ABSTRACT
A novel heteropolysaccharide named SHDP was isolated from spent Hovenia dulcis peduncles (SHD) using alkali extraction. The preliminary characterization of the polysaccharide was performed by physicochemical and spectral methods. The bioactivities in vitro were also investigated. The results indicated that SHDPs are homogeneous with an average molecular weight of approximately 4570 kDa, and contain uronic acid (2.3%) and protein (3.6%). It is mainly composed of rhamnose, fucose, arabinose, xylose, mannose, glucose, and galactose in a molar ratio of 3.04:1:8.55:8.51:3.80:19.85:9.59. The structure perhaps contains 6)-Glcp-(1→, 3)-Rhap-(1→, 4)-Xylp-(1→, 3)-Fucp-(1→. Meanwhile, xylose and arabinose were attached to (1→3, 6)-Manp and (1→3, 6)-Galp. Fourier-transform infrared (FTIR) analysis showed the typical polysaccharide absorption of the polysaccharide. Antioxidant activities assays in vitro indicated excellent 1,1-diphenyl-2-picrylhydrazyl (DPPH) and 2,2ʹ-azino-bis (3-ethylbenzthiazoline-6-suphonic acid) diammonium (ABTS) radical scavenging activities, high superoxide radical scavenging activity, and medium chelating ferrous ions activity in a dose-dependent manner. In addition, SHDPs have the ability to enhance the activity of alcohol dehydrogenase (ADH), which plays an important role in the process of preventing alcoholism.
Introduction
H. dulcis Thunb. (Rhamnaceae), widely known as Guai Zao or Zhi Ju, is mainly distributed in China and Korea. H. dulcis tastes like a mixture of sugar, raisin, cinnamon, and clove[Citation1] and has been consumed as a medicinal and deciduous fruit in many countries. What is noteworthy is that the fleshy H. dulcis peduncles are full of nutrients[Citation2] that may protect our body from damage. Based on medicine literature, most parts of H. dulcis, including peduncles, barks, seeds, and roots, are capable of slaking thirst, quenching acute alcohol toxicity, and anti-lipid peroxidation, among others.[Citation3] Especially, polysaccharides are one of the main constituents of the H. dulcis peduncles, and play an important role in pharmacologic functions. Recently, some researchers studied the strong antioxidant activities,[Citation3] immunostimulatory activity in vitro,[Citation1] and hepatoprotective effect in vivo[Citation4] of the polysaccharides from H. dulcis peduncles.
Compared with previous conventional extract-polysaccharide methods, such as boiling, heating, and ultrasonic extraction, alkali extraction technology is more simple and efficient, which has been used to obtain products from diverse natural sources. Spent H. dulcis peduncles (SHDs), as the residues of water extraction, still retain some cell wall polysaccharides. All these biological and physicochemical properties offered by water-soluble polysaccharides may be found in alkali polysaccharides from SHDs. However, only few articles and researches claimed that the characterization and bioactivities of polysaccharides extracted from SHDs by alkali extraction as exposed here. This in turn prevented it from being used in the exploitation, development, and utilization of H. dulcis. Therefore, to utilize fully and exploit effectively H. dulcis peduncles resource, the purpose of this study is to extract polysaccharides from SHD using an alkali treatment with the mixed liquor of sodium hydroxide and sodium borohydride. A homogeneous acidic polysaccharide named SHDPs was obtained. The preliminary structure was characterized by physicochemical and spectral methods. Moreover, the bioactivities in vitro including antioxidant activity and alcohol dehydrogenase (ADH) activity were evaluated.
Materials and methods
Raw materials, chemicals, and alkali extraction of SHDPs
Peduncles of H. dulcis were purchased from Ankang city, Shanxi province, China. The specimen was identified by Professor Junhui Wang. Voucher specimens were deposited in the herbarium of the School of Food Science and Engineering, Hefei University of Technology (No. HD001). The peduncles of H. dulcis were dried and ground into powder, which went through a 60-mesh sieve. The water-soluble polysaccharides were first extracted from the powder by hot water. After filtration, the residues were collected, dried, and kept at −20°C, which were the SHDs for alkali extraction.
The monosaccharide standards L-rhamnose, D-fucose, L-arabinose, D-xylose, D-mannose, D-glucose, and D-galactose (L-Rha, D-Fuc, L-Ara, D-Xyl, D-Man, D-Glc, and D-Gal), 2,2ʹ-azino-bis (3-ethylbenzthiazoline-6-suphonic acid) diammonium (ABTS), 1,1-diphenyl-2-picrylhydrazyl (DPPH), dimethyl sulfoxide (DMSO), and diethylaminoethyl (DEAE) cellulose were purchased from Sigma-Aldrich Co., Ltd. (St. Louis, MO, USA). Trifluoroacetic (TFA) and methyl iodide (CH3I) were purchased from Sinopharm Chemical Reagent Co., Ltd. Ultra-pure water was from a milli-Q water purification system. All other reagents were obtained from Sinopharm Chemical Reagent Co. Ltd (Shanghai, China) and are of analytical or chromatographic grade unless otherwise stated.
