ABSTRACT
Dairy products remain valuable components of human diet due to their balanced nutritive value and pleasant flavour. In this study, triacylglycerols (TAGs) from anhydrous milk fats were hydrolysed by Lipozyme-435 and Novozyme-435 and later on analysed by using ultra performance liquid chromatography coupled with quadrupole time-of-flight mass spectrometry. Results revealed that the percentages of TAGs (CN 28–34) and TAGs (CN 36–42) with at least two short-chain fatty acids and with short- and medium-chain fatty acids were respectively decreased after 24 h of enzymatic hydrolysis. On the other hand, TAGs (CN 44–54) with at least two long-chain fatty acids were found to increase in both Lipozyme-435- and Novozyme-435-treated anhydrous milk fat (AMF). Meanwhile, the melting and crystallization profiles of both Lipozyme-435- and Novozyme-435-treated AMF were modified and significantly different when compared with the untreated AMF.
Introduction
Milk fat is the third main source of lipid for human nutrition.[Citation1] It is widely acknowledged that milk fat imparts excellent flavour and superior mouthfeel to milk products.[Citation1,Citation2] Milk fat is an important ingredient in bakery and confectionery industry technology. To increase the use of milk fat as food ingredient, several technologies such as acyl exchange of the fatty acids on the triacylglycerols (TAGs) backbone have been incorporated into the manufacturing processes of food.[Citation3] Milk fat contains a complex fatty acid composition resulting in a mixture of TAGs with different physical and chemical properties.[Citation4–Citation6] Fatty acids in milk fat occur in a heterogeneous composition due to differences in chain length, their degree of saturation, and their binding position on the glycerol backbone.[Citation6–Citation8] These differences have resulted in variations in the physical and chemical properties[Citation8–Citation10] of milk fat; for example, the wide range differences in melting temperatures among milk products which have been found to occur between −40°C and 40°C[Citation4,Citation9–Citation14] are a typical result of heterogeneity of milk fat composition. The diversity of fatty acids has also enabled the modification of functional properties of milk and milk products thus bringing a variety of products in the market.[Citation12,Citation15]
The complexity of milk fat is compounded by the numerous number of fatty acids which in total sum almost up to 400[Citation14,Citation16] resulting in the generation of thousands of different kinds of TAGs.[Citation15,Citation17] Modification of milk fat composition is becoming a popular means for improving both the nutritional and the functional properties of food in the modern food industry.[Citation16,Citation18] Among the approaches include the enrichment of hydrolysed milk fat fraction with conjugated linoleic and trans-vaccenic acids[Citation17,Citation19] and enrichment of anhydrous milk fat in polyunsaturated fatty acid residues from linseed and rapeseed oils through enzymatic interesterification.[Citation1] Enzymatic hydrolysis of the TAGs to free fatty acids and glycerol is another approach for maximizing the production of short-chain fatty acids (C4:0-C8:0) for the enhancement of flavour development in milk products.[Citation17,Citation19]
During hydrolysis of milk fat, lipase plays a significant role by cleaving the bond between the fatty acid chain and the glycerol backbone. Depending on the type of lipase, the final product is a milk fat with improved flavour. To obtain this result, lipase with a characteristic ability of cleaving the fatty acid glycerol bond at sn_1,3 positions is highly recommendable.[Citation18,Citation20] However, up to date the application of lipase that cleaves only at sn_1,3 positions to our knowledge is still a challenge, due to the fact that it influences the unintended cleavage of other long-chain fatty acids which have little or nothing to do with the development of flavour. Long-chain saturated fatty acids, such as stearic acid, are primarily located at sn_1 position; likewise unsaturated fatty acids, such as oleic and linoleic acids, are located at sn_3 position.[Citation19,Citation21] Coincidentally, the short-chain fatty acids that are targeted for the development of flavour such as butyric, caproic, and octanoic acids are also located at sn_1,3 positions of a TAG molecule.[Citation18,Citation20,Citation22] For this reason, controlled hydrolysis of TAGs by careful selection of enzyme and timing enzyme reaction is essential in the development of desirable flavour. This implies that some of the TAGs will be partially hydrolysed while others will still remain intact. The intended outcome mainly relies on the cleavage of only those fatty acids that are responsible for flavour development. However, from the available literature, very little has been reported about the properties of TAGs in milk fat after enzymatic hydrolysis. On the other hand, studies conducted by Neff and Byrdwell[Citation22,Citation23] revealed that the oxidative stability of soya bean oil was partly dependent on the TAGs composition and their structure.
During hydrolysis of milk fat, the melting and crystallization profiles are modified due to cleavage especially of short-chain fatty acids, which eventually results in improvement of physical and chemical properties of food. Many other properties such as texture, mouthfeel, and rheology that depend on crystallization of milk fat are also affected by the enzymatic hydrolysis of milk fat.[Citation23,Citation24] The high melting fractions can be used as shortening in puff pastry in order to impart a desirable butter flavour or inhibit fat bloom in chocolate or as a substitute for cocoa butter in confectionary products, while the low melting fractions can be added in biscuits, short breads, and cold spreadable butter.[Citation16,Citation18] The rheological properties of fats are generally functions of the solid fat content (SFC) and other factors such as size, shape, number of the crystals, and clusters of TAG composition in milk fat.[Citation23,Citation24]
Up to date, a little amount of information concerning the impact of enzymatic hydrolysis of TAGs on milk fats has been reported. Therefore, it is against this background that the present study was aimed at characterizing the properties of TAGs after controlled enzymatic hydrolysis of milk fat for 24 h by using Lipozyme-435 and Novozyme-435. Specifically, the study quantified TAGs and their physical properties especially their crystallization and melting profiles after hydrolysis. It is hoped that this study would find applications in the development of dairy products and other food commodities for improvement of flavour and other functional properties.
