ABSTRACT
Portulaca oleracea L. (P. oleracea) has been used as a Chinese herbal medicine for the treatment of antipyretic, anti-scorbutic, antiseptic, antispasmodic, diuretic, anthelmintic, and urinary disorders. However, pharmacological studies are necessary to provide a scientific basis to substantiate its effectiveness. In this study, the antimicrobial effect and its mechanism of the flavonoids extract from P. oleracea. (POFE) were investigated. The antibacterial effects of POFE were tested on Enterobacter cloacae, Klebsiella pneumonia, Escherichia coli, Staphylococcus aureus, Salmonella enterica and Micrococcus luteus, and the results showed that some bacteria, especially Staphylococcus aureus, were sensitive to POFE. At the tested concentrations, POFE-treated cells displayed various apoptotic markers, such as reactive oxygen species (ROS) accumulation, membrane depolarisation, phosphatidylserine (PS) exposure, DNA fragmentation, and caspase-like protein, in a dose-dependently manner. Based on these data, we concluded that POFE entered the cytoplasm through the membrane pores of Staphylococcus aureus, and subsequently, two parallel reactions took place, namely increase in the level of ROS and DNA fragmentation, which caused cell death.
Introduction
Portulaca oleracea L. (P. oleracea), which is a widespread weed, is an edible plant and belongs to a genus of succulent annuals commonly distributed all over the world, including Turkey, the United States, Africa, China, Japan and the Middle East.[Citation1] It has been proved to be rich in α-linolenic acid and β-carotene. Also, it has been revealed that it is a healthy food for patients with cardiovascular diseases.[Citation2] Further, it is listed by the World Health Organisation (WHO) as one of the most commonly used medicinal plant. Many investigations have demonstrated that this plant exhibits a wide range of pharmacological effects, such as antiviral effects for the treatment of viral hepatitis and diabetes management,[Citation3] muscle relaxant activity,[Citation4] analgesic effect,[Citation3] anti-inflammatory effect,[Citation5,Citation6] anti-oxidant effect,[Citation7] anticancer effect,[Citation8] antibacterial effect,[Citation9,Citation10] and wound healing effect.[Citation11] It is applied in the Arabian Peninsula as an antiseptic, anti-scorbutic, and antispasmodic.[Citation1] It is used in China as an anti-bacterial agent (was praised as “natural antibiotics”) against Escherichia coli, Shigella sonnei, proteusbacillus vulgaris, Bacillus subtilis, Staphylococcus aureus etc.[Citation12–Citation14] In our preliminary work, the experimental data demonstrated that the crude flavonoids extract from P. oleracea (POFE) mainly inhibited S. aureus. However, the detailed mechanism underlying the antibacterial effect of POFE has not been elucidated so far.
Apoptosis is a genetically regulated form of programmed cell death that is essential to the development and long-term viability of multicellular organisms. Apoptosis occurs in response to all kinds of intra- and extracellular stimuli and stresses. The cumulative effects of these physiological changes are designed to induce cell cycle arrest, halt DNA repair and homeostasis, inactivate apoptosis inhibitor proteins, facilitate ultrastructural modifications, and tag the dying cell, thereby achieving the deconstruction of biomolecular architecture, breakdown of cellular contents and marking for death.[Citation15] It has the advantage of occurring without the release of harmful substances.[Citation16] In addition, in our previous report, we found that Hypericum ascyron extract could induce gram-positive bacteria (Micrococcus luteus) cells death through apoptosis-like pathway.[Citation17,Citation18] Therefore, we investigated whether POFE can also induce S. aureus cells death by apoptosis-like pathway.
Materials and methods
Plant materials
All the samples of P. oleracea were collected from Beijing, People’s Republic (PR) of China in August 2015. All specimens were authenticated by Xiu-Mei Li (Feed Research Institute, Chinese Academy of Agricultural Sciences, Beijing, PR China). A voucher specimen was deposited at the Feed Research Institute, Beijing, PR China (Specimen number: P20150801).