Polysaccharides from SHD were extracted according to the method described by Wang et al.[Citation5] with slight modification. Briefly, the powder of dried H. dulcis peduncles was graded through a 60-mesh screen, decoloured, defatted, and water-soluble polysaccharides were extracted with hot water at 100°C for 2 h twice. After filtration, the residues of SHDs were obtained. The fat-free SHD was dried to a constant weight and stored for further use. The alkali treatment was performed for polysaccharides extraction using SHD (170 g) added to 2 L of 5% NaOH/0.05% NaBH4 solution (100 g NaOH and 1 g NaBH4 dissolved in 2 L water), stirring constantly for 2 h at room temperature. The residues were discarded by centrifugation at 10000 × g for 10 min. The supernatant was collected and neutralized to pH 7 with glacial acetic acid immediately. Then the solution was concentrated and precipitated with a fourfold volume of 95% ethanol at 4°C for 24 h. The precipitation was gained, and dissolved in deionized water. Proteins in the solution were removed by the Sevage method[Citation6] and the process was repeated at least eight times.[Citation7,Citation8] The solution was decoloured, dialysed (Mw cut-off 3500), and lyophilized to obtain crude polysaccharides. Then, crude polysaccharides solution was purified by a DEAE cellulose chromatography column (3.0 × 40 cm), and eluted with distilled water at 0.25 mL/min. The elution curve was monitored by the phenol-sulphuric acid method[Citation9] and UV scanning at 260 nm and 280 nm.[Citation10] The obtained polysaccharide was named SHDPs.
Determination of homogeneity and average molecular weight
The homogeneity and average molecular weight of SHDPs were determined by high-performance liquid chromatography (HPLC) by Li’s method.[Citation11] The polysaccharide was dissolved in ultra-pure water at a concentration of 1.0 mg/mL and filtered through a 0.22 μm membrane. The proteins and nucleic acids were detected on a TU-1901 ultraviolet spectrophotometer at the wave-number range of 200–400 nm.
Analysis of chemical composition
The total sugar content of SHDPs was determined according to the phenol-sulphuric acid method in a 752-ultraviolet and visible spectrophotometer. Protein content was measured by the Bradford method at 595 nm.[Citation12] Uronic acid content was detected by the m-hydroxydiphenyl colorimetric method at 520 nm.[Citation13]
Analysis of monosaccharide composition
Five mg SHDPs were hydrolysed with TFA by Wang’s method.[Citation14] The hydrolysate was treated successively with 30 mg NaBH4 for 3 h. After neutralization with acetic acid, 3 mL acetic anhydride and 3 mL pyridine were added into the residue at 100°C and reacted for 1 h. The alditol acetate derivatives were analysed by gas chromatography-mass spectrometry (GC-MS; Shimadzu Co. Japan) according to the method of Chen et al.[Citation15] The monosaccharide standards were detected by the same methods. The monosaccharides were identified by their retention times (Rt) and quantitatively determined by their peak areas.
Analysis of partial acid hydrolysis
For the sake of simplicity and accuracy of structure, experiments of partial acid hydrolysis were performed. A total of 15 mg of sample was hydrolysed using 0.05 M TFA (8 mL) at 100°C for 3 h in a sealed test tube. The hydrolyte was transferred to dialysis bags and dialysed against distilled water for 48 h. Excess acid in the bag was removed by co-distillation with MeOH repeatedly. The hydrolyte inside the dialysis bag was dried by evaporation on a water bath (50°C), which was completely hydrolysed and converted into the corresponding alditol acetate derivatives. The monosaccharide composition was analysed as described in section of “analysis of monosaccharide composition”
Analysis of Fourier-transform infrared
The Fourier-transform infrared (FTIR) spectra were recorded on a Nicolet 5700 FTIR spectrometer in the wave number range of 4000–500 cm−Citation1 according to the method of potassium bromide (KBr) disc.[Citation16,Citation17] A total of 3 mg of sample was dried and ground with spectroscopic-grade KBr powder and pressed into 1 mm pellet for FTIR measurement.
Analysis of methylation and NMR spectroscopy
Before methylation, the sodium methyl sulfoxide (SMSM) reagent was prepared with free oil NaH and DMSO, using the method of Hakomori.[Citation18] Freeze-dried SHDPs (15 mg) were dissolved in dried DMSO (5 mL) and then methylated with the SMSM reagent (2 mL) and CH3I (2 mL). The reaction mixture was dialysed with tap water for 12 h. The insoluble substance was lyophilized to obtain methylated polysaccharide. Complete methylation was confirmed by the disappearance of the -OH band (3200–3700 cm−Citation1) in the FTIR spectrum by a 5700 FTIR spectrometer. The permethylated residue was depolymerized with 88% formic acid (3 mL) at 100°C for 6 h and evaporated to dry. After these procedures, the monosaccharide composition was analysed by GC. The methylated alditol acetate was analysed on a GC-MS instrument.
For Nuclear magnetic resonance (NMR) spectroscopy, 50 mg SHDPs was dried and dissolved completely in deuterium oxide (D2O) and the following treatments were performed by the method of Liu et al.[Citation19] and the chemical shifts were expressed in ppm.
Evaluation of antioxidant activity in vitro SHDPs
Assay of DPPH scavenging radical activity
The assay of DPPH scavenging radical activity was carried out according to the method of Xu et al.[Citation20] with some modifications. In total, 2 mL of SHDPs solution at different concentrations (0.02, 0.04, 0.06, 0.08, and 0.1 mg/mL) was mixed with freshly prepared DPPH (100 μmol/L in methanol), respectively. The mixtures were shaken for 2 min and incubated in the dark for another 30 min. The absorbance was measured at 517 nm against a blank (distilled water) and ascorbic acid (VC) was used as a positive control. The DPPH scavenging percentage was calculated by the following equation:
where A0 is the absorbance of the control (water instead of sample solution), A1 is the absorbance of the sample, and A2 is the absorbance of the sample under identical conditions as A1 with water instead of DPPH solution.
ABTS radical scavenging activity of SHDPs
The ABTS radical scavenging activity of SHDPs was determined as described in the reported method.[Citation21] The reaction mixture contained 0.2 mL of SHDPs solution with different concentrations (0.2, 0.4, 0.6, 0.8, and 1 mg/mL) and 4 mL of ABTS (7 mmol/L), respectively. After being incubated at room temperature for 6 min, the absorbance of the mixture was recorded at 734 nm. The distilled water was chosen as a blank and VC was used as a positive control. The ABTS radical scavenging activity of SHDPs was calculated by the following equation:
where A0 is the absorbance of the control (water instead of sample solution), A1 is the absorbance of the sample, and A2 is the absorbance of the sample under identical conditions as A1 with water instead of ABTS solution.
