ABSTRACT
To improve the efficiency of traditional boiling process, a new high-pressure decocting method was developed for the production of Radix Angelica sinensis (RAS) decoction with higher contents of efficacy components (Z-ligustilide and ferulic acid) and better flavour properties. Based on preliminary tests, a three-level Box–Behnken design was employed to optimise the process parameters, including decocting temperature (120–140°C), decocting time (15–45 min), and liquid/solid ratio (35:1–45:1 mL/g). The optimal conditions were as followed: a temperature of 125°C, a decocting time of 15 min, and a liquid/solid ratio of 37:1 mL/g. In addition, 38 major aroma-active compounds in optimum decoction were identified by gas chromatography–olfactrometry–mass spectrometry (GC-O-MS). With favour dilution (FD) factors ≥81, 6-undecanone, α-pinene, hexanal, octanal, furfural, benzaldehyde, 2-methoxy-4-vinylphenol, butylidenephthalide, and ligustilide were identified as important aroma components. Furthermore, pyridine, furfural, benzaldehyde, 2-methyl-benzothiazole, benzothiazole, and 2-methylthio-benzothiazole were also found in RAS decoction, which were not detected in RAS materials in previous studies.
Introduction
Radix Angelica sinensis (RAS), known as Danggui in China, is one of the most popular traditional Chinese medicines (TCMs). It has been used for thousands of years, which was firstly cited in Shennong Bencao Jing (200–300 A.D., Han Dynasty), a classical masterpiece of TCMs.[Citation1] RAS, also known as ‘female ginseng’, has been widely applied to treat gynecological conditions.[Citation2,Citation3] Besides that, constipation, hepatic fibrosis, anaemia, cardiovascular disease, and so forth can also be treated, for its pharmacological action on blood system, immune system, nervous system, respiratory system, cardio-cerebral vascular system, and other effects on anti-oxidative, anti-inflammatory, antineoplastic, and antiviral activities.[Citation4,Citation5] For blood system, RAS not only can promote hematopoiesis and anti-anaemia but also can restrain platelet aggregation and improve haemorheology.[Citation6–Citation8] For immune system, RAS has the pharmacological action of promoting T-lymphocyte proliferation, activating B-lymphocyte, increasing the phagocytosis of mononuclear macrophage and natural killer activity, and enhancing the activity of immune factors.[Citation9,Citation10] In addition, neuro protective effect and repair function for nervous system,[Citation11,Citation12] and inhibition of pulmonary fibrosis for respiratory system were also verified.[Citation13,Citation14] For cardio-cerebral vascular system, RAS has effects on protection of ischemic injury,[Citation15] anti-arrhythmia,[Citation16] anti-atherosclerosis,[Citation17] lowering blood lipid and blood pressure.[Citation17,Citation18]
Modern researches indicate that essential oil (main including monomeric phthalides, phthalide dimers, terpenes, etc.), organic acids (ferulic acid, protocatechuic acid, phthalic acid, p-hydroxybenzoic acid, vanillic acid, caffeic acid, nicotinic acid, folinic acid, etc.) and their esters, and (neutral and acidic) polysaccharides are main chemical components related to the bioactivities and pharmacological properties of RAS.[Citation2,Citation19,Citation20] In essential oil, one of the major monomeric phthalides is Z-ligustilide, with the efficiency of neuro protection, vasodilation, anticancer and antitumour activities, anti-inflammation, and analgesic.[Citation2,Citation21] In addition, ferulic acid, as a kind of organic acids in RAS, has extensive bioactivities related to medical function of RAS, physical conditioning by scavenging free radical, increasing the activity of glutathione S-transferase and quinone reductase, and inhibiting tyrosinase activity.[Citation2,Citation22] For RAS polysaccharides, many studies on phytochemistry and pharmacology have demonstrated its various bioactivities, which are strongly related to chemical structures and chain conformations, such as haematopoiesis, immunomodulation, anti-tumour, antioxidant, anti-ulcer, and radioprotection.[Citation23] Until now, more than 70 compounds have been isolated and identified from RAS, including other components, such as flavonoids, amino acids, biological bases, vitamins, nucleosides, and choline.[Citation2,Citation19,Citation20] Although there are mass constituents in RAS extract, the pharmacological action of RAS largely depends upon essential oil, organic acids, and polysaccharides, which can be applied to its quality evaluation. However, compared with Z-ligustilide and ferulic acid, RAS polysaccharides are rather difficult to be purified and used for quantitative analysis. According to ‘China Drug Code (2015)’, the quality evaluation indices of Radix Angelica sinensis (RAS) are the contents of ferulic acid and total volatile oil. Moreover, the pharmacological and chemical studies showed that Z-ligustilide was the predominate components of volatile oil, having stronger pharmacological actions on haematopoiesis system, circulation system, nervous system, and so forth. Therefore, based on ‘China Drug Code (2015)’ and some other relative researches, only Z-ligustilide and ferulic acid are selected as marker compounds to evaluate the quality of RAS frequently.[Citation1,Citation2,Citation24–Citation26]
For decocting RAS by traditional method, some active components can decompose during the long processing time involving high heat.[Citation27–Citation30] It is necessary to develop new decocting methods to improve the efficiency of the process. In addition, to the best of our knowledge, few papers have focused on evaluating the relative importance of flavour compounds in RAS decoction. Therefore, the objective of this study was to optimise high-pressure decocting process for both major efficacy components and flavour properties of RAS decoction by Box–Behnken experimental design. HPLC-DAD was used to determine the mark ingredient contents in the decoction boiled under different conditions. Meanwhile, flavour properties were evaluated by sensory evaluation according to acceptability (overall liking scores) given by panelists. Finally, the aroma-active compounds of RAS decoction were investigated by the method of SPME combined with GC-O-MS, and the aroma dilution method by changing GC split ratios was used to screen the key odour-active compounds with highest flavour dilution (FD) factors.