Materials and methods
Anhydrous milk fat (AMF) with moisture content of 0.14% was a gift from Shanghai Kerry Oil & Grains Company Ltd. The immobilized lipases (Lipozyme-435 (≥20,000 U/g) from Rhizomucor miehei and Novozyme-435 (≥5000 U/g) from Candida antarctica) were gifts from Novozyme (Shandong) Innovation & Business Center, China. The OPO (1, 3-dioleoyl-2-palmitoylglycerol) and OOO (tri-olein) (≥95%) TAG standards were obtained from Sigma-Aldrich Chemical Co. Ltd. (St. Louis, MO). n-Hexane, acetonitrile, and iso-propanol were of High Performance Liquid Chromatography (HPLC) grade. All other solvents were of the analytical or HPLC grade.
Fatty acid analysis
Fatty acids were determined by converting the free fatty acids released into their Fatty acid methyl esters (FAME) form. Briefly the sample was prepared as follows: A 50 mg sample of milk fat was added in 2 mL of n-hexane, then 0.5 mL 2 N KOH of methanol solution was added. The mixture was vigorously vortexed for 2 min. To remove excess water, sodium thiosulfate was also added. The mixture was centrifuged at 10,000 rpm for 10 min. The supernatant was passed through a 0.45-μm membrane filter. A 0.5-μL sample was injected into Shimadzu GC-2010 (Tokyo Japan) equipped with an Flame Ionization Detector (FID) detector, with a capillary column, PEG-20M 30 m i.d. 0.32 mm × 1.0 μm. The initial column temperature was set at 100°C for 3 min before ramped to 180°C for 8 min at a rate of 10°C/min and then maintained at this temperature for 1 min. Then the temperature was ramped to 240°C for 20 min at a rate of 3°C/min and this temperature was maintained for 9 min. Temperature for the injector and detector was set at 250°C. Carrier gas (N2) was maintained at 110.0 kPa and the flow rate was 3 mL/min while the flow rate of H2 gas was 47 mL/min. The air flow rate was set at 400 mL/min and the split ratio was set at 1:12. Fatty acids were identified by comparing retention times on the chromatogram with those exhibited by the 40 fatty acids standards used in the experiments.
Performance of milk fat hydrolysis reactions
Hydrolysis of AMF with Lipozyme-435 and Novozyme-435 was carried out at 55°C in a 125-mL Erlenmeyer flask as described in detail in our previous study.[Citation20,Citation22]
Ultra pressure liquid chromatography analysis
The TAGs in hydrolysed milk fat were identified and quantified by a Waters Acquity Ultra Pressure Liquid Chromatography (UPLC) system coupled to Waters Qu-ToF Premier mass spectrometer using an ACQUITY UPLC BEH C18 analytical column (i.d. 2.1 × 50 mm, 1.9 μm). An amount of 10-mM ammonium acetate was introduced into the mobile phase as an electrolyte. The efficient separation of TAGs was carried out by two mobile phases: A (acetonitrile/isopropyl alcohol 1:9 v/v) and B (40% acetonitrile) at 300 μL/min. Initially, 70% of mobile phase A was used and maintained at this level for 1 min, then allowed to reach 87% in 30 min and held for 1 min, then returned back to the initial 70% in 1 min and equilibrated for 4 min. Temperature of the sample chamber was set at 20°C while the column temperature was set at 45°C. An amount of 1.0 μL was set as dead volume and each sample was analysed in triplicate.
Mass spectrometry conditions
The exactive mass spectrometer operated in the positive ion mode was performed on a Waters Qu-ToF Premier mass spectrometer with a mass range of 200–1500 m/z in 1 s duration. High-purity nitrogen was used as a nebulizer and drying gas was set at a constant flow rate of 1.5 mL/min. The source parameters were: 400°C desolvation temperature, 100°C source temperature, 3.5 V capillary voltages, and 20 V sampling cone voltages. Mass Spectrometry/Mass Spectometry analysis was set at collision energy of 35 V. The sodium format was used previously to calibrate the instrument and the lock mass spray for precise mass determination was set by leucine enkephalin in the positive ion mode. All the data were acquired and processed by Mass Lynx V4.1 software.
Melting and crystallization profile conditions
Melting and crystallization profiles of AMF, Lipozyme-435-, and Novozyme-435-treated AMF were determined by using differential scanning calorimetry (DSC Q2000 V24.9 Build 121, TA Instruments, New Castle, DE). In order for the analysis to take place, the system was purged with nitrogen gas at 20 mL/min during the analysis, and also nitrogen was used to serve as a refrigerant to cool the system. Indium, eicosane, and dodecane standards were used during calibration. The samples in the ranges (5–8 mg) were hermetically sealed in an aluminium pan, heated to 80°C, and held for 5 min to erupt completely the previous crystal structure, then allowed to cool at −40°C for 5 min. This treatment enabled the recording of melting profiles from the initial −40°C to 80°C through heat treatment at the rate of 10°C/min. An empty aluminium pan was used as a reference and each sample test was conducted in duplicate.