FeCitation2+ chelating activity of SHDPs
The FeCitation2+ chelating activity of SHDPs was evaluated as described previously[Citation22] with minor modifications. A total of 1 mL of polysaccharides sample at different concentrations (1, 2, 3, 4, and 5 mg/mL) was added to 0.1 mL FeCl2, 0.2 mL ferrozine, and 2.7 mL deionized water, respectively. The mixture was shaken well and incubated at room temperature for 10 min; the absorbance of the mixture was measured at 562 nm. The distilled water was chosen as a blank and ethylene diamine tetraacetic acid disodium salt (EDTA-2Na) was used as a positive control. The FeCitation2+ chelating activity of SHDPs was calculated by the following equation:
where A0 is the absorbance of the control (water instead of sample solution), A1 is the absorbance of the sample, and A2 is the absorbance of the sample under identical conditions as A1 with water instead of FeCl2 solution.
Superoxide anion radical scavenging activity of SHDPs
Superoxide anion radicals were generated by the pyrogallic acid method[Citation23] and the scavenging activity was measured as reported by Liu et al.[Citation19] The sample solution at different concentrations was reacted with Tris-HCl (pH 8.2), distilled water, and pyrogallic acid solution in sequence. After incubating at room temperature for 5 min, the reaction was terminated with HCl (8 mmol/L). Then, the absorbance at 560 nm was measured against a blank (distilled water), and VC was used as a positive control in the assay. The scavenging activity on the superoxide anion radical was calculated by the following equation:
where A0 is the absorbance of the control (water instead of the sample solution), A1 is the absorbance of the sample, and A2 is the absorbance of the sample under identical conditions as A1 with water instead of another reaction liquid.
Effect of SHDPs on ADH activity
The effects of SHDPs on the alcoholic dehydrogenase activity were tested by the Valle & Hoch method. The assay mixture consisted of 1.5 mL sodium pyrophosphate buffer of pH 8.8, 0.5 mL of oxidized coenzyme Ι (NAD+, 0.027 mol/L), 0.5 mL ethanol of 11.5%v/v(8.85 mL of distilled water mixed with 1.15 mL anhydrous ethanol), and the reaction was started immediately by adding 0.1 mL of different concentrations of SHDPs (5, 10, 15, 20, and 25 mg/mL) in tubes and sealed. After shaking vigorously, the mixture was incubated at 25°C in a water bath for 5 min to achieve temperature equilibrium. Then, 0.1 mL ADH (0.25 U/mL) was added to each mixture immediately, and shaken well. Reduction of nicotinamide adenine dinucleotide (NAD), reduced form of nicotinamide-adenine dinucleotid (NADH) was followed for 5 min by recording the absorbance at 340 nm resulting per 10 s. The preparation of sodium pyrophosphate buffer of pH 8.8 was 1.427 g of Na2P2O7 · 10H2O dissolved in distilled water, adjusted to pH 8.8 with 1 mol/L NaOH in a final volume of 100 mL. The increase of NADH absorption was measured as a function of time by a 752-ultraviolet/visible spectrophotometer. Around 0.1 mL distilled water instead of polysaccharide was used as a blank control.
The increase in absorbance (ΔA340/min) per 10 s reaction time was calculated from the initial linear portion of the standard curve. Extinction coefficient (ε) value of 6.22 mM−Citation1 cm−Citation1 for NADH at 340 nm was used to calculate activity. One unit of enzyme activity was defined as millimoles of NAD reduced per min at room temperature and pH 8.8. The enzymatic units and activation rate of ADH (U/mL) were calculated as follows:
where Ucontrol is the ADH activity of the negative control and Usample represents the ADH activity of the positive control.
Statistical analysis
Results were expressed as the means ± standard deviation (SD) of triplicate experiments.
Results and discussions
Chemical analysis of SHDPs
The SHDPs were first prepared from SHD by alkali treatment, followed by ethanol precipitation and vacuum freeze-drying. The overall yield of SHDPs was 1.5% based on the dried residues used. The results of chemical analysis showed that the contents of total sugar and uronic acid were determined to be (80 ± 2.8)% and (2.3 ± 0.1)%, respectively. Notably, SHDPs contains protein (3.6 ± 0.3)%. The analysis of HPLC revealed only one single elution peak as shown in , indicating that SHDP is a homogeneous polysaccharide, corresponding to an average molecular weight of 4570 kDa from the equation y = −0.133x + 9.369 (the log[Mw](x) was regressed on the Rt (y), RCitation2 = 0.963) about the dextran calibration curve. The UV spectrum of SHDPs is shown in ; it confirmed that SHDPs contained a little bit of protein because of the weak absorption at 260 and 280 nm. After purification by DEAE-cellulose, two absorption curves (shown in ) were obtained at 280 and 490 nm, respectively. It indicated that SHDP is a protein-bound polysaccharide because of the simultaneous maximum absorbance values at 490 and 280 nm.[Citation24] The polysaccharides from various materials may contain neutral sugar, protein, and uronic acid, and they were conjugated with each other to possess various biological activities. Moreover, it was found that higher contents of protein and uronic acid imply higher biological activities and diverse antioxidant activities.[Citation22] Meanwhile, because of carrying out the deproteinization process several times, it was supposed that the protein remained on the polysaccharide by covalent bonds.[Citation7] Moreover, SHDP is a non-starch polysaccharide owing to the negative responses to iodide-potassium iodide test.[Citation4]
Figure 1. Extraction and characterizations of SHDPs produced by H. dulcis. (A) The HPLC spectrum of SHDPs; (B) UV spectra of SHDPs; (C) DEAE-cellulose chromatography of polysaccharide from spent H. dulcis.
