ABSTRACT
The objective of this study was to investigate the kinetic stability of lime essential oil emulsion using gum arabic (GA) and whey protein isolate (WPI) biopolymers as emulsifiers and stabilizers. Different solid compositions were evaluated: GA, GA/WPI (1:1) and WPI, as well as oil concentration (5.0, 7.5 and 10% w/w). Emulsions were prepared using an ultrasonic homogenizer. Viscosity, oil droplet size, zeta potential, pH and conductivity were measured to predict the emulsion stability over a period of 4 h (estimated time until complete drying in microencapsulation processes). Emulsion rheological behaviour and microstructure were characterized. All emulsions were stable during the time period analysed, and no destabilization characteristic was observed. The pH, conductivity and viscosity showed no significant difference (p > 0.05) over the 4-h period. Emulsions with WPI showed higher zeta potential values, confirming the biopolymer electrosteric stabilization. The combination of wall materials (GA/WPI) and the oil concentration increased the average droplet size. The results of the apparent viscosity at shear rate of 100 s−1 confirmed the emulsions stability. These values did not change significantly considering the fresh emulsions and emulsions after 4 h of preparation. The emulsifying biopolymers showed to be excellent natural stabilizers for lime essential oil emulsions, highlighting the results using WPI.
Introduction
Lime essential oil is widely used by the food, pharmaceutical and cosmetic industries due to its chemical and sensory characteristics.[Citation1] It is commonly used in products such as beverages, baked goods, sweets, desserts and ice cream.[Citation2] Lime essential oil is a complex mixture of chemical compounds divided into three classes: terpenes (75%), oxygenated complexes (12%) and sesquiterpenes (3%). It is composed of substances such as limonene, γ-terpene, citral, β-caryophyllene among others.[Citation2–Citation4] This essential oil is extremely volatile, and your encapsulation is an alternative to provide slow release of their bioactive compounds. The process of encapsulation begins with the preparation of stable emulsions using biopolymers and subsequent drying using spray-drier.
Figure 3. Microscopy images of emulsions prepared with GA and different essential oil concentration: (a) 5.0 g oil/100 g emulsion; (b) 7.5 g oil/100 g emulsion; (c) 10.0 g oil/100 g emulsion; (1) fresh and (2) after 4 h.
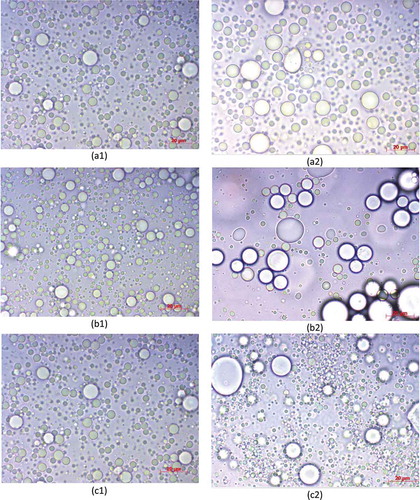
Figure 4. Apparent viscosity as function of shear rate for lime essential oil with GA (A), GA/WPI (B) e WPI (C).
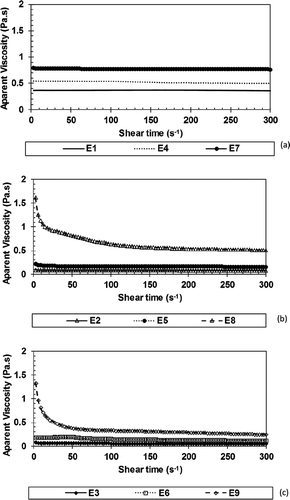
Emulsions can be defined as a mixture of two immiscible liquids, usually water and oil. Several products from food, pharmaceutical, chemical and other industries are characterized as emulsions.[Citation5] In foods, the emulsion behaviour can be defined in three parts: 1) the oil or fat within the emulsion droplet; 2) the interfacial material between lipid and aqueous phase; and 3) the aqueous phase itself. Food systems are extremely complex and may have different behaviours in different situations. Different compositions of the interfacial material, for example, may result in very different physical and chemical characteristics. Therefore, it is necessary to understand the characteristics of each system individually and collectively.[Citation6,Citation7] Stable emulsions are able to resist changes in their properties over a period of time. Physical and chemical processes can cause an emulsion to become unstable, causing destabilization processes such as agglomeration, creaming, coalescence, phase inversion, and others (physical instability), or oxidation and hydrolysis (chemical instability).[Citation7–Citation11] However, the basic mechanisms of emulsion stabilization are not accurately understood, particularly due to biopolymer structure complexity[Citation12,Citation13]
Biopolymers are widely used as emulsifying and stabilizing agents, for having good gelling and emulsifying capacity.[Citation14–Citation17] Stable emulsion production, using biopolymers such as gum arabic (GA) and whey protein isolate (WPI), is desirable and widely used in microencapsulation processes of food components.[Citation18–Citation24]GA is a mixture of anionic carbohydrates and some proteins, while WPI is a mixture of globular proteins. GA has good emulsifying properties due to the presence of highly branched proteins that are closely linked to the polysaccharide structure.[Citation24–Citation27] Globular proteins are quickly adsorbed onto the oil droplets surface during homogenization, facilitating the formation of small droplets.[Citation28,Citation29]
The homogenization process is directly related to the emulsion physical properties and is an important step in the study of microencapsulation processes.[Citation30] The process can be divided into two stages: the first in which the high shear stress leads to the drop deformation, increasing the surface area; and the second, the system stabilization by some emulsifying agent.[Citation31] In the literature, the most commonly used processes are mechanical stirring, ultrasonication and high pressure homogenization. Homogenization by ultrasound has been highlighted in food emulsion research[Citation15,Citation32–Citation35] and results showed to be an efficient method for obtaining emulsions with droplet size in micrometers or nanometers. The ultrasound homogenization mechanism is based on cavitation, in which high intensity ultrasound waves impact the liquid surface, forming high velocity jets, and are responsible for the droplet formation.[Citation30,Citation36,Citation37]
Emulsion plays an important role on efficiency and stability of the oil microparticles produced by encapsulation processes, specially spray drying. Some of the main properties to be evaluated are stability, viscosity, droplet size and solid concentration.[Citation38] Several works[Citation39–Citation43] on emulsions cite and study physical and chemical parameters that predict stability. Droplet size, zeta potential, surface tension, rheology, pH, conductivity, creaming rate and microstructure are examples of the main parameters used. Ensuring emulsion stability is extremely important for processes in the food and pharmaceutical industries, such as oil microencapsulation. From emulsion homogenization to complete drying, it is necessary that all its physical and chemical properties are maintained, so the microparticles quality is not compromised. Many research works on microencapsulation give special attention to the study of the emulsions stability as an interfering in powdered products final quality.[Citation34,Citation39–Citation43] This work aimed to study the different physical and chemical parameters of lime essential oil emulsions using GA and WPI biopolymers, evaluating the effect of lime oil concentration and wall material composition to predict the ideal conditions for water in oil emulsion stability. All parameters were evaluated in a 4-hour interval, as this time is sufficient for emulsion use in microencapsulation processes. The emulsions, in these cases, should remain stable between the emulsion preparation and drying steps.