Materials and methods
Sample and chemicals
Sample preparation
Samples of whole roots of RAS were collected from Gansu province of China. The samples were powdered to a homogeneous size in a mill and sieved through a 40-mesh sieve. The powdered sample was immediately sealed into a 50 mL plastic centrifuge tube and stored at −20°C for further analysis. Then 1.00 g of above sample was weighed, and the decocting process was performed in a 50 mL high-pressure autoclave (Parr-4848; USA) under different conditions with respect to temperature, time, and liquid/solid ratio. Because of its closed system, high temperature parameters accorded with the actual pressure parameters in the autoclave. After high-pressure boiling, the decoction was cooled and centrifuged at 5000 rpm for 10 min and then filtered to remove insoluble materials. The obtained liquid was used for further analysis.
Chemicals
Z-Ligustilide and ferulic acid were purchased from Reference Material Information Center of China (Beijing, China). Acetonitrile (chromatographic reagent) and phosphoric acid (chromatographic reagent) were obtained from Huihai Scientific Instruments Co., Ltd (Beijing, China), and n-alkanes (C7-C30) and 2-methyl-3-heptanonewere were obtained from Sigma-Aldrich (St Louis, MO, USA).
High performance liquid chromatography-diode-array detector
High performance liquid chromatography (HPLC) analysis was conducted on an Agilent Series 1200 LC instrument (Agilent Technologies, USA) equipped with an online degasser, a quaternary pump, a diode-array detector (DAD), a thermostated column compartment, and an auto-sampler. The analytes (ferulic acid and Z-ligustilide) separation was performed on a ZORBAX SB-C18 column (5 μm, 4.6 × 250 mm, Agilent, USA). The column temperature was maintained at 25°C. The mobile phase consisted of solvent A (acetonitrile) and B (0.1% aqueous phosphoric acid, v/v). Gradient elution was as follows: 85–80% B (v/v) at 0–15 min, 80–50% B (v/v) at 15–30 min, 50–20% B (v/v) at 30–60 min. The injection volume of each sample and standard solution was 5 μL, and the flow rate was continually maintained at 1.0 mL/min.
Quantification of ferulic acid and Z-ligustilide
Z-ligustilide and ferulic acid are selected as marker compounds to evaluate the quality of the decoction. Methanol stock solution containing standard substance was prepared and diluted to appropriate concentration ranges for the establishment of calibration curves. Six different concentrations of the two analytes were injected in triplicate, and then the calibration curves were constructed by plotting the peak areas versus the concentration of each analyte. Calibration data was listed in .
Table 1. Calibration date used for quantification of bioactive compounds of RAS decoction by High Performance Liquid Chromatography-Diode-Array Detector.
Sensory evaluation
Ten trained panelists (3 males and 7 females, aged 22–26 years) were recruited from Laboratory of Molecular Sensory Science, Beijing Technology and Business University. Experimental samples were prepared the day before the test, held in a freezer, and then warmed to room temperature just before serving to panelists in 50 mL plastic centrifuge tube coded with 3-digit random numbers. Each panelist rated the acceptability (overall liking) of the aroma (by sniffing) of each sample using a 9-point hedonic scale (1 = dislike extremely, 5 = neither like nor dislike, and 9 = like extremely).[Citation31] Each panelist was asked to take a rest for sensory evaluation between each set of the different samples. All the evaluations were performed under a dim light to mask the colour differences between samples. All samples were evaluated in one-time evaluation, and three independent sessions were included. Differences of the same result between sessions were not more than two category scales.
Response surface methodology experimental design
A Box–Behnken design[Citation32] was used for the design of experiment with three independent variables, including decocting temperature (X1), decocting time (X2), and liquid/solid ratio (X3) using a commercial statistical package, Design Expert version 9 (Stat Ease Inc., Minneapolis, MN, USA). The levels of each variable were established based in a series of preliminary experiments and coded as −1, 0, and 1 () resulting in a total of 15 experimental runs to investigate the effect of these process variables on the responses (the total contents of ferulic acid and Z-ligustilide, and overall liking value, respectively). Quadratic polynomial models were fitted to the data as necessary to obtain the regression equations. The statistical significance of the terms in the regression equations was examined by analysis of variance (ANOVA) for each response. The decocting parameters were optimised by using the numerical method of RSM based on Derringer’s desirability function[Citation33] to obtain decoction of functionality and acceptable odour property. The independent variables were kept within the experimental range while the responses were maximised.
Table 2. Factors and levels for the Box-Bhehnken experimental design.
