ABSTRACT
In this study, the rheological and structural properties of extracted fish scales gelatin by conventional water bath (CWB) and ultrasound-assisted water bath (UWB) at different temperatures (60°C, 70°C and 80°C) were studied. Rheological behaviours showed that the extracted fish scales gelatin by UWB at 60°C possessed the highest storage modulus (5000 Pa), gelation point (22.94°C), melting point (29.54°C), and apparent viscosity than the others. X-ray showed that high temperature and ultrasound reduced the triple-helix content of fish scales gelatin along with decreasing gelling properties. Atomic force microscopy showed that the largest aggregates could be observed in the surface of UWB60. Therefore, UWB proved to be a promising technology in the extraction of high-quality fish gelatin.
Introduction
Gelatin, a proteinaceous material derived from collagen, is an important biological polymer that has been widely used in food and pharmaceutical industries.[Citation1–Citation4] However, with the emergence of bovine spongiform encephalopathy and foot-and-mouth disease affecting human health, the safe use of mammalian gelatin has been disputed for many years.[Citation5] Furthermore, pig gelatin is not acceptable in Judaism and Islam because of their religious beliefs.[Citation6] Therefore, fish gelatin is considered as a promising alternative to mammalian gelatin due to its similar properties with mammalian gelatin.[Citation1,Citation3,Citation7] However, the market share of fish gelatin is very low because of the insufficient availability of raw materials, inferior rheological properties, variation of gelatin quality, etc.[Citation1,Citation3,Citation8,Citation9] The yield and quality of fish gelatin are influenced not only by the species or tissues from which it is extracted, but also by process variables, such as pH, temperature, and time during pretreatment and extraction.[Citation1,Citation10]
Conventional water bath (CWB) is a conventional extraction method and frequently used to extract fish gelatin from other sources, such as splendid squid,[Citation11] shark skin,[Citation12] and giant grouper skin[Citation9] .However, this process usually requires long time and high temperature, which could significantly influence the quality of gelatin.[Citation10] Thus, exploring effective preparation technologies to improve yield and guarantee the quality of fish gelatin has attracted the interests of researchers.
Recently, ultrasound treatment has emerged as a potential approach to extract substances from raw materials for improving the extraction efficiency and shortening the extraction time.[Citation13] In addition, many studies have reported that ultrasound treatment could improve the functional properties (solubility, emulsifying, gel, and rheological properties) of protein by changing the protein conformation.[Citation13–Citation15] Fish scales are composed of calcium phosphate and calcium carbonate, and are rich in protein (41–84%), and can serve as raw materials for the production of gelatin.[Citation7] Our previous work has shown that ultrasound-assisted water bath (UWB) could improve the yield and gel strength of fish scales gelatin.[Citation16] However, the yield and functional properties of fish gelatin were influenced not only by time, but also by extraction temperature.[Citation5] Recent studies demonstrated that gelatin could be hydrolysed under high temperature, resulting in gelatin with low gel properties.[Citation1,Citation10] Thus, it is important to identify a suitable extraction temperature for the functional properties of gelatin.
Rheological properties not only have great influences on the manufacture, storage, as well as the texture of hydrogels composed of proteins and polysaccharides, but also inherently reflect the molecular structure and chain conformation of macromolecules.[Citation4,Citation17] The rheological behaviours of fish gelatin are mainly influenced by pH, temperature, light chemicals, electrical and magnetic field, etc.[Citation17] With respect to extracted fish gelatin, several previous studies have reported their rheological characteristics.[Citation4,Citation18–Citation22] However, little information on the rheological behaviours of UWB-extracted fish gelatin has been established. In this work, the rheological behaviours as well as the structural properties of fish scales gelatin under different extractions were determined, aiming at further understanding the UWB influence on fish gelatin and obtaining fish gelatin with better rheological properties.