Materials and methods
Lime essential oil was used as dispersed phase (Ferquima, Vargem Grande Paulista, São Paulo). Gum Arabic (Alland & Robert, France) and whey protein isolate (Alibra Ingredients Ltda, Campinas, Brazil) were used as emulsifying and stabilizing agents.
Experimental design
The lime essential oil emulsions were obtained using gum arabic (GA) and whey protein isolate (WPI) and different concentrations of lime essential oil, in relation to the total solids as shown in . This work was carried out based on a complete factorial experimental design, where were evaluated two variables: emulsifier and lime essential oil concentration, both in three levels (WPI, GA/WPI and GA) and (5.0, 7.5 and 10.0 g oil/100 g emulsion), respectively. Solids concentration (Emulsifier + oil) was 30.0 g/100 g emulsion.
Table 1. Experimental design to lime essential oil emulsions.
Emulsion preparation
The different formulations studied () were added to water for 24 h to complete biopolymer hydration and saturation. The lime essential oil was added to the biopolymer solution, and homogenization was carried out under ultrasound (Digital Sonifier 450, Branson Ultrasonic Corporation, USA) for 2 min using nominal power of 160 W. After homogenization, emulsions were placed in an ice bath until they reach room temperature (The temperature after homogenization was 46 ± 0.3°C).
Ph measurement
Emulsion pH measurement was carried out in triplicate using a PHS-3E pH meter (pHTek, Curitiba, Brazil). The equipment was calibrated with buffer solutions, and pH values were measured by inserting the electrode directly into the sample at 25°C.[Citation44,Citation45]
Electrical conductivity
The emulsion electrical conductivity measurement was determined in triplicate, using a benchtop conductivity meter (Hanna, model HI 8731, Portugal). The conductivity values were measured by inserting the electrode directly into the sample at 25°C.[Citation46]
Zeta potential
The surface charge density (zeta potential) of the biopolymers was determined by Electrophoretic Light Scattering using ZetaSizer Nano-ZS (Malvern Instruments Ltd., Worcestershire, UK). The emulsions were diluted in Milli-Q water (Millipore, Bedford, USA) to 2.0% (v/v), according to the equipment optimal detection range. The measurements were performed in duplicate (each measure with 10 runs or more) at 25°C.[Citation12]
Droplet size measurement
The Z-Average diameter was determined by dynamic light scattering (DLS) technology using Zetasizer Nano ZS (Malvern Instruments, UK). The emulsions were diluted in Milli-Q water (Millipore, Bedford, USA) to 2.0% (v/v), according to the equipment optimal detection range. The measurements were performed in duplicate (each measure with 5 runs or more) at 25°C.[Citation44,Citation47] The polydispersity index (SPAN) was determined according to Eq. (1).
where d(10), d(50) and d(90) are the diameters at 10%, 50% and 90% cumulative volume, respectively.
Analysis of rheological behaviour
Emulsion rheological behaviour and viscosity were evaluated using an oscillatory rheometer HAAKE RheoStress 6000 (Thermo Fisher Scientific) coupled to a temperature controller HAAKE UTM Controller (Thermo Fisher Scientific). The geometry used was plate–plate with a 34.997 mm diameter and a 1 mm GAP. The emulsions were evaluated immediately after preparation and after 4 h. Analyses were performed in triplicate at 25°C. The rheological behaviour was analysed by the deformation rate (0–300 s−Citation1) increase, decrease and increase. After removal of time dependence (thixotropic), the third curve was obtained. The third curve data were adjusted to rheological model Power Law (Eq. 3). The model adequacy was evaluated by the coefficient of determination (RCitation2), the residual mean square and the significance of the parameters (p < 0.05). Apparent viscosity of emulsions was studied at shear rate of 100 s−Citation1. The parameter was evaluated at this shear rate since it is the typical rate for food processes, such as the flow through tubes in industry and stirring and mastication processes.[Citation7]
where δ = shear stress (Pa); = shear rate (s−Citation1); k = consistency index (Pa.sn); n = flow behaviour index.