Isolation of aroma-active components
Solid phase microextraction
Volatile extraction in the RAS decoction was carried out by headspace solid phase microextraction (HS-SPME). Decoction samples (10 mL) were placed in 40 mL flat bottom headspace vials fitted with a PTFE/silicone septum and crimp cap. The SPME fibre was preconditioned prior to analysis at 250°C for 30 min. The headspace vial was heated using water-bath heater at 55°C for 20 min. After equilibration, the volatile components in the samples were adsorbed onto a 50/30 μm layer of divinylbenzene–carboxen–polydimethylsiloxane fibre (Supelco Co., Bellefonte, USA) that was exposed to the sample headspace for 40 min. Finally, the SPME fibre was introduced to injection port to desorb aroma compounds for 5 min when GC-O-MS started to run pre-set programme.
Aroma dilution method
It is necessary to use dilution methods to find key aroma compounds in RAS decoction, and aroma dilution by changing GC split ratios is a reliable method. It was demonstrated that serial dilution of the flavour compounds in SPME-GC-O can be achieved through the successive increase of GC split ratio.[Citation34,Citation35] The volatiles extracted from RAS decoction were diluted by stepwise by varying the injector split ratio as follows: 1(splitless), 3, 9, 27, 81, and 243. Flavour dilution (FD) factor was defined as the maximum split ratio at which a compound could be perceived at the sniffing port, and FD factor was based on the evaluation of three experienced panelists.
Gas chromatography–olfactometry–mass spectrometry
All the volatiles were analysed by GC-MS (7890A-7000B; Agilent Technologies Inc., Santa Clara, CA, USA), which was equipped with an olfactory detector port (Sniffer 9000; Brechbühler, Schlieren, Switzerland). After injection, separations by GC were performed on a polar DB-Wax column (30 m × 0.32 mm × 0.25 m; J & W Scientific, Folsom, CA, USA) or a non-polar DB-5 column (30 m × 0.32 mm × 0.25 m; J & W Scientific), ultra-high purity helium was used as the carrier gas at 1.2 mL /min. The GC oven programme was held for 3 min at 40°C and ramped at 5°C/min to 200°C, and then ramped at 10°C/min to 230°C and held at this temperature for 5 min. The effluent from the capillary column was split 1:1, v/v, between the mass spectrometry detector (MSD) and the olfactory detector port (Sniffer 9000; Brechbühler). The GC-MS transfer line temperature was 240°C. Electron-impact liquor spectra were generated at 70 eV, with a m/z scan range from 40 to 300. The MS source temperature was 230°C. Three experienced panelists were recruited to perform sniffing test on GC-O-MS. To prevent drying of the nasal mucosa, humidified air was supplied to the sniff port at 30 mL/min.
Identification of the volatile compounds
The preliminary identification of volatile compounds was based on the NIST 08 mass spectra libraries installed in the GC-MS. Reconfirmation was performed by comparison of the mass spectrum (MS), retention index (RI), and odour descriptions with authentic standards as described previously.[Citation36]
Quantification of the volatile compounds
Relative contents of predominant odourants were studied by internal standard method. As the internal standard substance, 1 μL 2-methyl-3-heptanone (0.408 μg/μL in methanol) was added to 10 mL RAS decoction in the headspace vial, prepared under optimum condition. Therefore, the internal standard concentration in the decoction was 40.8 ng/g by calculation. The concentration of each compound was calculated as follows:
where was the concentration of an odourant;
was the concentration of internal standard, 40.8 ng/g;
was the area of the odourant on total ion chromatogram;
was the area of internal standard.
Statistical analysis
The calculations and figures of response surface models were done using the Design Expert version 9 (Stat Ease Inc., Minneapolis, MN, USA). The experiments of Box–Behnken design were evaluated using analysis of variance (ANOVA) to find the significance of each factors and interaction terms.
Results and discussion
Preliminary tests
The decocting temperature (100–150°C), decocting time (10–40 min), and liquid/solid ratio (10:1–50:1 mL/g) were studied using a single factor test to determine the most influential ranges for in-depth investigations. The total contents of Z-ligustilide and ferulic acid and the acceptability (overall liking value) were investigated for each factor while other factors were held at a constant value.
The appropriate temperature was the first factor investigated. The total yield for efficacy compounds was compared under different temperatures (100°C, 110°C, 120°C, 130°C, 140°C, and 150°C). A final temperature of 130°C was determined to be the most effective for extraction efficiency. It was likely due to the fact that some of the active compounds may decompose involving higher heat condition.[Citation28–Citation30] In addition, the overall liking according to the panelists’ evaluation indicated that the decoction with a strong smell of sweet or herbal aroma at the temperature of 130°C had a higher score than that of earthy or caramel aroma in the condition of lower or higher than 130°C, respectively (date not shown). Therefore, 130°C was reasonable for further study.
The decocting time was the second factor investigated. The total contents for the major bioactive compounds were compared in the boiling conditions 130°C for 0, 15, 30, 45, 60, and 90 min. The results indicated that the maximum value for total contents and acceptability was reached at 30 min. Hence, 30 min was determined to be the most appropriate time for further studies.