Materials and methods
Materials
Fresh bighead carp (Hypophthalmichthys nobilis) scales were obtained from a local fresh fish market in Nanchang city (Jiang Xi, China). Hydrochloric acid, sodium hydroxide, sodium chloride, sodium dodecyl sulphate (SDS), and other reagents were all of analytical grade. Double-distilled water was used throughout all the experiments.
Extraction of gelatin from bighead carp scales
Fish scales were washed with running water and immersed in 10% (w/v) NaCl solutions to remove impurity and unnecessary proteins, respectively. The fresh fish scales were decalcified with the method reported by Tu et al.[Citation16] After decalcification, the wet fish scales (300 g) were divided into six batches, and mixed with distilled water at a ratio of 1:3 (w/v). CWB extraction was performed at 60°C, 70°C, and 80°C for 1 h, labelled as CWB60, CWB70, and CWB80, respectively. The gelatin was extracted using an ultrasound purifier instrument (KQ 5200DE, Kun Shan Ultrasound Instrument, China) upon 200 W (40 kHz) at 60°C, 70°C, and 80°C for 1 h, named as UWB60, UWB70, and UWB80, respectively. Then the mixture was filtered through two layers of filter papers under vacuum. The gelatin solution was lyophilized and the yield is listed in Table S1.
Preparation of fish gelatin solution
The moisture, crude protein (micro Kjedahl), and ash content of lyophilized gelatin were determined according to AOAC[Citation23] (Table S1). Lyophilized fish gelatin was dissolved with deionized water in a water bath at 40°C for preparing 6.67% (w/v) protein solution.
Rheological measurements
Rheological measurements were performed according to the method of Pang et al.[Citation24] using a Paar–Physica MCR 302 controlled stress rheometer (Anton Paar GmbH, Ostfildern, Germany) equipped with stainless steel cone and plate geometry (CP 50–1, 50 mm diameter), and the gap was set as 0.102 mm. Silicone oil was spread over the outer edge of the gelatin to prevent water evaporation during heating. Temperature sweep tests were run from 40°C to 10°C followed by 10°C to 40°C using cooling /heating rate of 1°C/min at 1 Hz with constant 0.5% strain.
To assess the cross-linking behaviour of gelatin, a dynamic frequency sweep was performed from 0.1 to 100 Hz at 10°C and 0.5% strain. Gelatin gels were matured at 10°C for 15 min before measurement. The storage compliance J’ is evaluated as follows:[Citation25]
The gel strength is related to the value of storage compliance
at a frequency where G” exhibits a shallow dip[Citation25] as follows:
All the tests were measured within the identified linear viscoelastic region.
The gelatin solution was held at 25°C for 3 min. The shear rate was ramped from 0.01 to 100.00/s.[Citation3] Finally, the apparent viscosity was plotted as a function of this parameter. All experiments were performed in triplicate, with a standard deviation typically below 20%. The results presented in this work are examples of typical data obtained, not averages.
Emulsifying activity and emulsion stability
Emulsifying activity index (EAI) and emulsifying stability index (ESI) of the gelatin solution were determined according to the method of Nagarajan et al.[Citation11] Fish gelatin solution (0.1%, w/v) was prepared by dissolving dry fish gelatin in phosphate buffer (pH 7.0). Soybean oil (3 ml) and gelatin solution (9 ml) were homogenized using an Ultra-Turrax T25 Disperser (IKA-Werke, Staufen, Germany) at a speed of 20,000 rpm for 1 min at room temperature. The emulsion was pipetted out at 0 and 10 min and 100-fold diluted with 0.1% SDS solution, respectively. The mixture was mixed thoroughly for 10 s using a vortex mixer (WH-866, Ji ke Shanghai, China), then the absorbance was measured at 500 nm using a spectrophotometer (Model UV-3200, Mapada, Shanghai, China). EAI and ESI were calculated by the following formula:
where DF = dilution factor (100), l = path length of cuvette (m), φ = oil volume fraction, and C = protein concentration in aqueous phase (g/m3).
where A0 = A500 at time of 0 min, A10 = A500 at time of 10 min, and Δt = 10 min.