Optical microscopy
Optical microscopy was performed to assess the emulsion destabilization processes on a microstructural level. The analysis was performed after the emulsion preparation and after 4 h of storage, in an Axio Scope A1 optic microscope, Carl Zeiss (Germany), with a video camera attached. For this, an aliquot of emulsion was placed on the slide, covered with a cover slip, and examined with a 100× objective lens.[Citation48,Citation49]
Creaming index
The creaming index (CI) was determined by adding 5 mL of emulsion in plastic pots, immediately after preparation. After 4 h, the volume of the formed cream was measured and creaming percentage determined according to the Eq. (3):
where HC is the cream phase (upper phase volume) and HT is the initial emulsion height.
Statistical analysis
All treatments were manufactured in three repetitions. Analysis of variance (ANOVA) and rheological data adjustment to rheological models were performed using SAS software (Statistical Analysis System), version 9.1, licensed by the institution. Significant differences between the samples (p < 0.05) were assessed by the Scott–Knott mean comparison test.
Results and discussion
Ph and electrical conductivity
The pH is a very significant parameter because a significant change in its value may suggest chemical changes of the components present in the formulation. Thus, it can be inferred that the emulsions formed are stable systems and their components did not alter significantly over the analysed time period. The pH and conductivity of lime essential oil emulsions are shown in and , respectively. Food emulsions generally have pH in the range of 2.5 to 7.5.[Citation7] The variation in the oil amount did not influence the emulsion pH values, except for emulsions in which GA and WPI were used as emulsifier, which presented a slight increase in its value when oil concentration was increased from 5.0 to 7.5 g oil/100 g emulsion. The pH values showed no significant difference (p > 0.05) after 4 h. Silva et al.[Citation12] also found higher pH values for emulsions in which WPI was used as an emulsion stabilizer and emulsifying agent compared to the values of emulsions in which GA was used. The values found by the cited author are close to those found in this present work, around 5.0 for emulsions with GA and 6.2 for WPI emulsions, showing that pH is not influenced by the oil but by the polysaccharides used.
Electrical conductivity analysis showed reduced values with increasing oil concentration in the emulsion, when GA was used as emulsifier. In the emulsions in which GA/WPI and WPI were used, the conductivity value decreased only at the highest oil concentration. This behaviour can be explained by the poor conductive characteristic of the oil.[Citation50,Citation51] In addition, emulsions with lower electrical conductivity values were those where the GA/WPI combination was used. Higher for GA conductivity values were observed for emulsions with WPI. It can be seen that the emulsions with pure GA have higher conductivity values compared to the other treatments. Globular proteins exhibit large structure and higher capacity links with water molecules in its chain and free water increases the emulsion conductivities.[Citation52] Klein et al.[Citation53] studied the interaction between GA and WPI and observed that the conductivity values decrease when the two biopolymers are mixed. This observation can be explained by the cancellation of the biopolymer charges, reducing the solution conductivity. The increase in viscosity of solutions can cause a reduction in conductivity. Also, the process of cavitation and ultrasonic homogenization can cause ion aggregations, reducing the mobile ions of these systems and consequently the electrical conductivity.[Citation54] Conductivity values also did not present statistically significant alterations (p > 0.05) after 4 h. Variations in conductivity values can occur when there are creaming, sedimentation or phase inversion processes and sensitive alterations in the molecular structure of the formulation components. Conductivity is a parameter frequently used to determine emulsion stability during the storage period.[Citation55]
Zeta potential
According to Jayme et al.[Citation24] and Sari et al.,[Citation56] there are two types of emulsion stabilization processes: steric stability, when the emulsifier molecule adheres to the oil droplet surface, maintaining it stable; and electrostatic stability, when there is repulsion among the droplets due to the high surface charge, hindering the droplet agglomeration. Moreover, electrosteric stability can also occur, in which both types of stabilization processes are observed. The zeta potential is the most commonly used parameter to determine the electrostatic contribution to emulsion stability.[Citation5,Citation11,Citation12] Surface charges control possible system droplet–droplet interactions and contribute to the emulsion stability.[Citation57]
presents the results of zeta potential for the solutions (water + emulsifier) and the lime essential oil emulsion at 0 and 4 h. A significant difference in zeta potential values was observed after the homogenization process compared with the solution values, which were reduced, except for emulsions in which GA/WPI was used with oil concentrations of 5.0 and 7.5 g oil/100 g emulsion. O´Brien[Citation37] explains that the biopolymer chains may be broken due to the cavitation’s process. This chain breakage favours the increase in biopolymer surface charge. The decrease in zeta potential values in the emulsions in which GA and WPI were used can be explained by the reduction of biopolymer charges.
Table 2. Zeta potential by biopolymers solutions and emulsions at 0 and 4 h.
Emulsions E1, E3, E5, E6 and E9 showed no significant change (p > 0.05) in the zeta potential values after 4 h. This behaviour is the opposite to what occurred in E2, E4, E7 and E8 emulsions, which became less stable according to this parameter. The reduction in the zeta potential value, after a certain time, is especially common in emulsions, due to low oil polarity. Herculano et al.[Citation58] and Silva et al.[Citation39] report that emulsions are considered stable when the zeta potential values exceed ±30 mV. Zeta potential values from 0 to ±30 mV promote droplet attraction, leading to less stable emulsions.[Citation39,Citation49]
Therefore, the most electrostatically stable emulsions, according to this parameter, are emulsions in which WPI was used as a biopolymer, since they presented zeta potential above ±30 mV and there were no statistically significant changes after 4 h of storage. Proteins are known to be easily absorbed in the water-oil interface, promoting greater emulsion kinetic stability, especially by electrostatic repulsion, due to the high surface charge concentration. Protein also hinders the water adsorption in oil due to its low wet ability and long molecular chain.[Citation22,Citation28,Citation59,Citation60]
Gum arabic has less surface charge compared to whey protein isolate. Polysaccharides have the capacity to retain water causing system thickening, so even with the low charge concentration of the GA emulsion, they were stable, as confirmed by pH and conductivity data. It can be said that the gum arabic emulsifying properties are related to a steric mechanism.[Citation7,Citation61] In fact, the emulsions in which WPI was used showed the highest zeta potential values after preparation, and this value remained statistically the same after 4 h.