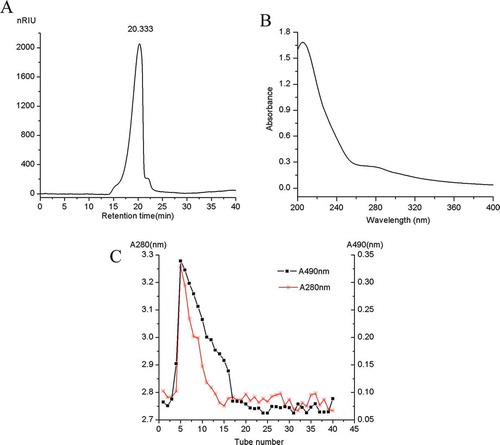
The monosaccharide compositions of SHDPs, fraction of partial acid hydrolysis, and monosaccharide standards are shown in –. The tested seven standard monosaccharides were completely separated from the baseline within 17 min, and the peaks in the chromatogram were identified in the order of L-Rha, D-Fuc, L-Ara, D-Xyl, D-Man, D-Glc, and D-Gal with the Rt of 11.813, 11.973, 12.028, 12.220, 15.398, 15.480, and 15.697 min, respectively. By comparing the Rt of SHDP with that of the commercial standards under the same conditions, SHDP contains Rha, Fuc, Ara, Xyl, Man, Glc, and Gal in a molar ratio of 3.04:1:8.55:8.51:3.80:19.85:9.59. The seven monosaccharides still existed in the fraction, combined with ; however, the relative contents of Ara and Xyl were decreased. This revealed that Ara and Xyl were located on the branch of SHDPs.
Analysis of FTIR spectroscopy, methylation, and NMR spectroscopy
FTIR spectra of SHDPs exhibited various typical absorption peaks of polysaccharides, as shown in . The intense stretching characteristic peak at 3391 cm−Citation1 represents hydroxyl group stretching vibration,[Citation24,Citation25] which is common to all polysaccharides. A weak absorption peak at 2926 cm−Citation1 suggests the stretching vibration of C-H in the sugar ring.[Citation26] The strong absorption band at 1040 cm−Citation1 corresponds to the stretching vibrations of C-O-C bonds, which confirm that SHDPs contain pyranose rings.[Citation27] A strong peak about 1630 cm−Citation1 and a weak peak about 1410 cm−Citation1 were the characteristic absorptions of the carboxylic group, which might be due to the appearance of a small amount of uronic acid and protein.[Citation3] The small peaks at 899, 862, and 754 cm−Citation1 suggested that β-linkage and α-linkage do exist in the SHDPs.[Citation24,Citation28,Citation29]
FTIR spectra of the methylated product are shown in . The absence of the hydroxyl peak at 3391cm−Citation1 and the obvious enhancement of C-H stretching vibration at 2926 cm−Citation1 indicated that the procedure of methylation was successful. The completely methylated SHDPs hydrolysed with TFA; the methylated derivatives were determined by GC-MS analysis. The identification and proportions are listed in . It revealed small amounts of 2,4-Me2-Rhap, 2,4-Me2-Fucp, 2,3-Me2-Xylp, 2,3,4-Me3-Xylp, 2,4-Me2-Manp and a large amount of 2,3,4-Me3-Glcp, 2,4-Me2-Galp, 2,3,5-Me3-Araf with a molar ratio of 13.569: 5.957: 6.084. This analysis shows that SHDPs contain these linkage forms: (1→3)-Rhap, (1→3)-Fucp, (1→4)-Xylp, (1→)-Xylp, (1→3,6)-Manp, (1→6)-Glcp, (1→3,6)-Galp, and (1→)-Araf. Based on the results of partial acid hydrolysis and GC-MS, we speculated that the terminal xylose and arabinose were attached to the (1→3,6)-Manp and (1→3,6)-Galp, respectively.
Table 1. Methylation analysis of SHDPs.
The structural properties of SHDPs were confirmed from NMR spectroscopy including Citation1H NMR and Citation13C NMR analyses. NMR spectroscopy is one of the most useful and important tools for the analysis of structural information such as monosaccharide, α-type or β-type anomeric configuration, and sequences of the sugar units.[Citation30] Most of the α-anomeric protons usually appear in the region that had chemical shifts larger than δ 4.8 ppm, while β-anomeric protons appear in the region of δ 4–4.8 ppm.[Citation31] the In Citation1H NMR spectrum of SHDPs (), all chemical shifts of anomeric proton at δ 4.9, 4.46, 5.18, 4.73, 4.40, 5.02, 4.93, and 5.06 ppm were lower or higher than 4.8 ppm, which suggested the presence of β-linkages and α-linkages in SHDPs. This was in agreement with the analysis results of FTIR. The weak resonance appearing around δ 1.96 ppm was attributed to the proton of acetyl group .[Citation24] The structure of SHDPs contains pyranose because of the absence of the anomeric proton at δ 5.4 ppm.[Citation32] The signal at δ 1.09 ppm was assigned to H-6 of α-L-Rhap and linked only at O-2.[Citation30] In the Citation13C spectrum (), the strong presence of carbon chemical shifts at δ 103.9 ppm was assigned to the anomeric carbon of 6)-β-D-Glcp-(1→ residue with a high proportion.[Citation11,Citation33] According to reference data set,[Citation24,Citation34–Citation39] the resonances at δ 104.9, 109.8, 105.7, 106.2, 104.3, 101.8, and 101.4 ppm were ascribed to the anomeric carbon of residues of 3,6)-α-D-Galp-(1→, α-L-Araf-(1→, 3,6)-β-D-Manp-(1→, 4)-β-D-Xylp-(1→, α-D-Xylp-(1→, 3)-α-D-Fucp-(1→, and 3)-α-L-Rhap-(1→, respectively. The signal identified at around δ 18.4 ppm was attributed to the methyl carbon of rhamnose residues.[Citation40] The weak signal in the region at δ 179.39 ppm originated from C-6 of the unesterified galacturonic acid unit.[Citation26]
Figure 4. NMR spectra of SHDPs. (A) Citation1H NMR spectrum of SHDPs; (B) Citation13C NMR spectrum of SHDPs.