Preparation of POFE
For the optimisation of POFE extraction conditions, the extraction process of POFE was carried out as follows: Briefly, the aerial parts of the dried plants were harvested and then selected through a 60-mesh sieve. Each sample powder (1.00 g) was accurately weighed and extracted using 30 mL 8.5:1.5 (v/v) ethanol-water solution by ultrasonic extraction for 30 min at 50°C. After centrifugation at 5000 × g for 10 min, the supernatant was evaporated by rotary evaporation at 40 ± 2°C, lyophilised, and stored at −20°C.
Determination of the total flavonoid content of POFE
The total flavonoid content of POFE was determined according to the method of Colorimetric aluminium chloride.[Citation19] POFE (0.01 g) was mixed with 1.0 mL of ethanol (85%, v/v), 1.0 mL of aluminium chloride (10%, w/v), 1.0 mL of sodium nitrite (5% g/v), 10.0 mL of sodium hydroxide (4% g/v) and 12 mL of 85% ethanol-water. After incubation at room temperature for 30 min, the absorbance was determined at 510 nm, and a calibration curve was prepared using rutin. The results are expressed in mg of rutin equivalents (mg RE)/g. The total POFE content is 845.27 ± 1.25 mg/g.
Strains
Escherichia coli (ATCC 25922), Enterobacter cloacae (ATCC 13047), Klebsiella pneumoniae (ATCC 10031), Staphylococcus aureus (ATCC 25923) and Salmonella enterica (ATCC 14028) were provided by Jianhua Wang (Feed Research Institute, Chinese Academy of Agricultural Sciences, Beijing, PR China). Micrococcus luteus (ATCC 10240) was provided by Xuegang Luo (Tianjin Key Lab of Industrial Microbiology; College of Biotechnology, Tianjin University of Science and Technology, Tianjin, PR China). All strains were maintained in Luria-Bertani (LB) agar (Hopebio, China) and stored at −20°C.
Minimum inhibitory concentration and minimum bactericidal concentration
MTT assay was used to measure the proliferation of bacterial cells.[Citation20] Briefly, bacterial cells (106 CFU/mL) were incubated in LB at 200 μL/well in 96-well microtiter plates. Various concentrations of POFE (5, 10,15, 20, 25, 30 mg/mL) were added to wells containing bacterial cells. After 24 h of incubation at 37 °C, each concentration was assayed in triplicate (n = 3). Twenty-four hours later, 10 μL of the MTT (5mg/mL) reagent was added to each well, and the plates were incubated for 1 h at 37 °C. Then, DMSO (100 μL) was added to terminate the reaction, and the plate was shaken slightly to redissolve the crystals formed. The absorbance of each well was measured using Synergy 4 microplate reader (BioTek Instruments, Winooski, VT, USA) (Li et al., 2015). All the results were expressed as the inhibition ratio of cell proliferation calculated as [(A-B)/A] ×100%, where A and B are the average numbers of viable bacterial cells of the control and samples, respectively. The MIC is the lowest concentration of the test sample required to inhibit any visible growth.
MBC, defined as the minimum concentration required to kill 99.9% of a bacteria inoculum, was determined by re-inoculating 20 μL of each culture medium from the microtiter plate wells onto the LB-agar plates. After 18 h of incubation at 37 °C, MBCs were determined by visually inspecting the agar plates for bacterial growth. All MIC and MBC measurements were performed at least in duplicate.