Powder X-ray diffraction analysis
The polymorphic forms of the AMF and hydrolysed products were determined by powder X-ray diffraction using a D8 Advance diffractometer (Bruker, Germany; λk = 1.54056 Å, 40 kV, 40 mA) equipped with a Vantec (Bruker, Karlsrube, Germany), using Cu-Ka with Ni filter. Diffraction patterns were recorded with 2θ in the range of 5‒50°C at a scan rate of 4°/min. The analysis was performed isothermally at 5°C, after the tempering period. Each sample test was determined in triplicate.
SFC analysis
The SFC analyses of AMF, Lipozyme-435-, and Novozyme-435-treated AMF were performed by using AM4000MQC (Oxford, Oxford shire, UK) low-resolution nuclear magnetic resonance (NMR). Approximately 2.5 mL of melted sample was placed in NMR tubes before kept at 80°C for 30 min, and then tempered at 0°C for 90 min, and finally 30 min at each 5°C intervals measuring temperature. The SFC was determined from 0°C to 40°C temperature range. All sample test observations were carried out in triplicate.
Statistical analysis
SPSS statistical software (v. 19.0, IBM SPSS, Chicago, IL) was applied in data analysis and means ± standard deviations were reported. Box plots were obtained by application of Origin 8.5 (Origin Lab, North Ampton, MA).
Results and discussion
Fatty acids composition
According to results, 18 fatty acids were identified in the range 0.25–33.93 mol/100 mol. Those were butanoic acid (1.96 mol/100 mol), caproic acid (1.58 mol/100 mol), caprylic acid (1.04 mol/100 mol), capric acid (2.67 mol/100 mol), lauric acid (5.04 mol/100 mol), myristic acid (13.31 mol/100 mol), pentadecanoic acid (1.07 mol/100 mol), palmitic acid (34.74 mol/100 mol), palmitoleic acid (1.69 mol/100 mol), margaric acid (0.40 mol/100 mol), stearic acid (10.42 mol/100 mol), oleic acid (22.43 mol/100 mol), linoleic acid (1.21 mol/100 mol), α-linolenic acid (0.51 mol/100 mol), γ-linolenic acid (0.61 mol/100 mol), stearidonic acid (1.00 mol/100 mol), arachidic acid (0.13 mol/100 mol), and gadoleic acid (0.19 mol/100 mol). Lauric, myristic, palmitic, stearic, and oleic acids were the most abundant fatty acids. The high amount of those fatty acids was also reported by others[Citation25,Citation26] who studied lipid composition analysis of milk fats from different mammalian species as potential for use as human milk fat substitutes and milk fat rancidity, respectively.
Triacylglycerols
A Qu-ToF MS with Electro Spray Ionization (ESI) is a rapid and unambiguous tool for determination of TAG profiles composition, and TAGs are detected mostly as sodiated molecules [M + Na]+. The OPO and OOO standards with sodiated [M + Na]+ adducts of 881 and 907 were respectively used. All the TAGs in AMF, Lipozyme-435-, and Novozyme-435-treated AMF were observed as sodiated [M + Na]+ and ammoniated [M + NH4]+ adducts.
presents TAGs and their relative percent peak area for AMF, Lipozyme-435-, and Novozyme-435-treated AMF. As shown in the table, it is clearly evident that the hydrolysis of the milk fat by the two lipases changed the distribution of TAG. The percentages of TAGs (carbon number CN 28–34) with at least two short-chain fatty acids and TAGs (CN 36–42) with some short- and medium-chain fatty acids generally decreased after 24 h of hydrolysis by both Lipozyme-435- and Novozyme-435-treated AMF. and show chromatograms and TAGs identified in Lipozyme-435- and Novozyme-435-treated AMF, respectively. The increase in the percentages of TAGs (CN 44–54) with at least two long-chain fatty acids was also observed in both Lipozyme-435- and Novozyme-435-treated AMF. These observations imply that the degree of hydrolysis was dependent on the number of carbon atoms on the fatty acid chains. Thus, hydrolysis preferentially affected TAGs containing esterified short-chain fatty acids; as a consequence of the loss of short-chain fatty acids on TAGs, there is an increase in the relative percentages of long-chain fatty acids on TAGs.[Citation24,Citation27]
Table 1. Triacylglycerol obtained in AMF and when AMF was treated with Lipozyme-435 and Novozyme-435 for 24 h.
Figure 1. Chromatograms and TAGs identified from Lipozyme-435- and Novozyme-435-treated AMF in 24 h.