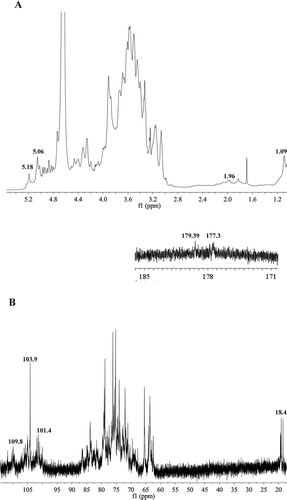
Antioxidant activities of SHDPs
The antioxidant activities of polysaccharides are due to their free radical scavenging and metal ion chelating activities. Among the free radical species, DPPH, ABTS radical, and superoxide radical are stable radicals, whose scavenging model has been widely used to evaluate the antioxidant activities of natural materials.[Citation41] Besides, as the most powerful oxidant with high reactivity, FeCitation2+ can stimulate lipid peroxidation by generating hydroxyl radicals through Fenton reaction.[Citation42,Citation43] Therefore, in this study, the scavenging activity against DPPH radical, ABTS radical, and superoxide radical, and ferrous ions chelating activity were selected to evaluate the antioxidant activity in vitro SHDPs.
shows the scavenging activity of SHDPs on DPPH radical. Noticeably, the scavenging activity of SHDPs increased with concentrations in a dose-dependent manner. At the concentration of 0.1 mg/mL of SHDPs and VC, the scavenging rates of DPPH free radical were 91.2% and 94.7%, respectively. Compared to water-extracted polysaccharide from H. dulcis,[Citation3] which displayed weak DPPH scavenging rate at IC50, SHDPs exerted stronger scavenging effect against DPPH radical with IC50. Many researches showed that the lower absorbance value of the solution represented a stronger free radical scavenging effect. DPPH radical was scavenged by a proton-donating substance on the base of reduced absorbance.[Citation20] It was evident that SHDP has a strong proton-donating ability and could be explored as a good antioxidant.
Figure 5. Antioxidant activities of SHDPs in vitro. (A) DPPH radical scavenging activity; (B) ABTS radical scavenging activity; (C) FeCitation2+ chelating ability; (D) superoxide radical scavenging activity. Values are means±SD, n = 3. (E) ADH activity. a: p ﹤ 0.05; b: p ﹤ 0.01 versus positive control.
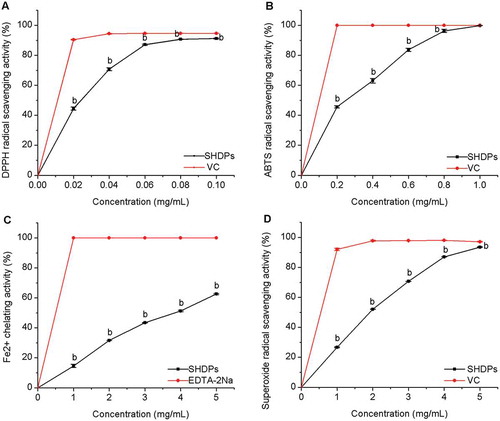
shows that the ABTS free radical scavenging of SHDPs enhanced rapidly with increasing concentrations. The scavenging activity against ABTS free radical was 99.9% at the concentration of 1 mg/mL. The ABTS scavenging activity of SHDPs is approximately equal to that of 1 mg/mL ascorbic acid. The IC50 value of SHDPs calculated by Origin (Version 8.0) was 0.26 mg/mL in the tested concentrations range. The results showed that SHDPs had a remarkable scavenging activity against the ABTS free radical.
The dose–response curve of SHDPs on the ferrous ion chelating capability is shown in Fig.5C. At the concentration of 5 mg/mL, the scavenging activity was 62.6%. The results indicated that SHDPs exhibited moderate chelating activity at the concentration of 5 mg/mL. The IC50 value of SHDPs was 3.86 mg/mL. FeCitation2+, as a powerful pro-oxide, can form violet-coloured complexes in the existence of ferrozine. However, violet-coloured complexes become lighter on account of disrupting complex formation in the presence of antioxidant and EDTA.[Citation44] The excessive ferrous ions in a biological system can lead to DNA damage.[Citation45] FeCitation2+ chelating ability would be somewhat beneficial in protecting against oxidative damage and eliminating DNA damage.
Superoxide anion radical is important for antioxidation works.[Citation19] Figure 5D shows the scavenging effect of SHDPs on superoxide radical in a dose-dependent manner. At the concentration of 5 mg/mL, the scavenging activity was 93.5%, which could bear comparison with that of VC. The IC50 value is 1.93 mg/mL.
Taking into account the complicated mechanism of antioxidant activity, one assay was insufficient to determine the potential antioxidant. Therefore, we selected four ways to evaluate the antioxidant activities of SHDPs in our studies. The results indicated that SHDPs have good biological activities. The bioactivity of polysaccharide could be related to the type of polysaccharide as well as to other factors, for instance, molecular weight, conformation, monosaccharide composition, isolation methods, glycosidic linkage, and extraction method.[Citation16] In this study, the SHDPs possess a molecular weight of 4570 kDa; the large molecular weight might contribute to its bioactivities.