Analysis of intracellular reactive oxygen species accumulation
S. aureus cells were treated with POFE (2.5 mg/mL, 5.0 mg/mL and 10.0 mg/mL). After 1 h, the S. aureus cells were harvested via centrifugation at 12,000 rpm and resuspended in PBS. In order to analyse intracellular ROS accumulation, the cells were incubated with a fluorescent dye, 2,7-dichlorodihydrofluorescein diacetate (H2DCFDA) (10 μM) (Invitrogen, Molecular Probes, Eugene, OR, USA), which reacts with intracellular ROS, at 37 °C for 1 h. The samples were suspended in PBS, and their relative fluorescence intensity was analysed by the FACSCalibur flow cytometer (FACScan; BD Biosciences).[Citation21,Citation22]
DNA fragmentation assay
The 3′-OH of the DNA fragments in apoptotic cells were labelled and stained by terminal dexynucleotidyl transferase (TdT)-mediated dUTP nick end labelling method using an apoptosis in situ detection kit (Promega, Germany), according to the manufacturer’s instructions. Fluorescent microscopy (Olympus, FV1000, Japan) was used to capture the image of the fluorescein-labelled TUNEL-positive cells. Moreover, the TUNEL assay was performed to complement flow cytometric assay in order to demonstrate the apoptosis level more accurately than using flow cytometric assay alone. The cell nucleus was labelled in blue by Hoechst 33342 (Invitrogen, Molecular Probes, Eugene, OR), and the nick-ends were labelled in green. A merge between the nucleus (blue) and nick-end (green) labelling showed up as purple.[Citation15,Citation17,Citation18,Citation21,Citation22]
Analysis of DNA structure
S. aureus cells (106 CFU/mL) were incubated with POFE (2.5 mg/mL, 5.0 mg/mL and 10.0 mg/mL) for 1 h. DNA staining was performed using the DNA specific stain, Hoechst 33342 (Invitrogen, Molecular Probes, Eugene, OR), according to kit instructions. Counterstaining was performed using PI. After staining, cells were analysed using the FACSCalibur flow cytometer (FACScan; BD Biosciences).[Citation15]
Analysis of phosphatidylserine exposure
In order to detect phosphatidylserine (PS) exposure, we employed the Annexin V-FITC apoptosis kit (Invitrogen, Molecular probes, Eugene, OR). S. aureus cells were treated with POFE (2.5 mg/mL, 5.0 mg/mL and 10.0 mg/mL) for 1 h. S. aureus cells were harvested and resuspended in PBS. The cell suspensions were mixed with 100 μL of annexin V binding buffer, 5 μL of annexin V-FITC, and 5 μL of PI, and the mixtures were incubated at room temperature for 15 min. The cells were pelleted and resuspended in 400 μL of annexin V binding buffer, according to the manufacturer’s recommendations (Invitrogen, Molecular Probes, Eugene, OR). The analysis of the cells was done using the FACSCalibur flow cytometer (FACScan; BD Biosciences). Cells were sorted into living, necrotic, early apoptotic, and late apoptotic cells. The relative ratio of early and late apoptotic cells was counted for further comparison. This assay was repeated more than three times.[Citation15,Citation17,Citation18,Citation21,Citation22]
Analysis of caspase-like proteins
In order to detect a homolog of eukaryotic caspase, the CaspACE FITC-VAD-FMK In Situ Marker (Promega, Fitchburg, WI, USA) was employed. S. aureus cells were harvested by centrifugation at 12,000 rpm and washed with PBS. The suspensions were stained with the pan-caspase inhibitor FITC-VAD-FMK (2.5 μM) and incubated at 37 °C for 30 min. The samples were centrifuged at 12,000 rpm and suspended in PBS. The relative percentages of fluorescent cells were analysed using the FACSCalibur flow cytometer (FACScan; BD Biosciences).[Citation15,Citation21,Citation22]
Analysis of membrane depolarisation
S. aureus cells were incubated with POFE (2.5 mg/mL, 5.0 mg/mL and 10.0 mg/mL) at 37 °C. One hour later, cells were collected, washed twice with cold PBS, and detected with Mitochondrial Membrane Potential and Annexin V Apoptosis Kit, according to the manufacturer’s recommendations (Invitrogen, Molecular probes, Eugene, OR). They were then analysed by FACScanto (BD Biosciences). Next, S. aureus cells were also stained with Rhodamine 123 (Sigma, Germany) for 30 min at 37 °C and analysed by FACScanto (BD Biosciences).[Citation17,Citation18]
Statistical analysis
All data were expressed as mean ± SE (standard error, SE), and MIC values were analysed using SPSS 17.0 statistical software package (SPSS Inc., Chicago, IL, USA).
Results and discussion
Evaluation of antibacterial activities
The antibacterial activity of flavonoids has been documented in several earlier studies.[Citation23,Citation24] In this work, the antibacterial activities of POFE were tested against pathogenic bacteria: Gram-positive bacteria (S. aureus and M. luteus) and Gram-negative bacteria (E.coli, E.cloacae, K. pneumoniae, S. enterica). Using the MTT method, the proliferation of bacterial cells was detected. POFE exhibited different levels of antibacterial activities against Gram-positive and Gram-negative bacteria. POFE showed obvious antibacterial activity in Gram-positive bacteria, especially S. aureus ().