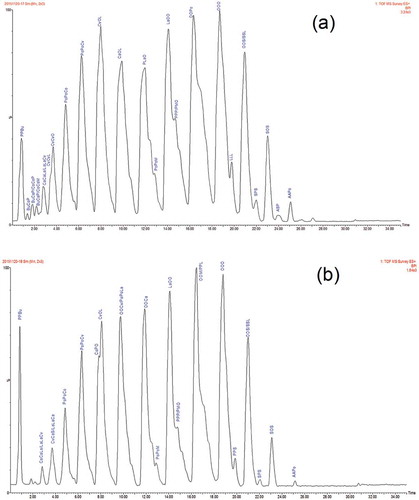
The major TAGs found in Lipozyme-435-treated AMF were PoPoCo, PoPoCy, CyOL, CaOL, PLaO, LaOO, PPP/PMO, OOPo, OOO, and OOS/SSL. These TAGs have a higher influence on the physical and functional properties of the product[Citation25,Citation28] than the rest of the TAGs due to their high percentage level. (a–d) shows MS/MS spectra of the four major TAGs obtained by Lipozyme-435-treated AMF. These TAGs were: CyOL (11.06 ± 0.14%), CaOL (10.69 ± 0.32%), OOPo (15.23 ± 0.32%), and OOO (10.32 ± 0.10%). The parent and daughter ions play an important role in the identification of TAGs. For instance, the daughter ions of CyOL (with parent ion m/z 767.58) were [Cy-L]+ (m/z 463.32), [Cy-O]+ (m/z 465.40), and [O-L]+ (m/z 601.51). The fragments formations were respectively due to the neutral loss of caprylic, linoleic, and oleic acids along with ammonia.
Figure 2. Some of major triacylglycerol mass spectra obtained when Lipozyme-435 was treated with AMF in 24 h.
aAMF: anhydrous milk fat.
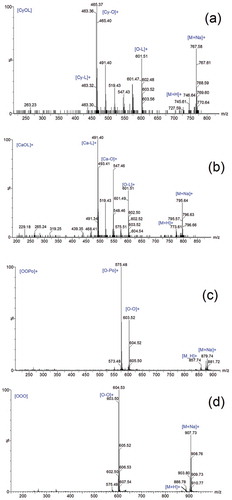
also shows the major TAGs found in Novozyme-435-treated AMF and these were: PoPoCo, PoPoCy, CoPO, CyOL, OOCy, OOCa, LaOO, PPP/PMO, OOM/PPL, OOO, and OOS/SSL. (a–d) shows the MS/MS spectra of the four major TAGs found in the Novozyme-435-treated AMF. These TAGs were OOCy (11.20 ± 0.32%), OOCa (9.41 ± 0.20%), OOM/PPL (16.54 ± 0.44%), and OOO (13.57 ± 0.26%). The parent and daughter ions detected as (OOO) m/z 907.71 and [O-O]+ (m/z 603.48), respectively, signified tri-olein. The high amount of OOM/PPL (16.54 ± 0.44%) found in the Novozyme-435-treated AMF could be the reason that led to decreasing oxidative stability in the previous study[Citation20,Citation22] as compared to Lipase-435-treated AMF (OOPo). It was found that TAGs comprising fatty acid with one or more double bonds were less stable than those with one or no double bonds. Similarly, lipids containing a higher amount of linoleic acid could be more unstable than the rest due to the high possibility of being oxidized at the double bond position. Our results support previous findings by Neff and Byrdwell[Citation22,Citation23] in which it was revealed that the oxidative stability of Soy bean oil (SBO) was partly dependent on the TAG composition and their structure.
Melting and crystallization profiles
(a, b) shows the melting and crystallization profiles of AMF, Lipase-435-, and Novozyme-435-treated AMF. Results revealed that the melting points of AMF occurred in the range of temperature between −38.36°C and 36.61°C (). The melting profile exhibited a broad shoulder between −38.36°C and −2.80°C, sharp shoulder between −2.80°C and 9.67°C, a very long sharp shoulder between 9.67°C and 20.29°C, and finally a broad shoulder again between 20.29°C and 36.61°C. Milk fat contains different TAGs which consist of short, medium-, and long-chain fatty acids. The broad range of melting temperature in the study could be attributed to the heterogeneity in fatty acid composition in the AMF [Citation9,Citation11,Citation12,Citation14] However, there were changes in melting profiles when Lipase-435- and Novozyme-435-treated AMF were applied in the experiments (). In Lipozyme-435-treated AMF, the melting curve depicted a broad shoulder that was observed between −38.62°C and −16.20°C, followed by a longer but broad shoulder between −3.60°C and 23.47°C, thereafter maintained another shoulder between 23.47°C and 31.43°C, and finally the shoulder was found between 31.43°C and 37.80°C. Previously, Tunick and Malin [Citation26,Citation29] found that the fresh cow milk mozzarella samples had a lower melting temperature in the range of −30°C and 11°C, followed by a short peak shoulder between 7°C and 8°C, a medium melting temperature with a tall peak at about 17°C, and finally a higher melting temperature was found in the range of temperature between 21°C and 38°C.
Figure 4. Melting and crystallization profiles obtained by AMF and when Lipozyme-435 and Novozyme-435 were treated with AMF in 24 h.
Part (a) represents melting profiles while (b) represents crystallization profiles.
