly(acrylic acid) System for Protein Partitioning: Influence of Molecular Weight, pH and Temperature. Process Biochemistry 2008, 43, 905–911.
- Show, P.L.; Tan, C.P.; Anuar, M.S.; Ariff, A.; Yusof, Y.A.; Chen, S.K.; Ling, T.C. Extractive Fermentation for Improved Production and Recovery of Lipase Derived from Burkholderia cepacia using a thermoseparating Polymer in Aqueous Two-Phase Systems. Bioresource Technology 2012, 116, 226–233.
- Reh, G.; Splenzini, D.; Tubio, G.; Pico, G.; Farruggia, B. Partition Features and Renaturation Enhancement of Chymosin in Aqueous Two Phase Systems. Journal of Chromatography B 2007, 860, 98–105.
- Arafat, G.M.; Hong, Y.; Min, C.; Charles, L. Aqueous Two-Phase Extraction Advances for Bioseparation. Journal of Bioprocessing and Biotechiques 2013, 4, 1–8.
- Zaslavsky, B.Y. Aqueous Two-Phase Partitioning: Physical Chemistry and Bioanalytical Application; Marcel Dekker: New York, 1995; 656 pp.
- Ketnawa, S.; Benjakul, S.; Ling, T.C.; Martínez-Alvarezand, O.; Rawdkuen, S. Enhanced Recovery of Alkaline Protease from Fish Viscera by Phase Partitioning and its Application. Chemistry Central Journal 2013, 7, 79–87.
- Bezerra, R.S.; Santos, J.F.; Paiva, P.M.G.; Correia, M.T.S.; Coelho, L.C.B.B.; Vieira V.L.A.; JR, L.B.C. Partial Purification and Characterization of a Thermostable Trysin from Pyloric Caeca of Tambaqui (Colossoma macropomum). Journal of Food Biochemistry 2001, 25, 199–210.
- Cheesman, D.F.; Zagalsky, P.F. Purification and Properties of Crustacyanin. Proceedings of the Royal Society B Biological Sciences 1996, 164, 130–151.
- Garate, A.M.; Milicua, J.C.G.; Gomez, R.; Macarulla, J.M.; Britton, G. Purification and Characterization of the Blue Carotenoprotein from the Carapace of the Crayfish Procambarus Clarkii (Girard). Biochimica et Biophysica Acta (BBA) 1986, 881, 446–455.