The most appropriate liquid/solid ratio was the third factor to be investigated. Liquid/solid ratios of 20:1, 25:1, 30:1, 35:1, 40:1, and 45:1 (mL/g) were utilised for boiling while maintaining a temperature of 130°C and decocting time of 30 min. The total contents of the marker compounds reached the peak when the liquid/solid ratio was 40:1(mL/g). However, there was less obvious change in the decoction for its acceptability from 35:1 to 45:1. Hence, 40:1(mL/g) was the most appropriate ratio for the decocting condition. Based on these preliminary tests, we adopted a decocting temperature of 120–140°C, a decocting time of 15–45 min, and a liquid/solid ratio of 35:1–45:1 mL/g for all subsequent RSM experiments.
Response surface methodology
Fitting the response surface models
The total contents of marker ingredients and overall liking from all 15 experiments are shown in . The Design Expert software generated two regression equations demonstrating the empirical relationship between the response values and parameters of decocting temperature (X1), decocting time (X2), and liquid/solid ratio (X3). The quadratic polynomial model equations for total contents of ferulic acid and Z-ligustilide (Y1) and overall liking (Y2) were as follows:
Table 3. The Box-Behnken experimental design with responses.
A model is well fitted to the experimental data if the regression analysis is significant but the lack-of-fit analysis is non-significant. For the equation Y1, the model F-value of 21.79 implied the model was significant and therefore suitable for this experiment (p-value was 0.0017). These results were confirmed by the non-significant results of the lack-of-fit error analysis (lack of fit F-value = 15.77, p = 0.062 > 0.05). The coefficient of determination (R2) and adjusted coefficient of determination (Adj. R2) were 0.9751 and 0.9304, respectively, indicating adequate accuracy and general availability of the polynomial model. Based on the factors above, it was deduced that the model was appropriate to predict the responses. For any term in the model, a small p-value indicates a more significant effect of that term on the respective response variables. p-values less than 0.05 indicated model terms were significant, such as X3, X1X2, X2X3, X12, and X32. Thus, the variable with the largest effect on the extraction rate was the quadratic term of liquid/solid ratio(X32) (p = 0.0002). This was followed by the quadratic term of the decocting temperature (X12) (p = 0.001) and the interaction term of decocting time and liquid/solid ratio (X2X3) (p = 0.0043). Finally, the interaction term of decocting temperature and decocting time (X1X2) (p = 0.0125) and the linear term of liquid/solid ratio(X3) (p = 0.0268) had less significant effect on the total contents of ferulic acid and Z-ligustilide. For the equation Y2, ANOVA results of overall liking also implied the model was suitable to predict the response. The model F value = 4.28(p = 0.037 < 0.05) of the equation Y2 illustrated that it was significant and was appropriate for response surface evaluation, which was also confirmed by the non-significant results of the lack-of-fit error analysis (lack of fit F-value = 13.99, p = 0.068 > 0.05). In addition, variable significant test indicated that decocting temperature(X1) (p = 0.0079) and liquid/solid ratio(X3) (p = 0.0277) were relative important factors to affect overall liking degree (Y2). In conclusion, both of the quotations, Y1 and Y2, were well fitted to the experimental data and were able to predict and evaluate the response results, effectively.
Analysis of response surface
Surface response plots of the model allow us to visualise the effect of the independent variables on the dependent variables and to have a better understanding of the interactive effects among the variables. Response surfaces were plotted for two independent variables with the other one independent variable fixed at constant level.
Direct correlation between temperature and time with total contents of marker ingredients (Y1) were observed (). Increasing time slightly decreased Y1. Maximum Y1 values were characterised by low levels of time and middle levels of temperature. The region of optimum response was localised at temperature 122–132°C and at a decocting time below 30 min. The response surface obtained when plotting Y1 against temperature and liquid/solid ratio () showed that Y1 initially increased with increasing liquid/solid ratio; however, at higher ratio, Y1 was slightly decreased. Similar results were observed for temperature. Optimum area of ratio and temperature for bio-active content was selected from the response surface plot as liquid/solid ratio 38:1–43:1 mL/g and temperature 123–135°C. Figure 1C demonstrated that as the liquid/solid ratio increased to middle range, the extraction of Y1 increased accordingly and declined after further increasing the ratio to a higher range, regardless of decocting time. Liquid/solid ratio had a greater influence on the bioactive contents (Y1) than did decocting time. The highest response was located in the area of time 15–26 min and liquid/solid ratio 38:1–41:1.
Figure 1. Response surface plots reflecting the effects of process parameters on mark ingredients: A: temperature versus time, X3 = 40:1 mL/g; B: temperature versus liquid/solid ratio, X2 = 30 min; C: time versus liquid/solid ratio, X1 = 130°C.