Table 1. Antibacterial activity of POFE from P. oleracea.
Possible reasons for these results may be associated with the several structural differences between Gram-positive and Gram-negative bacterial cell walls, as the latter has an outer membrane and a unique periplasmic space.[Citation25] The resistance of Gram-negative bacteria against antibacterial substances is because of its outer hydrophilic membrane surface that is rich in lipopolysaccharide molecules, creating a barrier effect for a lot of antibiotic molecules. This composition makes the cell wall impermeable to lipophilic solutes, and the porins in the cell wall do not allow the penetration of high molecular mass hydrophilic solutes, with an exclusion limit of about 600 Da.[Citation26] Further, enzymes in the periplasmic space are capable of breaking down molecules introduced from the outside. Gram-positive bacteria do not possess such an outer membrane and cell wall structure. Antibacterial substances can easily destroy the bacterial cell walls and cytoplasmic membrane, resulting in leakage and coagulation of the cytoplasm.[Citation27] In our opinion, the lack of an outer lipopolysaccharide membrane in the Gram-positive bacteria could allow increased permeability of POFE into S. aureus cells. Therefore, POFE showed significant antibacterial activity in Gram-positive bacteria. Our data demonstrated the potential role of POFE as an antibacterial agent against S. aureus.
Intracellular ROS accumulation
ROS accumulation can induce apoptosis in eukaryotic cells.[Citation28] ROS generation and oxygen stress have also been reported as general antibacterial events caused by many antibacterial ingredients.[Citation29] Therefore, we first evaluated whether POFE induces ROS accumulation in S. aureus cells. When intracellular ROS are accumulated, H2DCFDA reacts with them and is reduced to fluorescent DCF. The cells treated with POFE (2.5 mg/mL, 5.0 mg/mL and 10.0 mg/mL) possessed an increase in fluorescence intensity relative to untreated cells, indicating that POFE induces ROS accumulation ().
Effects of POFE on apoptosis-like death of S. aureus cells
Recent research has provided evidence of apoptotic-like death in bacteria and demonstrated that the activation of the apoptotic pathway is involved in the function of antibacterial drugs, such as membrane depolarisation, DNA fragmentation, phosphatidylserine exposure and chromosome condensation.[Citation15] However, in our group, we first found that the compounds from a natural product, H. ascyron, could induce M. luteus (ATCC 10240) cells deaths through membrane-mediated apoptosis-like pathway.[Citation17,Citation18] One key mechanism involved in the function of antibacterial drugs is the activation of the apoptotic pathway. The results of Annexin V/PI double staining showed that the apoptosis rate of S. aureus cells increased with an increase in the concentration of POFE, in a dose-dependent manner. As demonstrated in and , only a small percentage of apoptotic cells were found in the negative group (0.1 ± 0.1%) after being incubated by the vehicle for 1 h. However, the percentage of apoptotic cells significantly increased from 0.2 ± 0.1% to 1.0 ± 0.2% after being treated with different doses of POFE. The apoptosis effects of POFE on S. aureus cells were further confirmed via TUNEL assay. TUNEL positive cells were almost undetectable in the control group, whereas TUNEL positive signal increased significantly in a dose-dependent manner in POFE-treated S. aureus cells (), demonstrating that fragmentation of S. aureus DNA occurs upon treatment with POFE. To monitor the structural state of the bacterial chromosome after POFE treatment, we employed the DNA-specific and conformation-sensitive dye, Hoechst 33342. POFE-treated S. aureus cells displayed different levels of apoptosis, as shown in and. In order to investigate whether POFE induced S. aureus death through caspase-like proteins, we employed FITC-VAD-FMK, a fluorescently labelled version of the pan-caspase substrate VAD (valine–alanine–glutamate). In POFE-treated S. aureus cells, fluorescence was increased compared to untreated cells (), indicating that POFE stimulated the activation of caspase-like protein(s). Taken together, these results suggest that POFE could dose-dependently induce S. aureus cells deaths through apoptosis-like pathway.