In emulsions with annatto oil, Silva et al.[Citation12] observed higher zeta potential values for WPI compared to samples with GA, as in this work. Xu et al.[Citation62] also observed zeta potential values lower than −30 mV in model WPI and sunflower oil emulsions. In orange oil emulsions with gum arabic at different concentrations, Mirhosseini et al.[Citation63] observed zeta potential values varying between −22.6 and −28.7 mV. The addition of GA reduces the charges around the emulsion droplets, becoming less electrostatically stable than emulsions with WPI. Sukhotu et al.[Citation64] observed the same behaviour in peanut oil emulsions.
Polydispersion index, oil droplet size and emulsions optical microscopy
PDI and oil droplet size values for the emulsions are shown in . The PDI values are related to the distribution homogeneity of oil droplet size, and the closer to zero, the more uniform is the distribution.[Citation46,Citation62] For all biopolymers used, there is an increase in the PDI with the increase in the oil concentration used. With the increase in the oil concentration, the droplets were not perfectly mono-disperse and the PDI values or deviation from the average size increased (). Increased PDI values may be associated with destabilization phenomena, such as emulsion oil droplet coalescence. However, the phenomenon was not enough to promote creaming or phase separation.
Table 3. Polydispersion index (PDI) and droplet size by emulsions at 0 and 4 hours.
The oil droplet size is an impact factor for predicting food emulsion stability.[Citation7] The average droplet size () is in the range of 0.282–1.306 µm for fresh emulsions; and of 0.256–1.968 µm for emulsions after 4 h of storage. The biopolymer influenced significantly (p < 10–Citation10) the average oil droplet size and WPI emulsions showed smallest average droplet size in both time intervals (0 and 4 h). When comparing the influence of oil concentration on the average droplet size, only the GA/WPI blend presented significant difference (p < 10–Citation8) compared with the other biopolymers. In the study of WPI emulsions by Kim and Morr[Citation64] and Bae and Lee,[Citation48] they also observed an increase in the oil droplet size by increasing the amount of oil in the emulsions. Taneja et al.[Citation22] observed a reduction of average soybean oil droplet size when the WPI concentration was increased in the emulsion. Dluzewska et al.[Citation23] observed an increase in droplet size with increasing oil concentration in gum arabic emulsions. The same author also did not observe significant increase in droplet size during storage.
When comparing the droplet size over time (0 to 4 h), we observed that there was a significant increase (p < 0.05) in the droplet size in the GA/WPI blend. When studying the stability of essential oil and WPI emulsions, Dluzewska et al.[Citation23] found no significant increase in droplet size over time. Microscopic images () show the increase in droplet size with increasing oil amount for the GA/WPI treatment and also confirm the increase in droplet size after 4 h. There is a tendency for the oil droplet size to increase with increased amount of oil in the emulsions, since the amount of oil is higher for a smaller amount of emulsifier available to bind to the oil surface.[Citation40,Citation48,Citation65] Garcia et al.[Citation66] observed a tendency of increasing average droplet size with increasing oil concentration in emulsions of Basil essential oil with gum arabic.
Rheology
The Power Law rheological model was that best fit to all the experimental data due to the mean square low value (E(%) < 4.49). In addition, this model presented significant (p < 0.05) parameters (k and n) for all treatments. This model has been extensively used to describe emulsion rheological behaviour.[Citation12,Citation17,Citation61,Citation67–Citation69] shows the Power Law model parameters. Except for the E8 and E9 emulsions, all emulsions presented a flow behaviour index (n) value very close to 1, which shows a tendency of such systems toward a Newtonian behaviour, in which the relation between shear stress and shear rate is linear and viscosity is constant, which can be evidenced by the rheograms shown in . E8 and E9 emulsions have a higher oil concentration and were produced using WPI as emulsifier (GA/WPI and WPI, respectively). The n values found in these two cases were lower, showing that in these emulsions the rheological behaviour deviates from the Newtonian behaviour and shear-thinning behaviour being more evident. This behaviour is shown in in which there is reduction in the apparent viscosity values with increasing shear rate.
Table 4. Correlation coefficient, residual mean square and Power Law model parameters for emulsions prepared with different biopolymers and lime essential oil.
Table 5. Apparent viscosity values at 100 s−Citation1.
The consistency index increased with the increasing oil concentrations within each emulsifier, presenting the highest value in the emulsions in which GA/WPI was used. The consistency index is a parameter that also informs about fluid viscosity. Therefore, according to Ìbanoglu,[Citation70] the consistency index increases with the solids content and with the increase in oil concentration. The parameters behaviour remained the same after 4 h, which is the time necessary for microencapsulation.
. shows the relationship between the apparent viscosity and shear rate. It can be noted in the that, except for E8 and E9 emulsions, all emulsions exhibit nearly constant viscosity values with the increase in shear rate that is behaviour of Newtonian fluids. In the E8 and E9 emulsions, there is reduction in apparent viscosity that is typical behaviour of pseudoplastic fluids. Ìbanoglu,[70] when studying the rheological behaviour of emulsions with milk protein isolate and different gum arabic concentrations, also found the pseudoplastic behaviour present in these emulsions. Moschakis et al.[72] verify that emulsions stabilized with low WPI concentrations, present a behaviour very close to Newtonian, while in the presence of high polysaccharide concentrations, such as gum arabic, he found that these systems exhibit a pseudoplastic behaviour. According to the author, this tendency toward pseudoplastic behaviour, as occurred in the E8 and E9 emulsions, is due to weak associative interactions and suggests the formation of a weak network around the droplets. Silva et al.[61] studied emulsions stabilized by different polysaccharides for green coffee oil microencapsulation. The author also found that the behaviour of these systems can vary between Newtonian and pseudoplastic. Jafari et al.[17] found that at concentrations above 4%, emulsions which had Newtonian behaviour start exhibiting a pseudoplastic behaviour.