X-ray diffraction analysis
X-ray diffraction of fish gelatin was determined according to the method of Huang et al.[Citation26] using A D8 X-ray diffractometer (Bruker AXS Co., German). Copper Ka was used at 40 kV and 35 mA. The 2Ɵ range was set from 5° to 40°.
Atomic force microscopy analysis
Characterization of the gelatin nanostructure was carried out by atomic force microscopy (AFM) with an Agilent atomic force microscope (Agilent 5500, Agilent technologies, Santa Clara, CA, USA) according to the method of Huang et al.[Citation3] with some modifications. About 20 µL of gelatin solution (0.667% w/v) was pipetted rapidly onto a piece of freshly cleaved mica sheet that stuck onto a 15-mm-diameter AFM specimen disc. The silicon surface was then air dried and kept in a desiccator for at least 24 h before imaging. Imaging was taken in the tapping mode with a resonance frequency around of 330 kHz, a force constant of 48 N/m, and a scan rate of 0.6 Hz. All samples were tested at ambient conditions (25 ± 0.5°C and 50% ± 5 humidity).
Statistical analysis
The data were subjected to analysis of variance (ANOVA); the probability value of p < 0.05 was considered significant. All analyses were performed using an SPSS package (SPSS 17.0 for window, SPSS Inc., Chicago, IL, USA).
Results and discussion
Analysis of rheological behaviour
Temperature sweep
The development of storage modulus (G’) during the gel formation of fish gelatin is shown in the heating and cooling curves ( and ). During the initial cooling stage, all the gelatin solutions exhibited fluidity with low G’ and a relatively high loss modulus (G”). The steep increases in G’ and G” were observed as the temperature was decreased to 10°C, before which they remained fairly constant at high temperatures. The crossover of the G’ and G’’ curves is defined as the gelation point at which the gelatin was transformed from liquid-dominated phase to solid-dominated phase, indicating the formation of a gel network.[Citation27] The gelatin gels are formed through the single-strand to triple-helix transition of gelatin chains via ionic interaction, hydrogen bonding, van der Waals forces, hydrophobic association, and self-assembly.[Citation3] As shown in , UWB60 exhibited gelling first, indicating that the protein strands of UWB60 started self-associating and forming triple helices quickly upon cooling. In addition, all G’ and G” showed a clear dependence on temperature as a result of the increasing ability of gelatin to form triple helices along with the reduction of gelation temperature.[Citation28]
Figure 1. Storage modulus and loss modulus of the extracted gelatin from bighead carp scales by conventional water bath (CWB) and ultrasound-assisted water bath (UWB) upon temperature sweep from 40°C to 10°C (A) and from 10°C to 40°C. CWB60, CWB70, CWB80, UWB60, UWB70, and UWB80 mean fish gelatin was extracted at temperatures of 60°C, 70°C, and 80°C by CWB and UWB for 1 h, respectively.
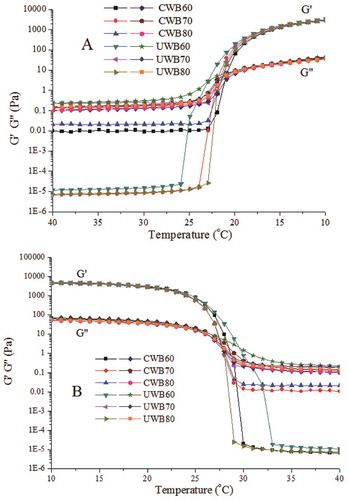
During the heating stage, both G’ and G” decreased as the temperature increased (), indicating the gelatin’s gel network was weakening. The melting point was obtained at the crossover point of G’ and G”.[Citation3] Above the melting point, the gels started to melt and the amount of triple helices decreased, and G” became higher than G’, indicating the transition of gel to liquid state. The reduction in G’ resulted in the loss of a network structure, and gelatin ultimately became a viscous liquid at higher temperatures because of the reduction of the triple helical structure.[Citation29] Compared with CWB, UWB provides more power to facilitate more high-molecular weight fragments of fish scales gelatin degrade during the extraction process as its mechanical and cavitation effects, resulting in weakening the gel network.[Citation16] Interestingly, in this study, gelatins prepared by UWB at 60, 70, and 80°C exhibited higher gelation and melting points than those prepared by CWB, and UWB60 had the highest gelation and melting points ( and Fig. S1). Furthermore, gelatin extracted by UWB had relatively higher max G’ at the same temperature than CWB. According to the viewpoints of Bode et al.[Citation28] and Huang et al.,[Citation3] gelatin extracted by UWB had higher contents of triple helices. These demonstrated that UWB could guarantee the gelatin structure, showing better rheological behaviours.