Effect of SHDPs on ADH activity
The activation rate of ADH activity is shown in . The improvement of ADH activity could be affected by SHDPs obtained from SHDP residues. The ADH activity significantly increased with the concentrations (5–15 mg/mL) increasing. The maximum activation rate was (43.3 ± 3.1)% at 15 mg/mL of polysaccharide concentration. The activation rate declined dramatically when the sample concentration was over 15 mg/mL. It is similar to the report of Cheng Zhan et al.[Citation46] This could be because the viscosity of the sample increased with mass concentration, and the contact between the enzyme and the substrate reduced, and then the activation of ADH changed. ADH is a key enzyme in ethanol metabolism, which converts ethanol into acetaldehyde (ethanal). Acetaldehyde was oxidized to generate acetic acid by aldehyde dehydrogenase (ALDH), and then converted to CO2 and water through Kreb’s cycle and will eventually be discharged. This is one of the important metabolic pathways of alcohol. The high enzyme activity of ADH can quicken the catabolism rate of alcohol, reduce the amount of accumulation in the body, and prevent alcoholism. The rate of alcohol metabolism was influenced by enzyme activity.
Conclusion
A polysaccharide named SHDP with an average Mw of 4570 kDa was obtained from spent H. dulcis peduncles by using alkali treatment. SHDP is a heteropolysaccharide with a small number of contents of protein (3.6%) and uronic acid (2.3%), which is mainly composed of rhamnose, fucose, arabinose, xylose, mannose, glucose, and galactose in a molar ratio of 3.04:1:8.55:8.51:3.80:19.85:9.59 by GC with a Mw of 4570 kDa. NMR analysis revealed that SHDPs possessed a complex structure and contained 6)-Glcp-(1→, 3)-Rhap-(1→, 4)-Xylp-(1→, 3)-Fucp-(1→, Xylp-(1→, and Araf-(1→. FTIR analysis showed the characteristic absorption peaks of the polysaccharide. The assays of antioxidant activity in vitro indicated excellent DPPH radical scavenging activity (91.2%), strong ABTS radical scavenging activity (99.9%), moderate chelating ferrous ions activity (62.6%), and high superoxide radical scavenging activity (93.5%) in a dose-dependent manner. The activation rate of ADH was (43.3 ± 3.1)% at 15 mg/mL of SHDPs concentration. Further studies on other activities of polysaccharide from H. dulcis are in progress.
Acknowledgement
The authors would like to thank Mr Cheng Qian (University of Nevada, Reno, NV, USA) for his critical reading of the manuscript.
Funding
Financial support was received from National Natural Science Foundation of China (No. 41372245).
Additional information
Funding
References
- Wang, M.C.; Jiang, C.X.; Ma, L.P.; Zhang, Z.; Cao, L.; Liu, J.; Zeng, X.X. Preparation, Preliminary Characterization and Immunostimulatory Activity of Polysaccharide Fractions from the Peduncles of Hovenia dulcis. Preparation, Preliminary Characterization and Immunostimulatory Activity of Polysaccharide Fractions from the Peduncles of Hovenia dulcis. Food Chemistry 2013, 138, 41–47.
- Wang, Y.L.; Han, Y.; Fan, Y.G. Study on Value of Hovenia dulcis Thunb as a Food I. Analyses of Nutritional Elements. Nature Product Research and Development 1994, 6, 89–92.
- Liu, Y.; Qiang, M.L.; Sun, Z.G.; Du, Y.Q. Optimization of Ultrasonic Extraction of Polysaccharides from Hovenia dulcis Peduncles and their Antioxidant Potential. International Journal of Biological Macromolecules 2015, 80, 350–357.
- Wang, M.C.; Zhu, P.L.; Jiang, C.X.; Ma, L.P.; Zhang, Z.J.; Zeng, X.X. Preliminary Characterization, Antioxidant Activity In Vitro and Hepatoprotective Effect on Acute Alcohol-induced Liver Injury in Mice of Polysaccharides from the Peduncles of Hovenia dulcis. Food and Chemical Toxicology 2012, 50, 2964–2970.
- Wang, J.H.; Xu, J.L.; Zhang, J.C.; Liu, Y.; Sun, H.J.; Zha, X.Q. Physicochemical Properties and Antioxidant Activities of Polysaccharide from Floral Mushroom Cultivated in Huangshan Mountain. Carbohydrate Polymer 2015, 131, 240–247.
- Sevag, M.G.; Lackman, D.B.; Smolens, J. The Isolation of the Components of Streptococcal Nucleoproteins in Serologically Active Form. The Journal of Biological Chemistry 1938, 124, 0425–0436.
- Han, X.Q.; Chan, B.C.L.; Dong, C.X.; Yang, Y.H.; Ko, C.H.; Yue, G.G.L.; Chen, D.; Wong, C.K.; Lau, C.B.S.; Tu, P.F.; Shaw, P.C.; Fung, K.P.; Leung, P.C.; Hsiao, W.L.; Han, Q.B. Isolation, Structure Characterization, and Immunomodulating Activity of a Hyperbranched Polysaccharide from the Fruiting Bodies of Ganoderma sinense. Journal of Agricultural and Food Chemistry 2012, 60, 4276–4281.
- Wang, Y.F.; Zhang, M.; Ruan, D.; Shashkov, A.S.; Kilcoyne, M.; Sevage, A.V.; Zhang, L.N. Chemical Components and Molecular Mass of Six Polysaccharides Isolated from the Sclerotium of Poria cocos. Carbohydrate Polymer 2004, 339, 327–334.