Figure 2. POFE-induce S. aureus cells apoptosis-like response. (A) and (B) Results of Annexin V/PI staining. Cells were sorted into living, necrotic, early apoptotic, and late apoptotic cells. (C) and (D). Hoechst 33342/PI double staining, Cells were sorted into living, apoptotic and dead cells. (E) Results of TUNEL assays. TUNEL positive cells (green channel). Hoechst 33342 (blue channel) is used to locate the nuclei of the cells. (F) Flow cytometric analysis of caspase-like response using FITC-VAS-FMK. S. aureus cells untreated with POFE were as the control.
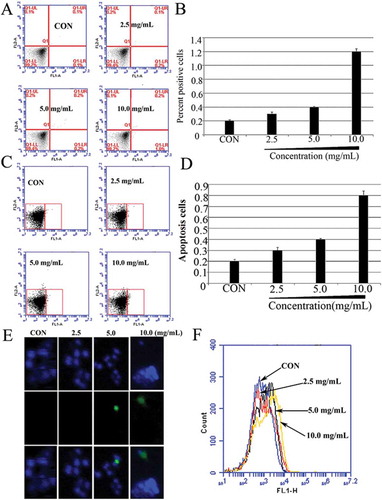
Effect of POFE on the membrane potential of S. aureus cells
ROS are natural byproducts of the metabolism of respiring organisms.[Citation30] Induction of ROS synthesis leads to the formation of highly reactive radicals that destroy the cells. Excess generation of ROS can attack bacterial cell membrane lipids and lead to a breakdown of bacterial cell membrane function.[Citation31] ROS generation and disruption of the membrane potential (ΔΨm) contribute to drug-induced death.[Citation32] The inhibition of bacterial growth by the formation of free radicals was also observed. In this investigation, Δψm was analysed by using Mitochondrial Membrane Potential/Annexin V Apoptosis Kit and Rhodamine 123, respectively. As demonstrated in , the results demonstrated that the membrane potential of S. aureus cells treated with POFE was lost, demonstrating that POFE induced irreversible apoptosis phenomenon by membrane-mediated apoptosis.
Figure 3. Membrane depolarisation analysis in POFE-treated S. aureus cells. (A) and (B) Mitochondrial Membrane Potential/Annexin V Apoptosis Kit analysed apoptosis based on PS translocation and changes in mitochondrial membrane potential. (C) Membrane potential (??m) was determined by using Rhodamine 123 staining and analysed by using flow cytometry. S. aureus cells untreated with POFE were as the control.
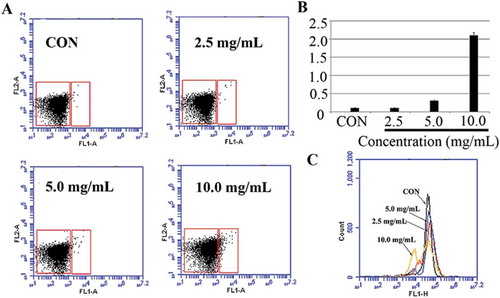
Conclusion
The antibacterial activity of POFE against S. aureus is due to its (POFE) entrance into the cytoplasm through membrane pores, subsequently causing two parallel reactions, namely increase in the level of ROS and DNA fragmentation, which cause cell death. In addition, the isolation and chemical characterisation of POFE are being performed by our group and represent a sustainable possibility of utilising the natural resources from P. oleracea. Finally, our data might provide a rational basis for the use of P. oleracea in clinical processes and throw light on the development of novel antibacterial drugs.
Funding
This work was financially supported by the Agricultural Science and Technology Innovation Program (ASTIP-FRI07), Special Fund for Agro-scientific Research in the Public Interest (201403047), and by China Postdoctoral Science Foundation (2016M591306).
Additional information
Funding
References
- Chan, K.; Islam, M.W.; Kamil, M.; Radhakrishnan, R.; Zakaria, M.N.; Habibullah, M.; Attas, A. The Analgesic and Antiinflammatory Effects of Portulaca oleracea L. Subsp. Sativa (Haw.) Celak. Journal of Ethnopharmacology 2000, 73, 445–451.