Table 1. Gel point, melting point, and max modulus of the extracted gelatin by CWB and UWB at a temperature of 60–80°C for 1 h.
Gel strength
The strength of the gel network can be explained by studying the frequency dependence of G’ and G”.[Citation25,Citation30] shows a typical frequency spectrum for a gel system, used to evaluate gel strength with Eqs. (1) and (2). Interestingly, the overall gel strength Gn was slightly enhanced as the oscillatory frequency increased, suggesting that the gel network was strengthened. In this study, UWB60 exhibited the highest gel strength Gn upon increasing frequency, which suggested that UWB60 possessed the strongest gel structure (Fig. S2). This observation is confirmed by evaluating the tan δ values (). When tan δ < 0.1, a confirmation of the solid state of gels is obtained.[Citation3] In this study, all the tan δ were lower than 0.1, indicating a good gel network or a more solid-like nature of the gels.[Citation30] However, all the tan δ of fish scales gelatin gels increased with increasing frequency, indicating a tendency towards weakening gel network behaviour at higher frequencies.[Citation3]
Figure 2. Gel strength (A) and tan δ (G”/G’) (B) of gelatin gels upon frequency sweep at 10°C; the viscosity of different gelatin solutions upon shear rate was set at 25°C (C). CWB60, CWB70, CWB80, UWB60, UWB70, and UWB80 mean fish gelatin was extracted at temperatures of 60°C, 70°C, and 80°C by CWB and UWB for 1 h, respectively.
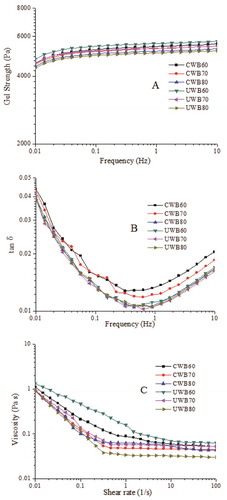
Apparent viscosity
Viscosity is partially controlled by molecular weight, molecular size distribution, and extraction conditions.[Citation31] Fig. 2C shows the apparent viscosity as a function of the shear rate for the samples. UWB60 exhibited the highest viscosity, and thus the widest applications. Jambrak et al.[Citation15] showed that ultrasound treatment could cause statistically significant changes in the flow behaviour indices and the consistency coefficients of soy protein isolate. Jambrak et al.[Citation32] also indicated that ultrasound had insignificant effect on the flow behaviour indices and consistency coefficients of α-lactalbumin, but all presented a time-independent non-Newtonian character. Viscosity was related to microstructure, and lower viscosity resulted in a weaker structure.[Citation22] In this study, UWB60 showed the highest apparent viscosity with the strongest structure, which might explain why UWB60 exhibited the best gel properties. Furthermore, as the shear rate increased, all the gelatin solutions exhibited shear thinning behaviour. This phenomenon might be caused by the changes in gels dispersity, molecular shape, and the strength of the intra-molecular chemical bonds upon various shear rates.[Citation3] These demonstrated the impairment of samples caused by shearing, and the structure was broken down.