The apparent viscosity () of the emulsions at shear rate of 100 s−Citation1 did not change significantly (p < 0.05) between the fresh emulsions and emulsions after 4 h of preparation, showing kinetic stability of these systems during the studied period. According to Silva et al.,[Citation12] rheological changes which may occur in the emulsions after preparation period are mainly associated with destabilization processes, since, in these processes, the oil is released into the continuous phase, altering the system rheological properties. Since oils have higher viscosity than water, the properties change. Viscosity values ranged from 0.791 to 0.7987 Pa s, for the lowest and highest emulsifier agent concentrations, respectively.
Creaming index
Creaming can be defined as a destabilization process due to the upward movement of the oil droplets since its density is lower than the continuous phase (water). Generally, this process occurs due to gravitational or centrifugal forces, which cause droplets to agglomerate, increase in size and accumulate at the top of the system.[Citation7,Citation71] In this study during the 4 h of storage, none of the samples exhibited a visible process of destabilization (creaming, phase separation or agglomeration). Even with the increase in oil concentration (), there was no emulsion creaming process in the 4-h interval. In the microencapsulation process, the emulsion must remain stable throughout the process, so that there is no interference in the powder properties, particularly as to the encapsulation efficiency reduction.
Moschakis et al.[Citation72] observed that sunflower oil emulsions with GA and WPI are stable and did not exhibit a creaming process over a week. Garcia et al.[Citation66] studied Basil essential oil emulsions with GA and found no system creaming process in 24 h. Tonon et al.[Citation40] observed that, even at high oil concentrations (30%), there was no oil phase separation in emulsions with flaxseed oil. In studies with flaxseed oil emulsions with WPI, Kuhn and Cunha[Citation59] did not observed creaming in 9 days of storage.
In order to have emulsion stability, there can be no creaming, flocculation or coalescence.[Citation26] The creaming index is a parameter which provides indirect information about the emulsion droplet agglomeration, and the larger the drops, the faster the creaming process.[Citation12,Citation71] Thus, it can be inferred that the emulsions of all lime oil concentrations and different biopolymers have small oil droplets which did not undergo agglomeration, which can lead to the creaming process, in the 4-hour period. That is, over the 4-hour period, the emulsion was visibly stable.
Conclusions
In this work, the evaluation of physicochemical properties indicated that these are related to the charges that were originated after application of ultrasound treatment. The treatments with WPI proved to have more surface charges than GA. These charges resulted in increases in pH values, increases in the zeta potential values, decreases the PDI values, Newtonian behaviour changes (n), and increases of the consistency index (k) values. The ultrasonic homogenization process favours breaking of the biopolymer chain and the increase in surface loads. These charges are concentrated around the droplets forming stable emulsions. The presence of oil and second biopolymer (GA) influences the electrostatic stability, and the effects on the physicochemical parameters are mitigated, as noted. Considering the emulsion stability between the time after preparation and feeding in the spay-dryer to produce microcapsules, the use of biopolymers with good emulsifying capacity was confirmed, highlighting the importance of this feature in essential oil encapsulation. The results show that for all biopolymer formulations, no phase separation during the first 4 hours of storage was observed. In all treatments, there was no significant change in pH and conductivity. Higher zeta potential values were found for WPI and higher oil concentration treatments due to the characteristics and functional groups present in the biopolymer and lime essential oil. The viscosity values do not change with time, demonstrating kinetic stability. All oil droplets were less than 2 μm in size. GA and WPI showed high emulsifying and stabilizing capacity in emulsions prepared with lime essential oil and can be used as wall material for microencapsulation systems. The results were satisfactory for all essential oil and biopolymer combinations, being more relevant in emulsions in which WPI was used as biopolymer.
Funding
The authors thank FAPEMIG (Minas Gerais State Foundation for Research Development, Brazil) (CAG-PPM-00318-11) and CNPq (National Council for Scientific and Technological Development, Brazil) (Grant Number: 448530/2014-7) for the financial support.
Additional information
Funding
References
- Rao, J.; McClements, D.J. Impact of Lemon Oil Composition on Formation and Stability of Model Food and Beverage Emulsions. Food Chemistry 2012, 134, 749–757.
- Gamarra, F.M.C.; Sakanaka, L.S.; Tambourgi, E.B.; Cabral, F.A. Influence on the Quality of Essential Lemon (Citrus aurantifolia) Oil by Distillation Process. Brazilian Journal of Chemical Engineering 2006, 23, 147–151.
- Santos, J.C. Dos. Avaliação Do Efeito Antimicrobiano De Óleos Essenciais Sobre Micro-Organismos Patogênicos Em Vôngole (Anomalocardia Brasiliana); Universidade Federal da Bahia, Salvador, Brazil, 2010.
- Cruz-Valenzuela, M.R.; Tapia-Rodriguez, M.R.; Vazquez-Armenta, F.J.; Silva-Espinoza, B.A.; Ayala-Zavala, J.F. Lime (Citrusaurantifolia) Oils. In Essential Oils in Food Preservation, Flavor and Safety. First Edit; Preedy, V.R.; Ed.; Elsevier Inc: London, 2016; 531–538
- Achouri, A.; Zamani, Y.; Boye, J.I. Stability and Physical Properties of Emulsions Prepared with and without Soy Proteins. Journal of Food Research 2012, 1, 254–267.
- Dalgleish, D.G. Food Emulsions—Their Structures and Structure-Forming Properties. Food Hydrocolloids 2006, 20, 415–422.