- Dubios, M.; Gilles, K.A.; Hamilton, J.K.; Rebers, P.A. Colorimetric Method for Determination of Sugars and Related Substances. Analytical Chemistry 1956, 28, 350–356.
- Yang, H.; Yin, T.T.; Zhang, S.T. Isolation, Purification and Characterization of Polysaccharides from Wide Morchella esculenta (L.) Pers. International. Journal of Food Properties 2015, 18, 1385–1390.
- Li, F.; Cui, S.H.; Zha, X.Q.; Bansal, V.; Jiang, Y.L.; Asghar, M.N.; Wang, J.H.; Pan, L.H.; Xu, B.F.; Luo, J.P. Structure and Bioactivity of a Polysaccharide Extracted from Protocorm-like Bodies of Dendrobium huoshanense. International Journal of Biological Macromolecules 2015, 72, 664–672.
- Bradford, M.M.; A Rapid and Sensitive Methode for the Quantitation of Microgram Quantities of Proiteins Utilizing the Principle of Protein-dye Binding. Analytical Biochemistry 1976, 72, 248–254.
- Blumenkrantz, N.; Asboe-Hansen, G. New Method for Quantitative Determination of Uronic Acids. Analytical Biochemistry 1973, 54, 484–489.
- Wang, J.H.; Luo, J.P.; Zha, X.Q. Structural Features of a Pecticpolysaccharide from the Stems of Dendrobinm nobile Lindl. Carbohydrate Polymer 2010, 81, 1–7.
- Chen, T.L.; Zhang, M.; Li, J.L.; Surhio, M.M.; Li, B.B.; Ye, M. Structural Characterization and Hypoglycemic Activity of Trichosanthes Peel Polysaccharide. LWT-Food Science and Technology 2016, 70, 55–62.
- Chen, R.Z.; Tan, L.; Jin, C.G.; Lu, J.; Tian, L.; Chang, Q.Q.; Wang, K. Extraction, Isolation, Characterization and Antioxidant Activity Ofpolysaccharides from Astragalus membranaceus. Industrial Crops and Products 2015, 77, 434–443.
- Xie, J.H.; Xie, M.Y.; Nie, S.P.; Shen, M.Y.; Wang, Y.X.; Li, C. Isolation, Chemical Composition and Antioxidant Activities of a Water-soluble Polysaccharide from Cyclocarya paliurus (Batal.) Iljinskaja. Food Chemistry 2010, 119, 1626–1632.
- Needs, P.W.; Selvendran, R.R. Avoiding Oxidative Degradation during Sodium Hydroxide/Methyl Iodide-mediated Carbohydrate Methylation in Dimethyl Sulfoxide. Carbohydrate Research 1993, 245, 1–10.
- Liu, Y.; Du, Y.Q.; Wang, J.H.; Zha, X.Q.; Zhang, J.B. Structural Analysis and Antioxidant Activities of Polysaccharideisolated from Jinqian Mushroom. International Journal of Biological Macromolecules 2014, 64, 63–68.
- Xu, W.T.; Zhang, F.F.; Luo, Y.B.; Ma, L.Y.; Kou, X.H.; Huang, K.L. Antioxidant Activity of a Water-soluble Polysaccharide Purified from Pteridium aquilinum. Carbohydrate Research 2009, 344, 217–222.
- Cheng, H.; Feng, S.; Jia, X.; Li, Q.; Zhou, Y.; Ding, C. Structural Characterization and Antioxidant Activities of Polysaccharides Extracted from Epimedium acuminatum. Carbohydrate Polymer 2013, 92, 63–68.
- Manivasagan, P.; Sivasankar, P.; Venkatesan, J.; Senthilkumar, K.; Sivakumar, K.; Kim, S.K. Production and Characterization of an Extracellular Polysaccharide from Streptomyces violaceus Mm72. International Journal of Biological Macromolecules 2013, 59, 29–38.
- Wu, Z.; Li, H.; Tu, D.W.; Yang, Y.; Zhan, Y. Extraction Optimization, Preliminary Characterization, and In Vitro Antioxidant Activities of Crude Polysaccharides from Finger Citron. Industrial Crops and Products 2013, 44, 145–151.
- He, L.; Ji, P.F.; Cheng, J.W.; Wang, Y.B.; Qian, H.; Li, W.Q.; Gong, X.G.; Wang, Z.Y. Structural Characterization and Immunostimulatory Activity of a Novel Protein-bound Polysaccharide Produced by Hirsutella sinensis Liu, Guo, Yu & Zeng. Food Chemistry 2013, 141, 946–953.
- Yao, Y.; Xue, P.; Zhu, Y.Y.; Gao, Y.; Ren, G.X. Antioxidant and Immunoregulatory Activity of Polysaccharides from Adzuki Beans (Vigna angularis). Food Research International 2015, 77, 251–256.
- Yu, Z.Y.; Liu, L.; Xu, Y.Q.; Wang, L.B.; Teng, X.; Li, X.G.; Dai, J. Characterization and Biological Activities of a Novel Polasaccharide Isolated from Raspberry (Rubus idaeus L.). Carbohydrate Polymer 2015, 132, 180–186.
- Mei, Y.X.; Zhu, H.; Hu, Q.M.; Liu, Y.Y.; Zhao, S.M.; Peng, N.; Liang, Y.X. A Novel Polysaccharide from Mycelia of Cultured Phellinus linteus Displays Antitumor Activity through Apoptosis. Carbohydrate Polymer 2015, 124, 90–97.
- Ma, G.X.; Yang, W.J.; Mariga, A.M.; Fang, Y.; Ma, N.; Pei, F.; Hu, Q.H. Purification, Characterization and Antitumor Activity of Polysaccharides from Pleurotus eryngii Residue. Carbohydrate Polymer 2014, 114, 297–305.