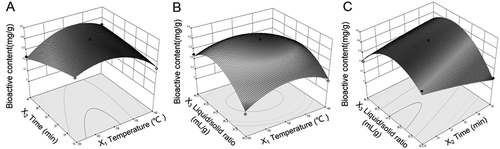
From , the best range for each of the three factors was basically same under different interactive effects among the variables. Ligustilide is an unstable compound, which can change to other phthalides through oxidation and dimerisation.[Citation28] In addition, as a major phenolic compound in RAS, ferulic acid was readily oxidised under heat and could be degraded in extreme condition.[Citation28–Citation30] Hence, the total content of ligutilide and ferulic acid would decrease under the condition of higher temperature or longer time. According to the analysis of response surface above, the suitable ranges for temperature and time were about 122–135°C and 15–30 min, respectively. For liquid/solid ratio, the total contents of ligustilide and ferulic acid in the decoction increased to a remained level with increasing the ratio, because more major efficacy compounds were dissolved in the decoction until balance. However, it was observed that the efficacy content slightly declined with the ratio higher than 41:1. It might be caused by the interactive effects among factors.
A three-dimensional response surface plot of overall liking (Y2) as a function of temperature and time at X3 = 37:1(mL /g) is shown in . On the whole, an increase in temperature from 120°C to 140°C resulted in a decrease in overall liking, especially at a longer time point, indicating significant influence of temperature. When the temperature was higher than 130°C, the acceptability (Y2) slightly decreased with increasing decocting time, while Y2 increased with the decocting time delayed when the temperature was lower than 130°C. Therefore, the best flavour properties of the decoction were in the condition of temperature 120–125°C and time longer than 30 min. Correlation between temperature and liquid/solid ratio with overall liking (Y2) was observed (, X2 = 40 min). Similar results were that increasing temperature decreased acceptability, regardless of liquid/solid ratio. The ratio had little influence on Y2 with the temperature higher than 130°C, and lower liquid/solid ratio could increase the overall liking. Figure 2C shows that overall liking slightly increased as liquid/solid ratio decreased. In general, Y2 initially increased with increasing decocting time; however, it was opposite at the ratio of 35:1–37:1 due to the interaction among the variables.
Figure 2. Response surface plots reflecting the effects of process parameters on acceptability (overall liking): A: temperature versus time, X3 = 37:1 mL/g; B: temperature versus liquid/solid ratio, X2 = 40 min; C: time versus liquid/solid ratio, X1 = 125°C.
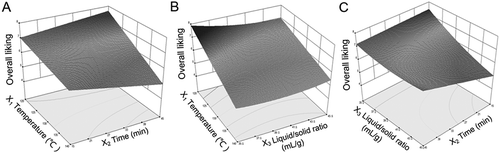
Panelists’ evaluation indicated that the decoction boiled with a temperature higher than 130°C might produce off-flavour, like caramel and burnt smell. In addition, long-time decocting at a lower temperature had a good flavour property with stronger sweet and herbal smell, which was easy to accept. Moreover, panelists preferred to lower liquid/solid ratio because it had stronger smell than did higher ratio.
The reason for aroma change during the heating process maybe is that the contents of odourant compounds change in different decocting conditions. The contents of volatile compounds increase with transferring to water. However, some of them are thermal labile to decompose or evaporate at high temperature, which might decrease the attractive special flavour.[Citation27] In addition, some new aroma-active compounds may be formed by some reactions, like Maillard reaction.[Citation37,Citation38]
Multiple responses optimisation
It is relatively simple to find the optimal conditions for a single response using surface response design; however, this study required optimisation of two responses. Consequently, a Derringer’s desirability function was utilised in order to optimise several responses simultaneously.[Citation33] Therefore, the decocting conditions were optimised with the Derringer’s desirability function using the numerical optimisation function of the Design Expert programme. The numerical ranges specified were temperature between 120°C and 140°C, time within 15–45 min, and liquid/solid ratio between 35:1 and 45:1. Simultaneously, the programme was designed to maximise Y1 and Y2. The optimum conditions given by the model were as follows: temperature of 125.38, time of 15 min, and liquid/solid ratio of 36.69:1 mL/g ().
Table 4. Predicted and experimental values of the responses obtained under the optimal extraction conditions.
Verification of predicted model
To examine the suitability of the quadratic equations for predicting the optimal response values, the verification experiments was carried out under adjusted conditions (125°C, 15 min, and 37:1 mL/g) on the basis of the optimal condition. The experiment results are listed in . In addition, variations between the predicted and experimental values obtained for Y1 and Y2 were within an acceptable error range as depicted by relative error (RE %). This confirmed that the model accurately predicted the decocting process for the optimal condition.
Aroma-active compounds in optimum decoction
To further investigate the flavour of RAS decoction that was boiled in the optimised condition, it is necessary to identify the predominant aroma-active compounds besides sensory evaluation. SPME-GC-O-MS was carried out to analyse the flavour quality of RAS decoction and the application of GC split ratio was used as dilution analysis.
In the decoction, 38 predominant odour-active compounds were detected by using both polar and non-polar columns for chromatography (). The FD factors of the compounds were detected to fall within the range of 3–243. Among them, 6-undecanone (herbal) reached the highest FD factor (243) and was considered as the odourants that had the most impact on the decoction. Moreover, α-pinene (wood-like), hexanal (cut-grass), octanal (green), furfural (bread-like), benzaldehyde (almond-like), 2-methoxy-4-vinylphenol (curry-like), butylidenephthalide (herbal), and ligustilide (herbal) with FD factor of 81 were also identified as important aroma components of the optimised decoction. Most of them have been reported in previous studies of volatile components in RAS.[Citation39–Citation45] Particularly, all of terpenes detected in the decoction were found in the RAS essential oil. Among them, the amounts of ligustilide and butylidenephthalide were the major volatile components of RAS extracts.[Citation43]
Table 5. Predominant odourants identified in optimum decoction by dilution analysis of GC split ratio.