- McClements, D.J. Food Emulsion: Principle, Practices, and Techniques. 2nd ed.; CRC Press, Boca Raton, FL, USA, 2005; p.628
- Dickinson, E.; Bergenstahl, B. Food Colloids - Proteins, Lipids and Polysaccharides. Molecular Nutritition and Food Research 2004, 41, 417.
- Charoen, R.; Jangchud, A.; Jangchud, K.; Harnsilawat, T.; Decker, E.A.; McClements, D.J. Influence of Interfacial Composition on Oxidative Stability of Oil-In-Water Emulsions Stabilized by Biopolymer Emulsifiers. Food Chemistry 2012, 131, 1340–1346.
- Charoen, R.; Jangchud, A.; Jangchud, K.; Harnsilawat, T.; Naivikul, O.; McClements, D.J. Influence of Biopolymer Emulsifier Type on Formation and Stability of Rice Bran Oil-In-Water Emulsions: Whey Protein, Gum Arabic, and Modified Starch. Journal of Food Science 2011, 76(1), 165–172.
- Bouyer, E.; Mekhloufi, G.; Rosilio, V.; Grossiord, J.; Agnely, F. Proteins, Polysaccharides, and Their Complexes Used as Stabilizers for Emulsions: Alternatives to Synthetic Surfactants in the Pharmaceutical Field? International Journal of Pharmaceutics 2012, 436(1–2), 359–378.
- Silva, E.K.; Gomes, M.T.M.S.; Hubinger, M.D.; Cunha, R.L.; Meireles, M.A.A. Ultrasound-Assisted Formation of Annatto Seed Oil Emulsions Stabilized by Biopolymers. Food Hydrocolloids 2015, 47, 1–13.
- Amine, C.; Dreher, J.; Helgason, T.; Tadros, T. Investigation of Emulsifying Properties and Emulsion Stability of Plant and Milk Proteins Using Interfacial Tension and Interfacial Elasticity. Food Hydrocolloids 2014, 39, 180–186.
- Matsumura, Y.; Satake, C.; Egami, M.; Mori, T. Interaction of Gum Arabic, Maltodextrin and Pullulan with Lipids in Emulsions. Bioscience, Biotechnology, and Biochemistry 2000, 64, 1827–1835.
- Gharibzahedi, S.M.T.; Razavi, S.H.; Mousavi, S.M. Ultrasound-Assisted Formation of the Canthaxanthin Emulsions Stabilized by Arabic and Xanthan Gums. Carbohydrate Polymers 2013, 96(1), 21–30.
- Ozturk, B.; McClements, D.J. Progress in Natural Emulsifiers for Utilization in Food Emulsions. Current Opinion in Food Science 2015, 7, 1–6.
- Jafari, S.M.; Beheshti, P.; Assadpoor, E. Rheological Behavior and Stability of D-Limonene Emulsions Made by a Novel Hydrocolloid (Angum Gum) Compared with Arabic Gum. Journal of Food Engineering 2012, 109(1), 1–8.
- Rosenberg, M.; Sheu, T.-Y. Microencapsulation of Volatiles by Spray-Drying in Whey Protein-Based Wall Systems. International Dairy Journal 1996, 6(3), 273–284.
- Frascareli, E.C.; Silva, V.M.; Tonon, R.V.; Hubinger, M.D. Effect of Process Conditions on the Microencapsulation of Coffee Oil by Spray Drying. Food and Bioproducts Processing 2012, 90(3), 413–424.
- Wang, B.; Wang, L.J.; Li, D.; Adhikari, B.; Shi, J. Effect of Gum Arabic on Stability of Oil-In-Water Emulsion Stabilized by Flaxseed and Soybean Protein. Carbohydrate Polymers 2011, 86(1), 343–351.
- Turchiuli, C.; Munguia, M.T.J.; Sanchez, M.H.; Ferre, H.C.; Dumoulin, E. Use of Different Supports for Oil Encapsulation in Powder by Spray Drying. Powder Technology 2014, 255, 103–108.
- Taneja, A.; Ye, A.; Jones, J.R.; Archer, R.; Singh, H. Behaviour of Oil Droplets during Spray Drying of Milk-Protein-Stabilised Oil-In-Water Emulsions. International Dairy Journal 2013, 28(1), 15–23.
- Dluzewska, E.; Sobiecka, A.; Maszewska, M. Effect of Oil Phase Concentration on Rheological Properties and Stability of Beverage Emulsions. Acta Scientiarum Polonorum Technologia Alimentaria 2006, 5(2), 147–156.
- Jayme, M.; Dunstan, D.; Gee, M. Zeta Potentials of Gum Arabicstabilised Oil in Water Emulsions. Food Hydrocolloids 1999, 13(6), 459–465.
- Shao, P.; Shao, J.; Jiang, Y.; Sun, P. Influences of Ulva Fasciata Polysaccharide on the Rheology and Stabilization of Cinnamaldehyde Emulsions. Carbohydrate Polymers 2016, 135, 27–34.
- Thevenet, F.;. Acaciagum (Gumarabic). In Food Stabilisers, Thinckeners and Gelling Agents; Imeson, A.; Ed.; Blackwell Publishing, New Jersey, USA, 2006; 11–30.
- Patel, S.; Goyal, A. Applications of Natural Polymer Gum Arabic: A Review. International Journal of Food Properties 2015, 18(5), 986–998.
- McClements, D.J. Protein-Stabilized Emulsions. Current Opinion in Colloid and Interface Science 2004, 9(5), 305–313.
- Akhtar, M.; Dickinson, E. Whey Protein-Maltodextrin Conjugates as Emulsifying Agents: An Alternative to Gum Arabic. Food Hydrocolloids 2007, 21(4), 607–616.