- Chien, R.C.; Yen, M.T.; Tseng, Y.H.; Mau, J.L. Chemical Characteristics and Anti-Proliferation Activities of Ganoderma tsugae Polysaccharides. Carbohydrate Polymer 2015, 128, 90–98.
- Fang, X.B.; Yin, X.X.; Yuan, G.F.; Chen, X.E. Chemical and Biological Characterization of Polysaccharides from the Bark of Avicennia marina. European Food Research and Technology 2015, 241, 17–25.
- Zhang, W.X.; Song, D.; Xu, D.; Wang, T.T.; Chen, L.; Duan, J.Y. Characterization of Polysaccharides with Antioxidant and Immunological Activities from Rhizoma Acori Tatarinowii. Carbohydrate Polymer 2015, 133, 154–162.
- Li, C.; Fu, X.; Huang, Q.; Luo, F.X.; You, L.J. Ultrasonic Extraction and Structural Identification of Polysaccharides from Prunella vulgaris and its Antioxidant and Antiproliferative Activities. European Food Research and Technology 2015, 240, 49–60.
- Dai, H.; Han, X.Q.; Gong, F.Y.; Dong, H.L.; Tu, P.F.; Gao, X.M. Structure Elucidation and Immunological Function Analysis of a Novel Β-glucan from the Fruit Bodies of Polyporus umbellatus (Pers.) Fries. Glycobiology 2012, 12, 1673–1683.
- Ge, Q.; Zhang, A.Q.; Sun, P.L. Structural Investigation of a Novel Water-soluble Heteropolysaccharide from the Fruiting Bodies of Phellinus baumii Pilat. Food Chemistry 2009, 114, 391–395.
- Sigida, E.N.; Fedonenko, Y.P.; Zdorovenko, E.L.; Konnova, S.A.; Shashkov, A.S.; Lgnatov, V.V.; Knirel, Y.A. Structure of Repeating Units of a Polysaccharide(S) from the Lipopolysaccharide of Azospirillum brasilense SR80. Carbohydrate Research 2013, 371, 40–44.
- Ding, X.; Feng, S.; Cao, M.; Li, M.T.; Tang, J.; Guo, C.X.; Zhang, J.; Sun, Q.; Yang, Z.R.; Zhao, J. Structure Characterization of Polysaccharide Isolated from the Fruiting Bodies of Tricholoma matsutake. Carbohydrate Polymer 2010, 81, 942–947.
- Malgorzata, P.; Komaniecka, L.; Zamlynska, K.; Szewczuk, A.T.; Choma, A. Structure of the O-specfic Polysaccharide from the Legume Endosymbiotic Bacterium Ochrobactrum cytisi Strain ESCT. Carbohydrate Research 2015, 413, 37–40.
- Souza, R.O.S.; Assreuy, A.M.S.; Madeira, J.C.; Chagas, F.D.S.; Parreiras, L.A.; Santos, G.R.C.; Mourao, P.A.S.; Pereira, M.G. Purified Polysaccharides of Geoffroea spinosa Barks Have Anticoagulant and Antithrombotic Activities Devoid of Hemorrhagic Risks. Carbohydrate Polymer 2015, 124, 208–215.
- Jing, Y.S.; Huang, L.J.; Lv, W.J.; Tong, H.; Song, L.Y.; Hu, X.Q.; Yu, R.M. Structural Characterization of a Novel Polysaccharide from Pulp Tissues of Litchi chinensis and its Immunomodulatory Activity. Journal of Agricultural and Food Chemistry 2014, 62, 902–911.
- Hokputsa, S.; Gerddit, W.; Pongsamart, S.; Inngjerdingen, K.; Heinze, T.; Koschella, A.; Harding, S.; Paulsen, B.S. Water-soluble Polysaccharides with Pharmaceutical Importance from Durian Rinds (Durio zibethinus Murr.): Isolation, Fractionation, Characterisation and Bioactivity. Carbohydrate Polymer 2004, 56, 471–481.
- Li, X.; Han, W.; Mai, W.; Wang, L. Antioxidant Activity and Mechanism of Tetrahydroamentoflavone in Vitro. Natural Product Communications 2013, 8, 787–789.
- Liu, J.; Luo, J.G.; Ye, H.; Sun, Y.; Lu, Z.X.; Zeng, X.X. In Vitro and In Vivo Antioxidant Activity of Exopolysaccharides from Endophytic Bacterium Paenibacilluspolymyxa EJS-3. Carbohydrate Polymer 2010, 82, 1278–1283.
- Sun, Y.X.; Kennedy, J.F. Antioxidant Activities of Different Polysaccha-Ride Conjugates (Crps) Isolated from the Fruiting Bodies of Chroogomphis rutilus (Schaeff.: Fr.) O. K. Miller. Carbohydrate Polymer 2010, 82, 510–514.
- Zheng, Y.; Li, Y.; Wang, W.D. Optimization of Ultrasonic-assisted Extraction and In Vitro Antioxidant Activities of Polysaccharides from Trametes orientalis. Carbohydrate Polymer 2014, 111, 315–323.
- Zha, X.Q.; Xiao, J.J.; Zhang, H.N.; Wang, J.H.; Pan, L.H.; Yang, X.F.; Luo, J.P. Polysaccharides in Laminaria japonica (LP): Extraction, Physicochemical Properties and Their Hypolipidemic Activities in Diet-induced Mouse Model of Atherosclerosis. Food Chemistry 2012, 134, 244–252.
- Cheng, Z.; Yang, Y.D.; Kuang, Q.R.; Sun, B.J.; Ren, D.F.; Lu, J. Optimized Extraction of Lentinus edodes Peptides and Effects on Facilitating Alcohol Matebolism In Vitro. Journal of Chinese Institute of Food Science and Technology 2015, 15, 93–102.