Although more than 50 volatile compounds were detected in RAS,[Citation44,Citation45] not all of them possessed odour activity. It was necessary to investigate the aroma-active compounds in the decoction and their contribution to overall flavour properties by means of GC-O-MS and aroma dilution methods. As shown in , a great variety of odour qualities were perceived, such as herbal, pine, hay-like, sweet, green, earthy, curry-like, which were positively correlated with the overall flavour properties of optimum decoction as the panelists’ description.
The volatile components were not an exact match between RAS and its decoction. Pyridine (rancid), furfural (sweet, bread-like), benzaldehyde (almond-like), 2-methyl-benzothiazole (burnt, pungent), and benzothiazole (rubber-like, caramel) were identified for the first time in RAS decoction, which were not detected in RAS. Maillard reaction widely occurred in food processing and preparation of the alternative herbal medicines, the boiling process of soup for instance, and so forth.[Citation37] In preliminary tests, it was observed that the decoction colour turned from light brown to dark with the increase of boiling time and temperature. As we know, a non-enzymatic browning reaction reacted in some foods that were rich in proteins, amino acids, and saccharides. The RAS decoction was a complex system that contained many compounds that came from RAS, including those from essential oil, phthalide dimers, coumarins, organic acids and their esters, polysaccharides, polyacetylenes, vitamins, amino acids, and others.[Citation2] In the heating process, some of them might participate in Mallard reaction in RAS decoction. Hence, the sulphur-containing compounds or nitrogen-containing compounds, like pyridine, 2-methyl-benzothiazole, benzothiazole, and 2-methylthio-benzothiazole, might be formed by Maillard reaction. These compounds reflected off-flavour in the decoction and should be controlled by boiling conditions. Furans were formed by sugar caramelisation and carbohydrate degradation.[Citation46] Furfural, as a kind of furan compound, might come from the degradation and caramelisation of polysaccharides in RAS. It had a sweet or bread-like property, which can improve the acceptability of the decoction.
Conclusions
In this research, a new rapid and efficient decocting method was developed to boil RAS for the decoction with both high contents of efficacy components and better flavour properties. The decocting conditions were obtained by the RSM coupled with Box–Behnken design procedure. In addition, Derringer’s desirability function was also applied to optimise the responses of the marker contents (Z-ligustilide and ferulic acid) and acceptability (overall liking) simultaneously. The optimum condition was determined to be a temperature of 125°C, a decocting time of 15 min, and a liquid/solid ratio is 37:1 mL/g. Under this condition, 38 aroma-active compounds were identified in the decoction by SPME-GC-O-MS with two different polar columns. The key odourants were identified by changing split ratios, and some sulphur-containing compounds or nitrogen-containing compounds might be formed by Mallard reaction in heating process, which was not found in RAS in previous studies. This research may help to develop machines for decocting TCMs. In further studies, the changing contents of key aroma-active compounds in the decocting process will be investigated to reveal the correlation between sensory evaluation and flavour compounds.
LJFP_A_1306552_Supplemental_Figure.tif
Download TIFF Image (4.1 MB)Supplemental data
Supplemental data for this article can be accessed on the publisher’s website.
References
- The State Pharmacopoeia Commission of the People’s Republic of China, Pharmacopoeia of the People’s Republic of China. China Medical Science and Technology Press 2015, 1, 133–134.
- Yi, L.; Liang, Y.; Wu, H.; Yuan, D. The Analysis of Radix Angelicae sinensis (Danggui). Journal of Chromatography A 2008, 1216, 1991–2001.