- Jafari, S.M.; He, Y.; Bhandari, B. Nano-Emulsion Production by Sonication and Microfluidization - A Comparison. International Journal of Food Properties 2006, 9(3), 475–485.
- Perrier-Cornet, J.M.; Marie, P.; Gervais, P. Comparison of Emulsification Efficiency of Protein-Stabilized Oil-In-Water Emulsions Using Jet, High Pressure and Colloid Mill Homogenization. Journal of Food Engineering 2005, 66(2), 211–217.
- Le, H.D.; Le, V.V.M. Application of Ultrasound to Microencapsulation of Coconut Milk Fat by Spray Drying Method. Journal of Food Science and Technology 2015, 52(4), 2474–2478.
- Shanmugam, A.; Ashokkumar, M. Ultrasonic Preparation of Stable Flax Seed Oil Emulsions in Dairy Systems – Physicochemical Characterization. Food Hydrocolloids 2014, 39, 151–162.
- Silva, E.K.; Azevedo, V.M.; Cunha, R.L.; Hubinger, M.D.; Meireles, M.A.A. Ultrasound-Assisted Encapsulation of Annatto Seed Oil - Whey Protein Isolate versus Modified Starch. Food Hydrocolloids 2016, 56, 71–83.
- Ramisetty, K.A.; Pandit, A.B.; Gogate, P.R. Ultrasound Assisted Preparation of Emulsion of Coconut Oil in Water: Understanding the Effect of Operating Parameters and Comparison of Reactor Designs. Chemical Engineering and Processing: Process Intensification 2015, 88, 70–77.
- Cucheval, A.; Chow, R.C.Y. A Study on the Emulsification of Oil by Power Ultrasound. Ultrasonics Sonochemistry 2008, 15(5), 916–920.
- O’Brien, W.D.;. Ultrasound-Biophysics Mechanisms. Progress in Biophysics & Molecular Biology 2007, 93(1–3), 212–255.
- Jafari, S.M.; Assadpoor, E.; He, Y.; Bhandari, B. Encapsulation Efficiency of Food Flavours and Oils during Spray Drying. Drying Technology 2008, 26, 816–835.
- Silva, H.D.; Cerqueira, M.Â.; Vicente, A.A. Nanoemulsions for Food Applications: Development and Characterization. Food and Bioprocess Technology 2012, 5(3), 854–867.
- Tonon, R.V.; Pedro, R.B.; Grosso, C.R.F.; Hubinger, M.D. Microencapsulation of Flaxseed Oil by Spray Drying: Effect of Oil Load and Type of Wall Material. Drying Technology 2012, 30(13), 1491–1501.
- Botrel, D.A.; Borges, S.V.; Fernandes, R.V.D.B.; Carmo, E.L. Optimization of Fish Oil Spray Drying Using a Protein: Inulin System. Drying Technology 2014, 32(3), 279–290.
- Carneiro, H.C.F.; Tonon, R.V.; Grosso, C.R.F.; Hubinger, M.D. Encapsulation Efficiency and Oxidative Stability of Flaxseed Oil Microencapsulated by Spray Drying Using Different Combinations of Wall Materials. Journal of Food Engineering 2013, 115(4), 443–451.
- Beristain, C.I.; Garcı́a, H.S.; Vernon-Carter, E.J. Spray-Dried Encapsulation of Cardamom (Elettariacardamomum) Essential Oil with Mesquite (Prosopisjuliflora) Gum. LWT - Food Science and Technology 2001, 34(6), 398–401.
- Adjonu, R.; Doran, G.; Torley, P.; Agboola, S. Formation of Whey Protein Isolate Hydrolysate Stabilised Nanoemulsion. Food Hydrocolloids 2014, 41, 169–177.
- Mirhosseini, H.; Tan, C.P.; Hamid, N.S.A.; Yusof, S. Effect of Arabic Gum, Xanthan Gum and Orange Oil Contents on Ζ-Potential, Conductivity, Stability, Size Index and Ph of Orange Beverage Emulsion. Colloids and Surfaces A: Physicochemical and Engineering Aspects 2008, 315(1–3), 47–56.
- Mirhosseini, H.; Tan, C.P.; Hamid, N.S.A.; Yusof, S. Optimization of the Contents of Arabic Gum, Xanthan Gum and Orange Oil Affecting Turbidity, Average Particle Size, Polydispersity Index and Density in Orange Beverage Emulsion. Food Hydrocolloids 2008, 22(7), 1212–1223.
- Wang, B.; Li, D.; Wang, L.-J.; Özkan, N. Effect of Concentrated Flaxseed Protein on the Stability and Rheological Properties of Soybean Oil-In-Water Emulsions. Journal of Food Engineering 2010, 96(4), 555–561.
- Bae, E.K.; Lee, S.J. Microencapsulation of Avocado Oil by Spray Drying Using Whey Protein and Maltodextrin. Journal of Microencapsulation 2008, 25(8), 549–560.
- Rosa, M.T.M.G.; Silva, E.K.; Santos, D.T.; Petenate, A.J.; Meireles, M.A.A. Obtaining Annatto Seed Oil Miniemulsions by Ultrasonication Using Aqueous Extract from Brazilian Ginseng Roots as a Biosurfactant. Journal of Food Engineering 2016, 168, 68–78.
- Al-Malah, K.I.; Azzam, M.O.J.; Omari, R.M. Emulsifying Properties of BSA in Different Vegetable Oil Emulsions Using Conductivity Technique. Food Hydrocolloids 2000, 14(5), 485–490.
- Mirhosseini, H.; Tan, C.P.; Taherian, A.R. Effect of Glycerol and Vegetable Oil on Physicochemical Properties of Arabic Gum-Based Beverage Emulsion. European Food Research and Technology 2008, 228(1), 19–28.