- Liu, L.; Howe, P.; Zhou, Y.F.; Xu, Z.Q.; Hocart, C.; Zhan, R. Flavonoids from Halostachys caspica and Their Antimicrobial and Antioxidant Activities. Journal of Chromatograph A 2000, 893, 207–213.
- Hu, L.F.; Xu, X.Y.; Wang, B.Q. Research and Utilization Situation of Portulaca oleracea L in China. Practical Journal of Medicine & Pharmacy 2003, 20, 315–316.
- Habtemariam, S.; Harvey, A.L.; Waterman, P.G. The Muscle Relaxant Properties of Portulaca oleracea are Associated with High Concentrations of Potassium Ions. Journal of Ethnopharmacology 1993, 40, 195–200.
- Xiang, L.; Xing, D.; Wang, W.; Wang, R.; Ding, Y.; Du, L. Alkaloids from Portulaca oleracea L. Phytochemistry 2005, 66, 2595–2601.
- Lee, A.S.; Kim, J.S.; Lee, Y.J.; Kang, D.G.; Lee, H.S. Anti-TNF-Α Activity of Portulaca oleracea in Vascular Endothelial Cells. International Journal of Molecular Sciences 2012, 13, 5628–5644.
- Boğa, M.; Hacıbekiroğlu, I.; Kolak, U. Antioxidant and Anticholinesterase Activities of Eleven Edible Plants, Pharmaceutical Biology 2011, 49(3), 290–295.
- Kamal Uddin, M.; Juraimi, A.S.; Eaqub Ali, M.; IsmailInt, M.R. Evaluation of Antioxidant Properties and Mineral Composition of Purslane (Portulaca oleracea L.) at Different Growth Stages. Journal of Molecular Sciences 2012, 13, 10257–10267.
- Elkhayat, E.S.; Ibrahim, S.R.M.; Aziz, M.A. Portulene, a New Diterpene from Portulaca oleracea L. Journal of Asian Natural Products Research 2008, 10, 1039–1043.
- Lei, X.; Li, J.; Liu, B.; Zhang, N.; Liu, H. Separation and Identification of Four New Compounds with Antibacterial Activity from Portulaca oleracea L, Molecules 2015, 20(9), 16375–163787.
- Rashed, A.N.; Afifi, F.U.; Disi, A.M. Simple Evaluation of the Wound Healing Activity of a Crude Extract of Portulaca oleracea L. (Growing in Jordan) in Mus Musculus JVI-1.Journal of Ethnopharmacology 2003, 88, 131–136.
- Zhang, L.H.; Qin, Y.H. Purslane Treatment of Limbs in 108 Cases, China’s Naturopathy 1999, 9(9),44.
- Zhang, X.J.; Qiao, Y.B.; Zh, Q.; Xia, Y.; Ch., J.; Wang, L. Experimental Studies on Antibiotic Functions of Portulaca oleracea L. in Vitro, Chinese Journal of Microecology 2002, 14(5), 277–279.
- Wang, L.; Liu, W.P. Antibacterial Effect of Portulacaoleracea L. Chinese Journal of Traditional Veterinary Science 2007, 3, 5–6.
- Dwyer, D.J.; Camacho, D.M.; Kohanski, M.A.; Callura, J.M.; Collins, J.J. Antibiotic-Induced Bacterial Cell Death Exhibits Physiological and Biochemical Hallmarks of Apoptosis. Molecular Cell 2012, 46, 561–572.
- Edinger, A.L.; Thompson, C.B. Death by Design: Apoptosis, Necrosis and Autophagy, Current Opinion in Cell Biology 2004, 16(6), 663–669.
- Li, X.M.; Luo, X.G.; Si, C.L.; Wang, N.; Zhou, H.; Li, K.; Ma, N.; Zhang, T.C. The Extract of Hypericum ascyron L. Induces Bacterial Cell Death through Apoptosis Pathway. Journal of Ethnopharmacology 2015a, 166, 205–210.