- Wang, Z.; Xiao, Y.; Ou, L. Research Progress of Angelica. Journal of Medicine and Pharmacy of Chinese Minorities 2013, 9, 64–66. (in Chinese)
- Deng, P.; Deng, Y. Study Progress on Pharmacodynamics of Dang Gui and Its Active Ingredients. Western Journal of Traditional Chinese Medicine 2012, 9, 125–128. (in Chinese)
- Liu, Y.; Yang, S.; Ma, W.; Song, Y. Study Progress on Pharmacological Action of Angelica sinensis. China Modern Medicine 2014, 22, 192–196. (in Chinese)
- Li, J.; Liu, Y. Research Progress on Hematopoiesis of Angelica sinensis. Medical Recapitulate 2010, 7, 1089–1091. (in Chinese)
- Liu, X.; Li, W.; Cai, B. Chemical Composition of Angelica sinensis and Its Study Progress on Cardiovascular System. Journal of Nanjing University of Traditional Chinese Medicine 2010, 2, 155–157. (in Chinese)
- Wu, D.; Song, C.; Han, Z.; Fan, Y.; Kong, J.; Hu, Z. Effects of Angelicae sinensis Decoction for Supplementing Blood on the Cardiac Function in Myocardial Ischemia Reperfusion Injury of Rats. Pharmacology and Clinics of Chinese Materia Medica 1999, 15, 1–3. (in Chinese)
- Yang, T.; Lu, B.; Jia, M.; Mei, Q. The Effect of Angelica sinensis Polysaccharide on Immunologic Function in Mice. Chinese Pharmacological Bulletin 2003, 4, 448–451. (in Chinese)
- Feng, J.; Zhao, G. Study Progress on Immunopharmacology of Angelica sinensis. Fujian Journal of Traditional Chinese Medicine 2003, 3, 49–51. (in Chinese)
- He, L. Research Progress and Prospect of Angelica sinensis on the Effect of Nervous and Immune System. Lishizhen Medicine and Materia Medica Research 2007, 9, 2282–2284. (in Chinese)
- Lin, G. Protective Effect of Angelica sinensis Polysaccharide on Hippocampal Neuron in Cerebral Ischemia Reperfusion Injury of Rats. Chinese Traditional and Herbal Drugs 2015, 4, 558–561. (in Chinese)
- Wang, Y.; Wang, X.; Zhang, X. Effect of Angelica sinensis Polysaccharide on Lung Function and Coefficient in Pulmonary Fibrosis of Rats. Gansu Journal of Traditional Chinese Medicine 2010, 11, 28–31. (in Chinese)
- Liu, X. Clinical Research of Angelica sinensis Injection on the Effect of Pulmonary Fibrosis Tissue of Patients. Chinese Journal of Basic Medicine in Traditional Chinese Medicine 2014, 3, 351–352. (in Chinese)
- Chen, Y.; Ming, H.; Wang, Q.; Zhao, P.; Guo, C.; Zhao, Y. Effect of Angelica sinensis from Min Country on Ischemia-Reperfusion Arrhythmia of Rats. Western Journal of Traditional Chinese Medicine 2013, 2, 15–17. (in Chinese)
- Wang, Z.; Guo, M.; Ren, Y. Research Progress of Angelica sinensis and Its Pharmaceutics on Anti-Arrhythmia. Journal of Gansu College of Traditional Chinese Medicine 2008, 6, 49–51. (in Chinese)
- Lin, Z.; Zhou, L.; Yi, Q. Effects of Angelica sinensis Decoction for Supplementing Blood on Blood Lipid of Atherosclerosis Rats. Clinical Journal of Chinese Medicine 2013, 12, 19–20. (in Chinese)
- Ji, L.; Shi, X.; Chen, P.; Wang, L.; Yi, L. Study Progress of Angelica sinensis Extract on the Mechanism of Lowering Blood Pressure. Lishizhen Medicine and Materia Medica Research 2016, 8, 1965–1967. (in Chinese)
- Wang, Y.; Zhang, S.; Zhu, T.; Jin, L.; Li, Y. Researches on the Extraction and Analysis of Effective Components of Angelica sinensis. Journal of Traditional Chinese Veterinary Medicine 2016, 2, 75–79. (in Chinese)
- Dong, Q.; Chen, C. Researches on Chemical Composition and Pharmacological Action of Angelica sinensis. Asia-Pacific Traditional Medicine 2016, 2, 32–34. (in Chinese)
- Zhang, L.; Lv, J.; Chen, L.; Duan, J.; Liu, J. Research Progress on Phthalides and Their Pharmacological Action in Angelica sinensis. China Journal of Chinese Materia Medica 2016, 2, 167–176. (in Chinese)
- Liang, N.; Sun, S.; Luo, Y.; Di, L.; Liu, B. Researches on Ferulic Acid. Heilongjiang Journal of Traditional Chinese Medicine 2009, 3, 39–40. (in Chinese)
- Jin, M.; Zhao, K.; Huang, Q.; Xu, C.; Shang, P. Isolation, Structure and Bioactivities of the Polysaccharides from Angelica sinensis (Oliv.) Diels: A Review. Carbohydrate Polymers 2012, 89, 713–722.
- Wang, J.; Zhao, J.; Song, P. The Determination of Z-Ligustilide and Ferulic Acid in 30 Batches of Samples. Chinese Journal of Experimental Traditional Medical Formulae 2011, 16, 70–73. (in Chinese)
- Jiang, W.; Wang, C.; Wang, Z. Study on Preparation of Extract from Radix Angelicae and Its Quality Control. West China Journal of Pharmaceutical Sciences 2010, 25, 87–89. (in Chinese)
- Duan, R.; Xue, R.; Li, J.; Yang, S.; Dong, T.; Zhan, H. Quality Evaluation of Radix Angelica sinensis from Different Habitats by HPLC-DAD. Modern Chinese Medicine 2009, 11, 27–29. (in Chinese)
- Wu, T.; Shiau, S.; Chang, R. Effects of Drying Temperature and Extraction Solvent on the Antioxidant, Color and Sensory Properties of Angelica sinensis Leaves. Journal of Food Processing & Preservation 2013, 37, 371–379.
- Huang, S.; Chen, C.; Lin, C.; Chiang, B. Antioxidant and Flavor Properties of Angelica sinensis Extracts as Affected by Processing. Journal of Food Composition & Analysis 2008, 21, 402–409.