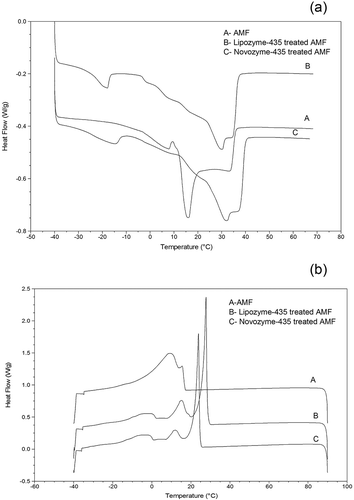
On the other hand, the Novozyme-435-treated AMF melting temperature curve exhibited a broad peak shoulder between −38.89°C and −11.56°C, followed by broader peak shoulder between −11.56°C and 22.67°C, then a peak shoulder between 22.67°C and 33.82°C, and finally a short broad peak between 31.43°C and 40.45°C. It is clear from the results that the sharp shoulder of melting temperature observed between −2.80°C and 9.67°C in AMF decreased suddenly to −38.62°C and −16.20°C and −38.89°C and −11.56°C, respectively, when AMF was treated with Lipozyme-435 and Novozyme-435. This implies that both Lipozyme-435- and Novozyme-435-treated AMF contained TAGs with a higher composition of saturated short- and medium-chain fatty acids than the untreated AMF since it has been previously found out that the melting point of the fat decreases with decreasing chain length and increasing degree of unsaturation of the fatty acids in milk fat.[Citation27,Citation30] The long sharp shoulder observed in AMF between 9.67°C and 20.29°C was modified into broad melting temperature ranges for Lipase-435- and Novozyme-435-treated AMF. The long broad ranges of temperature imply that the AMF contained TAGs with medium- and long-chain fatty acids. To our surprise, the final melting temperature depicted by the Novozyme-435-treated AMF (40.45°C) was higher than those for AMF (36.61°C) and Lipozyme-435-treated AMF (37.80°C). This implies that the Novozyme-435-treated AMFs contained a higher percentage of long-chain fatty acids than the rest which might be the reason for the high melting temperature observed. In a previous study, Rao et al. [Citation28,Citation31] investigated the melting profile of coconut oil triglycerides with a stearic acid level of 2% and modified lipids containing varying levels of stearic acid and found that increase in the level of stearic acid substitution in coconut oil triglycerides resulted in a gradual corresponding shift to higher melting temperatures. In addition, molecular composition with long-chain fatty acids imparts higher melting and crystallization temperatures than shorter chain fatty acids.[Citation32]
shows crystallization curves of AMF, Lipozyme-435-, and Novozyme-435-treated AMF. The crystallization curve for AMF depicts a broad range between −34.96°C and 13.86°C and short arm between 13.86°C and 15.53°C. However, three crystallization regions were observed in both Lipozyme-435- and Novozyme-435-treated AMF. A broad range was found in the region between −36.02°C and 0.97°C, followed by a short shoulder between 7.04°C and 16.44°C, and finally a very long sharp shoulder between 16.44°C and 25.84°C in Lipozyme-435-treated AMF. On the other hand, Novozyme-435-treated AMF exhibited a broad range between −35.30°C and 0.92°C, followed by a short shoulder between 7.82°C and 19.90°C, and finally a very long sharp shoulder between 19.90°C and 29.71°C. The gradual increase in crystallization temperature is associated with an increase in long-chain saturated [Citation28,Citation31] and unsaturated fatty acids. The crystallization regions for both Lipozyme-435- and Novozyme-435-treated AMF were similar implying that both treatments introduced resulted in an increase in short- and medium-chain fatty acids and as consequences TAGs with long-chain fatty acids were increased but on the other hand, the differences in crystallization temperatures were due to variations in the proportions of the fatty acid composition.[Citation17,Citation19] Previously, it was found that long-chain saturated fatty acids (C16:0, C18:0, and C20:0) and medium-chain saturated fatty acids (C10:0, C12:0, and C14:0) were more concentrated in the high crystallization temperature peak region while long-chain unsaturated fatty acids (C18:1 t11, C18:1 ω-9) were more concentrated in the middle melting point fraction. On the other hand, short-chain fatty acids such as C6:0 and C8:0 were concentrated in the lower crystallization temperature region.[Citation17,Citation19] Haddad et al.[Citation29,Citation33] also revealed that the composition of TAGs is responsible for variation in the melting and crystallization behaviour as well as rheological properties of milk fat.
Polymorphism
shows the polymorphisms of AMF, Lipozyme-435-, and Novozyme-435-treated AMF. The AMF exhibited d-spacings at 13.28 Å, a wide angle at 4.57 Å which represents the β crystal form, and 3.82 Å which represents the βʹ form.[Citation30,Citation34] Changes in d-spacings were found after 24 h of hydrolysis for both Lipozyme-435- and Novozyme-435-treated AMF. This could be due to change in the distribution of fatty acids which in turn affected intersolubility properties within the TAGs.[Citation31,Citation35] After hydrolysis the d-spacings at 13.28 Å in AMF were reduced to 12.67 Å in both Lipozyme-435- and Novozyme-435-treated AMF. Thereafter, d-spacings shifted to β crystal form (4.58 Å) and βʹ crystal form (4.15 Å and 3.78 Å) in Lipozyme-435-treated AMF. On the other hand, d-spacings shifted to β crystal form (4.60 Å) and βʹ crystal form (4.15 Å and 3.79 Å) in Novozyme-435-treated AMF. It is clear from the results that enzymatic hydrolysis had played a significant role in changing β to βʹ, which was the most desirable crystal form in shortenings. Previously, it has been reported that the formation of small crystal nucleus and large surface area as a result of changing β to βʹ form enables the inclusion of air into shortenings thus improving the creaming properties of mixtures.[Citation30,Citation32,Citation34,Citation36]
Figure 5. Characteristic X-ray powder diffraction (XRD) patterns of the polymorphic forms found in AMF and when Lipozyme-435 and Novozyme-435 were treated with AMF in 24 h. The pattern was obtained from 5°C to 50°C at a rate of 4°C/min.