- Masson, D.S.; Morais, G.G.; De Morais, J.M.; De Andrade, F.F.; Dos Santos, O.D.H.; De Oliveira, W.P.;, et al. Polyhydroxy Alcohols and Peach Oil Addition Influence on Liquid Crystal Formation and Rheological Behavior of O/W Emulsions. Journal of Dispersion Science and Technology 2005, 26(4), 463–468.
- Klein, M.; Aserin, A.; Ishai, P.B.; Garti, N. Interactions between Whey Protein Isolate and Gum Arabic. Colloids and Surfaces B: Biointerfaces 2010, 79(2), 377–383.
- Jambrak, A.R.; Mason, T.J.; Lelas, V.; Herceg, Z.; Herceg, I.L. Effect of Ultrasound Treatment on Solubility and Foaming Properties of Whey Protein Suspensions. Journal of Food Engineering 2008, 86, 281–287.
- Casteli, V.C.; Mendonça, C.C.; De Campos, M.A.L.; Ferrari, M.; Machado, S.R.P. Desenvolvimento E Estudos De Estabilidade Preliminares De Emulsões O/A Contendo Cetoconazol 2.0%. Acta Scientiarum. Health Sciences 2008, 30(2), 121–128.
- Sari, T.P.; Mann, B.; Kumar, R.; Singh, R.R.B.; Sharma, R.; Bhardwaj, M.;, et al. Preparation and Characterization of Nanoemulsion Encapsulating Curcumin. Food Hydrocolloids 2015, 43, 540–546.
- Mohammadzadeh, H.; Koocheki, A.; Kadkhodaee, R.; Razavi, S.M.A. Physical and Flow Properties of D-Limonene-In-Water Emulsions Stabilized with Whey Protein Concentrate and Wild Sage (Salvia macrosiphon) Seed Gum. Food Research International 2013, 53(1), 312–318.
- Herculano, E.D.; De Paula, H.C.B.; De Figueiredo, E.A.T.; Dias, F.G.B.; Pereira, V.D.A. Physicochemical and Antimicrobial Properties of Nanoencapsulated Eucalyptus staigeriana Essential Oil. LWT - Food Science Ande Technology 2015, 61(2), 484–491.
- Kuhn, K.R.; Cunha, R.L. Flaxseed Oil - Whey Protein Isolate Emulsions: Effect of High Pressure Homogenization. Journal of Food Engineering 2012, 111(2), 449–457.
- Jayasundera, M.; Adhikari, B.; Aldred, P.; Ghandi, A. Surface Modification of Spray Dried Food and Emulsion Powders with Surface-Active Proteins: A Review. Journal of Food Engineering 2009, 93(3), 266–277.
- Silva, V.M.; Vieira, G.S.; Hubinger, M.D. Influence of Different Combinations of Wall Materials and Homogenisation Pressure on the Microencapsulation of Green Coffee Oil by Spray Drying. Food Research International 2014, 61, 132–143.
- Xu, D.; Yuan, F.; Wang, X.; Li, X.; Hou, Z.; Gao, Y. The Effect of Whey Protein Isolate-Dextran Conjugates on the Freeze-Thaw Stability of Oil-In-Water Emulsion. Journal of Dispersion Science and Technology 2010, 32(1), 77–83.
- Mirhosseini, H.; Tan, C.P.; Hamid, N.S.A.; Yusof, S.; Chern, B.H. Characterization of the Influence of Main Emulsion Components on the Physicochemical Properties of Orange Beverage Emulsion Using Response Surface Methodology. Food Hydrocolloids 2009, 23(2), 271–280.
- Sukhotu, R.; Guo, S.; Xing, J.; Hu, Q.; Wang, R.; Shi, X.;, et al. Changes in Physiochemical Properties and Stability of Peanut Oil Body Emulsions by Applying Gum Arabic. LWT - Food Science and Technology 2016, 68, 432–438.
- Garti, N.;. Hydrocolloids as Emulsifying Agents for Oil-In-Water Emulsions. Journal of Dispersion Science and Technology 1999, 20(1–2), 327–355.
- Garcia, L.C.; Tonon, R.V.; Hubinger, M.D. Effect of Homogenization Pressure and Oil Load on the Emulsion Properties and the Oil Retention of Microencapsulated Basil Essential Oil (Ocimumbasilicum L.). Drying Technology 2012, 30(13), 1413–1421.
- Camino, N.A.; Pilosof, A.M.R. Hydroxypropylmethylcellulose at the Oil-Water Interface. Part II. Submicron-Emulsions as Affected by Ph. Food Hydrocolloids 2011, 25(5), 1051–1062.
- Kuhn, K.R.; Silva, F.G.D.; Netto, F.M.; Cunha, R.L. Assessing the Potential of Flaxseed Protein as an Emulsifier Combined with Whey Protein Isolate. Food Research International 2014, 58, 89–97.
- Conceição, M.C.; Junqueira, L.A.; Silva, K.C.G.; Prado, M.E.T.; Resende, J.V. Thermal and Microstructural Stability of a Powdered Gum Derived from Pereskiaaculeata Miller Leaves. Food Hydrocolloids 2014, 40, 104–114.
- İbanoğlu, E.;. Rheological Behaviour of Whey Protein Stabilized Emulsions in the Presence of Gum Arabic. Journal of Food Engineering 2002, 52(3), 273–277.
- Tadros, T.F.;. Rheology of Dispersions: Principles and Applications. First Edit; Rheology of Dispersions: Principles and Applications. Wiley-VCH, Weinheim, Germany, 2010; p218 .
- Moschakis, T.; Murray, B.S.; Biliaderis, C.G. Modifications in Stability and Structure of Whey Protein-Coated O/W Emulsions by Interacting Chitosan and Gum Arabic Mixed Dispersions. Food Hydrocolloids 2010, 24(1), 8–17.