Emulsifying properties
As shown in , an increased tendency of EAI could be observed at 70°C, and UWB70 showed the highest EAI among all the samples (p < 0.05). This might be due to partial gelatin hydrolysis at 70°C (as Fig. S3 illustrated), which in turn provided a better potentiality for the adsorption at the oil–water interface, resulting in an enhancement in EAI. This was similar to the results of Zhang et al.,[Citation13] who observed that ultrasonic treatment could induce peanut protein isolate unfolding, exposing the hydrophobic group of protein and improving its EAI. Interestingly, EAI decreased as the temperature reached 80°C; this might be due to the transformation of gelatin long chains into short chains induced by the higher temperature. Shorter chains possessing more hydrophilicity were preferably localized in the aqueous phase, leading to less-stabilized oil droplets by gelatin films and lower EAI.[Citation11] These results also highlighted that the ESI of gelatins obtained by CWB and UWB showed insignificant difference (p > 0.05), suggesting that ultrasound treatment for 1 h had no significant effect on the ESI of gelatin.
Table 2. Emulsifying and peak intensity at 7° of X-ray (counts) of gelatins extracted by CWB and UWB.
X-ray diffraction of fish gelatin
Fish gelatin usually shows two diffraction peaks at approximately 7–8° and 17–20°.[Citation33,Citation34] The peak at 2Ɵ around 7° is related to the diameter of the triple helix, whereas its intensity is associated with the triple helix content of the gelatin.[Citation6,Citation35] The diffractograms of dried gelatin are shown in . All the extracted gelatin showed two diffraction peaks at approximately 7° and 19–20°, which were similar to the diffraction peaks (at around 7° and 20°–21°) of bighead carp scales gelatin prepared using ammonium sulphate.[Citation6] This demonstrated that ammonium sulphate, high temperatures, and ultrasound had no significant effects on the diffraction peak values of fish gelatin.
Figure 3. X-ray spectra of gelatin extracted by CWB and UWB; CWB60, CWB70, CWB80, UWB60, UWB70, and UWB80 mean fish gelatin was extracted at temperatures of 60°C, 70°C, and 80°C by CWB and UWB for 1 h, respectively.
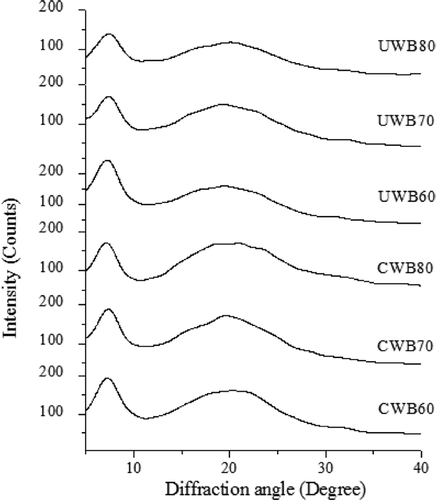
The intensity of diffraction peaks at around 7° is shown in . UWB60 possessed the highest peak intensity at 7°, indicating it had the highest content of triple helix. And this was also consistent with our rheology results. The peak intensity at 7° of gelatin decreased as the temperature increased, indicating that high temperatures could decrease the content of triple helix in fish gelatin and result in reduced gel properties (). Moreover, UWB at higher temperature (70–80°C) presented more damage to the triple helix content of gelatin than that of CWB. This might have resulted from the hydrolysis of fish gelatin facilitated by localized cavitation and heating effects, which then reduced the ability of gelatin to assemble into triple helices. This might explain why UWB80 exhibited weaker gel properties than CWB80.
