ABSTRACT
Suan yu, a type of Chinese traditional fermented freshwater fish in solid form, is cheered among consumers due to its characteristic sensory flavour, primarily taste and smell. The purpose of this study was to investigate the presence of esterase activity of Lactobacillus plantarum 120, Staphylococcus xylosus 135, and Saccharomyces cerevisiae 31, in selecting appropriate processing means to accelerate the formation of the desired flavour in Suan yu. The results showed that all strains exhibited esterase activities in both intracellular and extracellular fractions against p-nitrophenyl (p-NP)-acetate, p-NP-butyrate, and p-NP-octanoate, and also that the maximum activity was observed in the intracellular fraction with p-NP-octanoate. Esterase activities of all strains were inhibited by low pH and low water activity (Aw), with the exception of L. plantarum 120 exhibiting an increase in intracellular activity with decreasing Aw. Moreover, L. plantarum 120 and S. xylosus 135 esterase were negatively affected by decreasing temperature, while a positive influence was observed on the intracellular esterase of S. cerevisiae 31. The results suggested that a low pH, a low Aw and a high temperature would be more appropriate for Suan yu processing to produce more flavour compounds which are acceptable by consumers.
Introduction
Suan yu is a type of Chinese fermented fish in solid form, which originates from the Miao and Dong nationalities areas. It is a traditional fermented fish product with higher protein, lower fat, lower biogenic amine, and a variety of free amino acids.[Citation1] Such products are commonly produced via a traditional homemade technology, including curing, salting, drying, and long-term anaerobic fermentation. Common carp or grass carp is usually used as the raw fish, with ingredients such as cooked corn or glutinous rice, carbohydrate, salt, and deferent kind of spice. The fish produced in this area is supposed to possess a characteristic flavour, which leads to its popularity among consumers.
Esters were detected as one of the predominant volatile compounds in traditional Suan yu.[Citation2] These are common volatile components of flavour in fermented products, providing the desirable fruity aroma to the overall profile of wines and dairy products.[Citation3,Citation4] Esterase and lipase are the main enzymes that are responsible for the formation of these compounds.[Citation5] More information is found about the enzymatic synthesis by microbial esterase in fermented products, such as yeast in wine and lactic acid bacteria (LAB) in cheese.[Citation5,Citation6] Thus, microflora may play an important role in the formation of esters in Suan yu.
Esterase (E.C. 3.1.1.3) are defined as the enzymes that normally catalyse the hydrolysis of water-soluble esters composed of short-chain fatty acids, including “simple” esters (e.g., ethyl acetate) and triglycerides, which could also synthesize esters under certain conditions.[Citation7] The primary impact of microbial esterase is probably to liberate free fatty acids (FFAs) and alcohols of esters. FFAs and alcohols are not only important flavour precursors of catabolic reactions which produce volatile compounds such as esters, but also contribute to overall aroma directly. Therefore, esterolytic activity during fermentation has the potentiality to either increase or decrease the amount of esters.
A large number of researches have been focused on the esterase activity of yeast,[Citation8] LAB[Citation9–Citation12] from dairy products, and LAB from wines.[Citation13,Citation14] A few authors also demonstrated the esterase activity of autochthonous cultures from fermented sausages. Mendoza et al.[Citation15] detected the esterase activity of Cryptococcus genus, Rhodosporidium diobovatum, Trichosporon japonicum, and Candida zeylanoides among 414 yeasts isolated from llama meat fermented sausages. Casaburi et al.[Citation16] reported high esterase activities among S. xylosus and S. equorum species from different types of Southern Italian fermented sausages. However, little information is available about the esterase activities of strains from fermented freshwater fish products.
Lactobacillus plantarum 120, Staphylococcus xylosus 135, and Saccharomyces cerevisiae 31 were isolated from the traditional Suan yu, which were the most appropriate strains owing to their technological and antimicrobial characteristics (unpublished). Zeng et al.[Citation1] studied the biochemical characteristics of L. plantarum 120, S. xylosus 135, and S. cerevisiae 31 autochthonous strains, among which S. cerevisiae 31 exhibited ester-forming activity and the others were not tested. It is not clear whether these strains are associated with the changes of esters concentration or not, during fermentation. The aim of this work was to investigate the presence of esterase activity of L. plantarum 120, S. xylosus 135, and S. cerevisiae 31 and to evaluate the effect of environmental parameters (pH, temperature, and water activity) on their activities, so as to determine the esterolytic systems of those microorganisms present in Suan yu.