- Lu, G.; Chan, K.; Chan, C.; Leung, K.; Jiang, Z.; Zhao, Z. Quantification of Ligustilides in the Roots of Angelica sinensis and Related Umbelliferous Medicinal Plants by High-Performance Liquid Chromatography and Liquid Chromatography-Mass Spectrometry. Journal of Chromatography A 2004, 1046, 101–107.
- Song, Z.; Ji, Z.; Lo, C.; Dong, T.; Zhao, K.; Li, O.; Haines, C.; Kung, S.; Tsim, K. Chemical and Biological Assessment of a Traditional Chinese Herbal Decoction Prepared from Radix Astragali and Radix Angelicae sinensis: Orthogonal Array Design to Optimize the Extraction of Chemical Constituents. Planta Medica 2005, 70, 1222–1227.
- Wu, Y.; Baek, H.H.; Gerard, P.D.; Cadwallader, K.R. Development of a Meat-Like Process Flavoring from Soybean-Based Enzyme-Hydrolyzed Vegetable Protein (E-HVP). Journal of Food Science 2000, 65, 1220–1227.
- Box, G.E.P.; Behnken, D.W. Some New Three Level Designs for the Study of Quantitative Variables. Technometrics 1960, 2, 455–475.
- Vera-Candioti, L.; Olivieri, A.C.; Goicoechea, H.C. Simultaneous Multiresponse Optimization Applied to Epinastine Determination in Human Serum by Using Capillary Electrophoresis. Analytica Chimica Acta 2007, 595, 310–318.
- Kim, T.; Lee, S.; Kim, Y.; Kim, K.; Oh, S.; Lee, H. Aroma Dilution Method Using GC Injector Split Ratio for Volatile Compounds Extracted by Headspace Solid Phase Microextraction. Food Chemistry 2003, 83, 151–158.
- Feng, Y.; Cai, Y.; Sun-Waterhouse, D.; Cui, C.; Su, G.; Lin, L.; Zhao, M. Approaches of Aroma Extraction Dilution Analysis (AEDA) for Headspace Solid Phase Microextraction and Gas Chromatography–Olfactometry (HS-SPME–GC–O): Altering Sample Amount, Diluting the Sample or Adjusting Split Ratio? Food Chemistry 2015, 187, 44–52.
- Liu, J.; Liu, M.; He, C.; Song, H.; Guo, J.; Wang, Y.; Yang, H.; Su, X. A Comparative Study of Aroma-Active Compounds between Dark and Milk Chocolate: Relationship to Sensory Perception. Journal of the Science of Food & Agriculture 2014, 95, 1362–1372.
- Cao, Z.; Chen, X.; Chang, E.; Du, J. Effective Components of Chinese Herbal Compound Decoction and Maillard Reaction. Chinese Journal of Integrative Medicine 2009, 15, 224–228. (in Chinese)
- Ke, L.; Zhou, J.; Lu, W.; Gao, G.; Rao, P. The Power of Soups: Super-Hero or Team-Work. Trends in Food Science & Technology 2011, 22, 492–497.
- Lu, S.; Li, Y.; Chen, Y.; Zeng, H.; Zhang, L.; Zheng, X. Study on the Chemical Constituents of Volatile Oil from Different Parts of Embelia parviflora Wall. Ex A. DC by GC-MS. Medicinal Plant 2012, 1, 58–61.
- Piao, X.; Park, J.; Cui, J.; Kim, D.; Yoo, H. Development of Gas Chromatographic/Mass Spectrometry-Pattern Recognition Method for the Quality Control of Korean Angelica. Journal of Pharmaceutical & Biomedical Analysis 2007, 44, 1163–1167.
- Zheng, W.; Tao, Y.; Shi, J.; Hu, Y.; Shao, J.; Xiao, W. Optimize the Technique of Extracting Volatile Oil from Angelica sinensis by Steam Distillation. Modern Chinese Medicine 2013, 10, 879–882. (in Chinese)
- Liu, G.; Liu, Y.; Liu, K. Influences of Steam Distillation on the Isomerization of the Essential Oils of Angelica sinensis (Oliv.) Diels. Chinese Traditional Patent Medicine 2001, 11, 784–786. (in Chinese)
- Kim, M.; El-Aty, A.M.A.; Jeong, H.; Kang, B.; Shim, J. Determination of Volatile Flavor Components in Danggui Cultivars by Solvent Free Injection and Hydrodistillation Followed by Gas Chromatographic–Mass Spectrometric Analysis. Journal of Chromatography A 2006, 1116, 259–264.
- Kim, M.; El-Aty, A.M.A.; Jeong, H.; Kang, B.; Shim, J. Identification of Volatile Components in Angelica Species Using Supercritical-Co2 Fluid Extraction and Solid Phase Microextraction Coupled to Gas Chromatography-Mass Spectrometry. Biomedical Chromatography 2006, 20, 1267–1273.
- Tan, H.; Hu, D.; Song, J.; Xu, Y.; Cai, S.; Chen, Q.; Meng, Q.; Li, S.; Chen, S.; Mao, Q.; Xu, H. Distinguishing Radix Angelica sinensis from Different Regions by HS-SFME/GC–MS. Food Chemistry 2014, 186, 200–206.
- Shibamoto, T. Heterocyclic Compounds Found in Cooked Beef. Journal of Agricultural & Food Chemistry 1980, 28, 237–243.