(a) AMF, (b) Lipozyme-435-treated AMF, and (c) Novozyme-435-treated AMF.
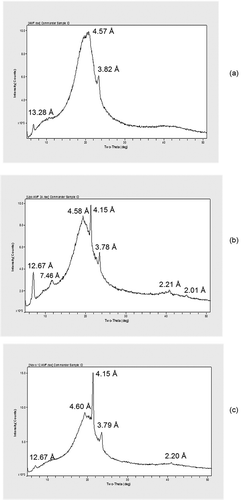
Solid fat content
The consistencies of AMF, Lipozyme-435-, and Novozyme-435-treated AMF were tested for SFC using NMR set at the range of 0‒35°C as shown in . The SFC is an important parameter used in monitoring milk fat and milk fat products at different temperatures to evaluate their suitability in food processing. In addition, the determination of SFC values at various temperatures is very useful when creating product’s physical attributes and predicting the behaviour of the final product.[Citation37] According to results of SFC in AMF, Lipozyme-435-, and Novozyme-435-treated AMF decreased with increasing testing temperature. As shown in , the SFC values of AMF obtained from 0°C to 15°C testing temperatures were higher than the Lipozyme-435-, and Novozyme-435-treated AMF. This phenomenon could be explained by the fact that AMF contained a higher proportion of TAGs composed of short-chain fatty acids than Lipozyme-435- and Novozyme-435-treated AMF. Higher SFC values were found in Novozyme-435-treated AMF than in Lipozyme-435-treated AMF. However, at the testing temperature range of 15‒25°C, the SFC values of AMF and Novozyme-435-treated AMF were approximately the same, though higher values were found in Lipozyme-435-treated AMF than AMF even at 30°C. Another interesting point that was noted was that when Lipozyme-435- and Novozyme-435-treated AMF were subjected at SFC testing temperature ranges of 15‒35°C, Novozyme-435-treated AMF was found with slightly lower values of SFC than Lipozyme-435-treated AMF. This observation could be explained by the fact that Novozyme-435-treated AMF had relatively higher percentage area of OOO (13.57 ± 0.26%) TAG than OOO (10.32 ± 0.10%) TAG of Lipozyme-435-treated AMF. In addition, Novozyme-435-treated AMF had higher relative percentage area of OOM/PPL (16.54 ± 0.44%) TAG than OOPo (15.23 ± 0.32%) TAG of Lipozyme-435-treated AMF. The composition of fatty acids in TAG molecule influenced the characteristics of lipids in the sample. Unsaturated fatty acids with one or more double bonds are usually in a liquid state at room temperature while saturated fatty acids are in a solid state at the same temperature. Since Novozyme-435-treated AMF comprised of a higher percentage of OOO and OOM/PPL TAGs, it is justifiable that they had lower SFC values than Lipozyme-435-treated AMF. Additionally, a higher proportion of TAGs with short-chain fatty acids were found in Lipozyme-435-treated AMF than in Novozyme-435-treated AMF (). In general, both Lipase-435- and Novozyme-435-treated AMF displayed elements of plasticity in the SFC range of 15–35%, thus their application in dairy products would regulate consistency.[Citation28,Citation31]
Conclusion
The TAG composition of both Lipozyme-435- and Novozyme-435-treated AMF was significantly changed after 24 h of controlled hydrolysis. The percentages of TAGs (CN 28–40) with some short- and medium-chain fatty acids decreased with corresponding increasing TAGs (CN 44–54) with long-chain fatty acids during the 24 h of controlled hydrolysis. The decrease in amount of TAGs with short-chain fatty acids and those with medium-chain fatty acids with increasing TAGs comprising long-chain fatty acids suggests selective enzymatic hydrolysis of TAGs. The difference in TAG results obtained by each Lipase-435- and Novozyme-435-treated AMF samples may be due to the enzyme specificity toward substrate. Both melting and crystallization profiles of Lipase-435- and Novozyme-435-treated AMF were different from AMF implying that enzymatic hydrolysis influenced the fatty acids composition of TAGs. The study has also revealed that Lipase-435- and Novozyme-435-treated AMF displayed elements of plasticity within SFC values implying that they could find application in the processing of dairy and dairy products. Finally, treatment of AMF with Lipase-435- and Novozyme-435 yielded βʹ form of crystals which is the most desirable crystal form. This would find application in shortenings since the formation of small crystal nucleus and large surface area enables the incorporation of more air into the shortenings and improvement of creaming properties.
Declaration of interest
The authors have declared no conflict of interest.
Acknowledgments
We are grateful to Shanghai Kerry Oils & Grains Industries for their provision of raw materials.
Funding
We are grateful to the National Natural Science Foundation of China for financial support (Grant No. 31401525), China Postdoctoral Science Foundation (Grant No. 2015M571666), and Collaborative Innovation Center of Food Safety and Quality Control in Jiangsu Province.