Nanostructure of fish gelatin extracted by WB and UWB
Structural information can help in determining the properties of fish skin gelatin.[Citation4] Several studies have explored the nanostructure of fish gelatin.[Citation3,Citation9,Citation36] However, few studies have used AFM to observe the gelatin extracted by CWB and UWB at different temperatures. Therefore, the topographic images of gelatin extracted by CWB and UWB were studied and the structural changes are presented in . The extracted gelatin almost possessed a spherical structure and cluster on the surface, indicating the multimeric association process and homogeneity of fish gelatin. Similarly, Sow and Yang[Citation8] reported that large aggregates could be observed in tilapia fish gelatin. Shahiri et al.[Citation4] also showed acid white cheek shark gelatin with separated individual aggregates. The nanostructure of gelatin was largely dependent on concentration, because the way gelatin molecules fold together was impacted by protonation or deprotonation of the amino or carboxylic acid residues in the gelatin molecules.[Citation37] The aggregates were influenced by hydrogen bonds and hydrophobic interactions.[Citation8] Clearly, the largest irregular aggregates on the surface of UWB60 could be observed. The large irregular aggregates and heights decreased as the temperature increased from 60°C to 80°C. This phenomenon might be due to the crosslinking of gelatin under the effect of ultrasound and high temperatures.
Conclusion
Compared with CWB, UWB could be used to obtain fish gelatin with better rheological behaviours, higher gel strength, and emulsifying properties. The rheological results showed that UWB60 possessed the highest gelling and melting points, gel strength, and apparent viscosity. UWB could produce gelatin with a higher EAI, but had insignificant effect on ESI. High temperature and ultrasound reduced the intensity of diffraction peaks and triple helix contents, reducing the gel properties of the resulting gelatin. Moreover, AFM studies demonstrated that high temperature and ultrasound could decrease the aggregates in the fish gelatin surface. Thus, UWB conducted in a short time (1 h) could enhance the rheological behaviour of gelatin, and obtain high quality of fish scales gelatin. Moreover, the obtained fish gelatin should be studied further in modification, films, emulsion, emulsion gelation, and even release delivery systems.
LJFP_A_1295388_Supplemantal.doc
Download MS Word (1.8 MB)Funding
This work was funded by the Collaborative Innovation Center for Major Ecological Security Issue of Jiangxi Province and Monitoring Implementation (JXS-EW-00), Earmarked fund for Jiangxi Agriculture Research System (JXARS-04), Science and Technology of Jiangxi Province (No. 20142BBF60009), National Natural Science of Foundation of China (No. 31660487), and Jiangxi Province Science and Technology Support Program (No. 20143ACG70013).
Supplemental data
Supplemental data for this article can be accessed on the publisher’s website.
Additional information
Funding
References
- Karim, A.; Bhat, R. Fish Gelatin: Properties, Challenges, and Prospects as an Alternative to Mammalian Gelatins. Food Hydrocolloids 2009, 23, 563–576.
- Devi, A.F.; Liu, L.H.; Hemar, Y.; Buckow, R.; Kasapis, S. Effect of High Pressure Processing on Rheological and Structural Properties of Milk–Gelatin Mixtures. Food Chemistry 2013, 141, 1328–1334.
- Huang, T.; Tu, Z.-C.; Wang, H.; Shangguan, X. Pectin and Enzyme Complex Modified Fish Scales Gelatin: Rheological Behavior, Gel Properties and Nanostructure. Carbohydrate Polymers 2017, 156, 294–302.
- Shahiri Tabarestani, H.; Sedaghat, N.; Jahanshahi, M.; Motamedzadegan, A.; Mohebbi, M. Physicochemical and Rheological Properties of White-Cheek Shark (Carcharhinus dussumieri) Skin Gelatin. International Journal of Food Properties 2016, 19, 2788–2804.
- Sae‐Leaw, T.; Benjakul, S.; O’Brien, N.M.; Kishimura, H. Characteristics and Functional Properties of Gelatin from Seabass Skin as Influenced by Defatting. International Journal of Food Science & Technology 2016, 51, 1204–1211.
- Sha, X.-M.; Tu, Z.-C.; Liu, W.; Wang, H. Effect of Ammonium Sulfate Fractional Precipitation on Gel Strength and Characteristics of Gelatin from Bighead Carp (Hypophthalmichthys nobilis) Scale. Food Hydrocolloids 2014, 36, 173–180.