- Li, X.M.; Luo, X.G.; Si, C.L.; Wang, N.; Zhou, H.; He, J.F.; Zhang, T.C. Antibacterial Active Compounds from Hypericum ascyron L. Induce Bacterial Cell Death through Apoptosis Pathway. European Journal of Medicinal Chemistry 2015b, 96, 436–444.
- Mubo, A.; Sonibare Oluwafunmilola, T.; Aremu Patricia, N.; Okorie, A. Antioxidant and Antimicrobial Activities of Solvent Fractions of Vernonia cinerea (L.) Less Leaf Extract, Health Sciences 2016, 16(2), 629–639.
- Wang, X.; Wu, Y.C.; Xia, S.P.; Gao, Y.; Li, C.X. Experimental Study on the Application of MTT Colorimetric Method in Counting Live Escherichia coli. Journal of Luzhou Medicine College 2002, 25, 291–293.
- Yun, D.G.; Lee, D.G. Antibacterial Activity of Curcumin via Apoptosis-Like Response in Escherichia Coli, Applied Microbiology and Biotechnology 2016, 100(12), 5505–5514.
- Choi, H.; Hwang, J.S.; Lee, D.G. Coprisin Exerts Antibacterial Effects by Inducing Apoptosis-Like Death in Escherichia coli, IUBMB Life 2015, 68(1), 72–78.
- Süzgec¸-Selcuka, S.; Birteksözb, A.S. Flavonoids of Helichrysum Chasmolycicumand Its Antioxidant and Antimicrobial Activities. South African Journal of Botany 2011, 77, 170–174.
- Liu, H.; Mou, Y.; Zhao, J.; Wang, J.H.; Zhou, L.G.; Wang, M.G.; Wang, D.Q.; Han, J.G.; Yu, Z.; Yang, F.Y.; Zhou, L.G. Flavonoids from Halostachys caspica and Their Antimicrobial and Antioxidant Activities, Molecules 2010, 15(11), 7933–7945.
- Duffy, C.F.; Power, R.F. Antioxidant and Antimicrobial Properties of Some Chinese Plant Extracts. International of Journal Antimicrobial Agents 2001, 17, 527–529.
- Gangou E-Pi Eboji, J.; Eze, N.; Djintchui, A.N.; Ngameni, B.; Tsabang, N.; Pegnyemb, D.E. The In-Vitro Antimicrobial Activity of Some Traditionally Used Medicinal Plants against Beta-Lactam-Resistant Bacteria. Journal of Infection Developing Countries 2009, 3, 671–680.
- Gao, Y.; Van Belkum, M.J.; Stiles, M.E. The Outer Membrane of Gram-Negative Bacteria Inhibits Antibacterial Activity of Brochocin-C. Applied and Environmental Microbiology 1999, 65(10), 4329–4333.
- Perrone, G.G.; Tan, S.X.; Dawes, I.W. Reactive Oxygen Species and Yeast Apoptosis. Biochimica Et Biophysica Acta (Bba)-Molecular Cell Research 2008, 1783, 1354–1368.
- Nordberg, J.; Arner, E.S.J. Reactive Oxygen Species, Antioxidants, and the Mammalian Thioredoxin System. Free Radical Biology and Medicine 2001, 31, 1287–1312.
- Kim, J.S.; Kuk, E.; Yu, K.N.; Kim, J.H.; Park, S.J.; Lee, H.J. Antimicrobial Effects of Silver Nanoparticles. Nanomedicine 2007, 3, 95–101.
- Ninganagouda, S.; Rathod, V.; Singh, D.; Hiremath, J.; Singh, A.K.; Mathew, J. Growth Kinetics and Mechanistic Action of Reactive Oxygen Species Released by Silver Nanoparticles from Aspergillus niger on Escherichia coli. Biomed Research International 2014, 2014, 1–9.
- Ye, R.S.; Xu, H.Y.; Wan, C.X.; Peng, S.S.; Wang, L.J.; Xu, H.; Aguilar, Z.P.; Xiong, Y.H.; Zeng, Z.; Wei, H. Antibacterial Activity and Mechanism of Action of 3-Poly-L-Lysine. Biochemical and Biophysical Research Communications 2013, 439, 148–153.