Additional information
Funding
References
- Aguedo, M.; Hanon, E.; Danthine, S.; Paquot, M.; Lognay, G.; Thomas, A.; Vandenbol, M.; Thonart, P.; Wathelet, J.P.; Blecker, C. Enrichment of Anhydrous Milk Fat in Polyunsaturated Fatty Acid Residues from Linseed and Rapeseed Oils through Enzymatic Interesterification. Journal of Agricultural and Food Chemistry 2008, 56, 1757–1765.
- Reddy, S.Y. Improving Plasticity of Milk Fat for Use in Baking by Fractionation. Journal of the American Oil Chemists’ Society 2010, 87, 493–497.
- Grall, D.S.; Hartel, R.W. Kinetics of Butterfat Crystallization. Journal of the American Oil Chemists’ Society 1992, 69, 741–747.
- Büyükbeşe, D.; Emre, E.E.; Kaya, A. Supercritical Carbon Dioxide Fractionation of Anhydrous Milk Fat. Journal of the American Oil Chemists’ Society 2014, 91, 169–177.
- Destaillats, F.; de Wispelaere, M.; Joffre, F.; Golay, P.A.; Hug, B.; Giuffrida, F.; Fauconnot, L.; Dionisi, F. Authenticity of Milk Fat by Fast Analysis of Triacylglycerols: Application to the Detection of Partially Hydrogenated Vegetable Oils. Journal of Chromatography A 2006, 1131, 227–234.
- Balcão, V.M.; Kemppinen, A.; Malcata, F.X.; Kalo, P.J. Modification of Butterfat by Selective Hydrolysis and Interesterification by Lipase: Process and Product Characterization. Journal of the American Oil Chemists’ Society 1998, 75, 1347–1358.
- Schenkel, P.; Samudrala, R.; Hinrichs, J. Thermo-Physical Properties of Semi-Hard Cheese Made with Different Fat Fractions: Influence of Melting Point and Fat Globule Size. International Dairy Journal 2013, 30, 79–87.
- Ayyildiz, H.F.; Topkafa, M.; Kara, H.; Sherazi, S.T.H. Evaluation of Fatty Acid Composition, Tocols Profile, and Oxidative Stability of Some Fully Refined Edible Oils. International Journal of Food Properties 2015, 18, 2064–2076.
- Badu, M.; Awudza, A.J. Determination of the Triacylglycerol Content for the Identification and Assessment of Purity of Shea Butter Fat, Peanut Oil, and Palm Kernel Oil Using Maldi-Tof/Tof Mass Spectroscopic Technique. International Journal of Food Properties 2017, 20, 1271–1280.
- Öğütcü, M.; Yılmaz, E. Characterization of Hazelnut Oil Oleogels Prepared with Sunflower and Carnauba Waxes. International Journal of Food Properties 2015, 18, 1741–1755.
- Arul, J.; Boudreau, A.; Makhlouf, J.; Tardi, R.; Bellavia, T. Fractionation of Anhydrous Milk Fat by Short-Path Distillation. Journal of the American Oil Chemists’ Society 1988, 65, 1642–1646.
- Fatouh, A.E.; Singh, R.K.; Koehler, P.E.; Mahran, G.A.; Metwally, A.E. Physical, Chemical and Stability Properties of Buffalo Butter Oil Fractions Obtained by Multi-Step Dry Fractionation. Food Chemistry 2005, 89, 243–252.
- Queirós, M.S.; Grimaldi, R.; Gigante, M.L. Addition of Olein from Milk Fat Positively Affects the Firmness of Butter. Food Research International 2016, 84, 69–75.
- Ramel, P.; Marangoni, A.G. Engineering the Microstructure of Milk Fat by Blending Binary and Ternary Mixtures of Its Fractions. RSC Advances 2016, 6, 41189–41194.
- Prado, G.H.C.; Khan, M.; Saldaña, M.D.A.; Temelli, F. Enzymatic Hydrolysis of Conjugated Linoleic Acid-Enriched Anhydrous Milk Fat in Supercritical Carbon Dioxide. The Journal of Supercritical Fluids 2012, 66, 198–206.
- Bugeat, S.; Perez, J.; Briard-Bion, V.; Pradel, P.; Ferlay, A.; Bourgaux, C.; Lopez, C. Unsaturated Fatty Acid Enriched Vs. Control Milk Triacylglycerols: Solid and Liquid TAG Phases Examined by Synchrotron Radiation X-Ray Diffraction Coupled with DSC. Food Research International 2015, 67, 91–101.
- Robinson, N.P.; MacGibbon, A.K.H. The Composition of New Zealand Milk Fat Triacylglycerols by Reversed-Phase High-Performance Liquid Chromatography. Journal of the American Oil Chemists’ Society 1998, 75, 993–999.
- Lopez, C.; Bourgaux, C.; Lesieur, P.; Riaublanc, A.; Ollivon, M. Milk Fat and Primary Fractions Obtained by Dry Fractionation: 1. Chemical Composition and Crystallisation Properties. Chemistry and Physics of Lipids 2006, 144, 17–33.
- Martínez-Monteagudo, S.I.; Khan, M.; Temelli, F.; Saldaña, M.D. Obtaining a Hydrolyzed Milk Fat Fraction Enriched in Conjugated Linoleic Acid and Trans-Vaccenic Acid. International Dairy Journal 2014, 36, 29–37.