- Sankar, S.; Sekar, S.; Mohan, R.; Sunita, R.; Sundaraseelan, J.; Sastry, T.-P. Preparation and Partial Characterization of Collagen Sheet from Fish (Lates calcarifer) Scales. International Journal of Biological Macromolecules 2008, 42, 6–9.
- Sow, L.C.; Yang, H. Effects of Salt and Sugar Addition on the Physicochemical Properties and Nanostructure of Fish Gelatin. Food Hydrocolloids 2015, 45, 72–82.
- Lin, C.C.; Chiou, T.K.; Sung, W.C. Characteristics of Gelatin from Giant Grouper (Epinephelus lanceolatus) Skin. International. Journal of Food Properties 2015, 18, 2339–2348.
- Ahmad, T.; Ismail, A.; Ahmad, S.A.; Khalil, K.A. Recent Advances on the Role of Process Variables Affecting Gelatin Yield and Characteristics with Special Reference to Enzymatic Extraction: A Review. Food Hydrocolloids 2017, 63, 85–96.
- Nagarajan, M.; Benjakul, S.; Prodpran, T.; Songtipya, P.; Kishimura, H. Characteristics and Functional Properties of Gelatin from Splendid Squid (Loligo formosana) Skin as Affected by Extraction Temperatures. Food Hydrocolloids 2012, 29, 389–397.
- Kittiphattanabawon, P.; Benjakul, S.; Visessanguan, W.; Shahidi, F. Effect of Extraction Temperature on Functional Properties and Antioxidative Activities of Gelatin from Shark Skin. Food and Bioprocess Technology 2012, 5, 2646–2654.
- Zhang, Q.-T.; Tu, Z.-C.; Xiao, H.; Wang, H. Influence of Ultrasonic Treatment on the Structure and Emulsifying Properties of Peanut Protein Isolate. Food and Bioproducts Processing 2014, 92, 30–37.
- Rosell, C.M.; Marco, C.; García‐Alvárez, J.; Salazar, J. Rheological Properties of Rice–Soybean Protein Composite Flours Assessed by Mixolab and Ultrasound. Journal of Food Process Engineering 2011, 34, 1838–1859.
- Jambrak, A.R.; Lelas, V.; Mason, T.J.; Krešić, G.; Badanjak, M. Physical Properties of Ultrasound Treated Soy Proteins. Journal of Food Engineering 2009, 93, 386–393.
- Tu, Z.-C.; Huang, T.; Wang, H.; Sha, X.-M. Physico-Chemical Properties of Gelatin from Bighead Carp (Hypophthalmichthys nobilis) Scales by Ultrasound-Assisted Extraction. Journal of Food Science and Technology 2015, 52, 2166–2174.
- Huang, J.; Zeng, S.; Xiong, S.; Huang, Q. Steady, Dynamic, and Creep-Recovery Rheological Properties of Myofibrillar Protein from Grass Carp Muscle. Food Hydrocolloids 2016, 61, 48–56.
- Madhamuthanalli, C.V.; Bangalore, S.A. Rheological and Physico‐Chemical Properties of Gelatin Extracted from the Skin of a Few Species of Freshwater Carp. International Journal of Food Science & Technology 2014, 49, 1758–1764.
- Fernández-Dı́, M.; Montero, P.; Gómez-Guillén, M. Effect of Freezing Fish Skins on Molecular and Rheological Properties of Extracted Gelatin. Food Hydrocolloids 2003, 17, 281–286.
- Giménez, B.; Gómez-Guillén, M.; Montero, P. The Role of Salt Washing of Fish Skins in Chemical and Rheological Properties of Gelatin Extracted. Food Hydrocolloids 2005, 19, 951–957.
- Chandra, M.; Shamasundar, B. Rheological Properties of Gelatin Prepared from the Swim Bladders of Freshwater Fish Catla Catla. Food Hydrocolloids 2015, 48, 47–54.
- García, M.C.; Alfaro, M.C.; Calero, N.; Muñoz, J. Influence of Polysaccharides on the Rheology and Stabilization of Α-Pinene Emulsions. Carbohydrate Polymers 2014, 105, 177–183.