- Nyyssölä, A.; Miettinen, H.; Kontkanen, H.; Lille, M.; Partanen, R.; Rokka, S.; Järvenpää, E.; Lantto, R.; Kruus, K. Treatment of Milk Fat with Sn-2 Specific Pseudozyma antarctica Lipase A for Targeted Hydrolysis of Saturated Medium and Long-Chain Fatty Acids. International Dairy Journal 2015, 41, 16–22.
- Ruiz-Gutierrez, V.; Barron, L.J.R. Methods for the Analysis of Triacylglycerols. Journal of Chromatography B: Biomedical Sciences and Applications 1995, 671, 133–168.
- Omar, K.A.; Gounga, M.E.; Liu, R.; Mlyuka, E.; Wang, X. Effects of Microbial Lipases on Hydrolyzed Milk Fat at Different Time Intervals in Flavour Development and Oxidative Stability. Journal of Food Science and Technology Mysore 2016, 53, 1035–1046.
- Neff, W.E.; Byrdwell, W.C. Soybean Oil Triacylglycerol Analysis by Reversed-Phase High-Performance Liquid Chromatography Coupled with Atmospheric Pressure Chemical Ionization Mass Spectrometry. Journal of the American Oil Chemists’ Society 1995, 72, 1185–1191.
- Wiking, L.; de Graef, V.; Rasmussen, M.; Dewettinck, K. Relations between Crystallisation Mechanisms and Microstructure of Milk Fat. International Dairy Journal 2009, 19, 424–430.
- Jahn, M.; Jahn, D. Milk Fat/Rancidity. In Handbook of Hydrocarbon and Lipid Microbiology Institut für Mikrobiologie, Technical University Braunschweig, Braunschweig, Germany,, Springer, 2010; 2377–2391.
- Zou, X.; Huang, J.; Jin, Q.; Guo, Z.; Liu, Y.; Cheong, L.; Xu, X.; Wang, X. Lipid Composition Analysis of Milk Fats from Different Mammalian Species: Potential for Use as Human Milk Fat Substitutes. Journal of Agricultural and Food Chemistry 2013, 61, 7070–7080.
- Fontecha, J.; Mayo, I.; Toledano, G.; Juáre, M. Use of Changes in Triacylglycerols during Ripening of Cheeses with High Lipolysis Levels for Detection of Milk Fat Authenticity. International Dairy Journal 2006, 16, 1498–1504.
- Tzompa-Sosa, D.A.; van Valenberg, H.J.F.; van Aken, G.A.; Bovenhuis, H. Milk Fat Triacylglycerols and Their Relations with Milk Fatty Acid Composition, DGAT1 K232A Polymorphism, and Milk Production Traits. Journal of Dairy Science 2016, 99, 3624–3631.
- Tunick, M.H.; Malin, E.L. Differential Scanning Calorimetry of Water Buffalo and Cow Milk Fat in Mozzarella Cheese. Journal of the American Oil Chemists’ Society 1997, 74, 1565–1568.
- Smiddy, M.A.; Huppertz, T.; van Ruth, S.M. Triacylglycerol and Melting Profiles of Milk Fat from Several Species. International Dairy Journal 2012, 24, 64–69.
- Rao, R.; Sankar, K.U.; Sambaiah, K.; Lokesh, B.R. Differential Scanning Calorimetric Studies on Structured Lipids from Coconut Oil Triglycerides Containing Stearic Acid. European Food Research and Technology 2001, 212, 334–343.
- Makeri, M.U.; Karim, R.; Abdulkarim, M.S.; Ghazali, H.M.; Miskandar, M.S.; Muhammad, K. Comparative Analysis of the Physico-Chemical, Thermal, and Oxidative Properties of Winged Bean and Soybean Oils. International Journal of Food Properties 2016, 19, 2769–2787.
- Haddad, I.; Mozzon, M.; Strabbioli, R.; Frega, N.G. Electrospray Ionization Tandem Mass Spectrometry Analysis of Triacylglycerols Molecular Species in Camel Milk (Camelus dromedarius). International Dairy Journal 2011, 21, 119–127.
- Shi, C.; Chang, M.; Liu, R.; Jin, Q.; Wang, X. Trans-Free Shortenings through the Interesterification of Rice Bran Stearin, Fully Hydrogenated Soybean Oil and Coconut Oil. International Journal of Food Engineering 2015, 11, 467–477.
- Danthine, S.; Lefébure, E.; Trinh, H.N.; Blecker, C. Effect of Palm Oil Enzymatic Interesterification on Physicochemical and Structural Properties of Mixed Fat Blends. Journal of the American Oil Chemists’ Society 2014, 91, 1477–1487.
- Reddy, S.Y.; Jeyarani, T. Trans-Free Bakery Shortenings from Mango Kernel and Mahua Fats by Fractionation and Blending. Journal of the American Oil Chemists’ Society 2001, 78, 635–640.
- Torbica, A.; Jambrec, D.; Tomić, J.; Pajin, B.; Petrović, J.; Kravić, S.; Lončarević, I. Solid Fat Content, Pre-Crystallization Conditions, and Sensory Quality of Chocolate with Addition of Cocoa Butter Analogues. International Journal of Food Properties 2016, 19, 1029–1043.