- AOAC, W.H. Official Methods of Analysis. The United States Pharmacopeia. Arlington, VA, 1990.
- Pang, Z.; Deeth, H.; Sopade, P.; Sharma, R.; Bansal, N. Rheology, Texture and Microstructure of Gelatin Gels with and without Milk Proteins. Food Hydrocolloids 2014, 35, 484–493.
- Fonkwe, L.G.; Narsimhan, G.; Cha, A.S. Characterization of Gelation Time and Texture of Gelatin and Gelatin–Polysaccharide Mixed Gels. Food Hydrocolloids 2003, 17, 871–883.
- Huang, T.; Tu, Z.-C.; Wang, H.; Shangguan, X. Comparison of Rheological Behaviors and Nanostructure of Bighead Carp Scales Gelatin Modified by Different Modification Methods. Journal of Food Science and Technology 2017. (In press). doi:10.1007/s13197-017-2511-1.
- Biscarat, J.; Galea, B.; Sanchez, J.; Pochat-Bohatier, C. Effect of Chemical Cross-Linking on Gelatin Membrane Solubility with a Non-Toxic and Non-Volatile Agent: Terephthalaldehyde. International Journal of Biological Macromolecules 2015, 74, 5–11.
- Bode, F.; Da Silva, M.A.; Drake, A.F.; Ross-Murphy, S.B.; Dreiss, C.A. Enzymatically Cross-Linked Tilapia Gelatin Hydrogels: Physical, Chemical, and Hybrid Networks. Biomacromolecules 2011, 12, 3741–3752.
- Mohtar, N.F.; Perera, C.O.; Quek, S.-Y.; Hemar, Y. Optimization of Gelatine Gel Preparation from New Zealand Hoki (Macruronus novaezelandiae) Skins and the Effect of Transglutaminase Enzyme on the Gel Properties. Food Hydrocolloids 2013, 31, 204–209.
- Sarbon, N.M.; Badii, F.; Howell, N.K. The Effect of Chicken Skin Gelatin and Whey Protein Interactions on Rheological and Thermal Properties. Food Hydrocolloids 2015, 45, 83–92.
- Koli, J.M.; Basu, S.; Nayak, B.B.; Patange, S.B. Functional Characteristics of Gelatin Extracted from Skin and Bone of Tiger-Toothed Croaker (Otolithes ruber) and Pink Perch (Nemipterus japonicus). Food and Bioproducts Processing 2012, 90, 555–562.
- Jambrak, A.R.; Mason, T.J.; Lelas, V.; Krešić, G. Ultrasonic Effect on Physicochemical and Functional Properties of Α-Lactalbumin. LWT-Food Science and Technology 2010, 43, 254–262.
- Zhang, F.; Xu, S.; Wang, Z. Pre-Treatment Optimization and Properties of Gelatin from Freshwater Fish Scales. Food and Bioproducts Processing 2011, 89, 185–193.
- Liu, Z.; Ge, X.; Lu, Y.; Dong, S. Effects of Chitosan Molecular Weight and Degree of Deacetylation on the Properties of Gelatine-Based Films. Food Hydrocolloids 2012, 26, 311–317.
- Bigi, A.; Panzavolta, S.; Rubini, K. Relationship between Triple-Helix Content and Mechanical Properties of Gelatin Films. Biomaterials 2004, 25, 5675–5680.
- Nazeer, R.; Deepthi, M.K. Physicochemical and Nanostructural Properties of Gelatin from Uneconomical Marine Cornet Fish (Fistularia petimba). Food Science and Biotechnology 2013, 22, 9–14.
- Saxena, A.; Sachin, K.; Bohidar, H.; Verma, A.K. Effect of Molecular Weight Heterogeneity on Drug Encapsulation Efficiency of Gelatin Nano-Particles. Colloids and Surfaces B: Biointerfaces 2005, 45, 42–48.