Material and methods
Preparation of Suan yu samples
The samples were prepared according to the traditional techniques combined with modern biotechnologies. Fresh common carps (Cyprinus carpio L.) with weight of 2–2.5 kg were purchased from a local market, gutted, and eviscerated. The head, tail, scales, and spur were removed and cleaned using tap water. Then, the fish body were cut into 20–30 g pieces and cured with sucrose (2% w/w) and salt (3% w/w) at 0–4°C for 48 h. Afterwards, the pieces were dried at 60°C for 3 h. The roasted corn (25% w/w) was then divided into four batches and added with different single-starter culture that suspended in saline water, including S1 (L. plantarum 120), S2 (S. xylosus 135), S3 (S. cerevisiae 31), and a batch without inoculation (NS) as the control. After that, the fish pieces, roasted corn, and starter cultures were completely mixed in a glass container and sealed tightly with water surrounded. Each starter culture (7–9 log cfu/g) was inoculated to the fish pieces and fermented at 24°C for 5 weeks. The samples were withdrawn at each of the five different processing stages: raw, at the end of drying, at the end of first week, third week, and fifth week of fermentation, respectively.
About 1 g of each sample was added with 10 mL of potassium phosphate buffer (100 mM, pH 6.8). The mixture was homogenized for 30 s using an IKA T10 basic ULTRA-TURRAX homogenizer (IKA, Staufen, Germany) in ice water bath followed by filtration through four-layer gauze. The filtrate was separated by centrifugation (Sigma Laborzentrifugen, Model 4K15, Osterode, Germany) at 12,000 × g for 15 min at 4°C. The supernatant was obtained as the crude fish esterase, used immediately or stored at −18°C.
Preparation of cell extracts
L. plantarum 120 and S. xylosus 135 were separately subcultured twice according to the method of Hu et al.[Citation17] S. cerevisiae 31 was subcultured twice in yeast extract peptone dextrose (YPD) medium at 30°C for 24 h, containing: 1% (w/v) yeast extract, 2% (w/v) peptone, and 2% glucose (w/v).[Citation18] Supernatants were harvested as the extracellular fractions by centrifugation at 10,000 × g for 10 min at 4°C. At the same time, the left cells were washed twice with potassium phosphate buffer (100 mM, pH 6.8) and sonicated in the same buffer using a JY88-II ultrasonic homogenizer (Ningbo Scientz Biotechnology Co. Ltd., Ningbo, China) at 300 W (L. plantarum 120 for 5 min, S. xylosus 135 for 5 min, and S. cerevisiae 31 for 10 min, respectively). Whole cells and cellular debris were separated by centrifugation at 15,000 × g for 20 min at 4°C. The supernatant was obtained as the intracellular fraction, used either immediately or stored at −18°C. The concentrations of protein in the cell extracts were determined by the method of Bradford.[Citation19] The results are expressed as mg protein/mL.
Determination of pH and water activity (Aw)
The pH values of the samples were determined according to the Zeng et al.[Citation20] Aw was measured by a digital water activity meter (LabSwift-Aw, novasina, Switzerland).
Determination of esterase activity
Esterase activity was determined according to the procedure described by Matthews et al.[Citation14] with some modifications. Three p-NP-linked esters of varying carbon-chain length were used as substrates, including (1) p-NP-acetate, (2) p-NP-butyrate, and (3) p-NP-octanoate. The assay mixture contained 4.7 mL of 100 mM sodium phosphate buffer (pH 6.8), 100 μL of 26 mM p-NP ester (in ethanol), and 200 μL of cell-free extracts or supernatant or crude fish esterase. After the mixture was incubated at 30°C for 2 h, 900 μL of 0.1 M NaOH was added to adjust the pH to 7.0–7.2. Then, the liberated p-nitrophenol was quantified by measuring the absorbance at 410 nm using a spectrophotometer (Techcomp, UV-1000, Shanghai, China) and was quantified by comparing with p-nitrophenol standard, expressed as mg/mL. One unit (1U) of esterase activity was defined as 1 μmol of p-nitrophenol released by the crude enzyme in 1 h. Specific activities were expressed as U/mg protein.
Influence of pH, temperature, and Aw on esterase activity
To determine the influence of pH, 0.1 M McIlvane buffer was adjusted to pH 3.6, 4.0, 4.4, 5.4, and 6.8. All reactions were incubated at 30°C. The influence of temperature was studied using 0.1 M McIlvane buffer of pH 5.4. The assayed temperatures were 10, 20, 30, and 40°C in biochemical incubators. The influence of Aw was studied using 0.1 M McIlvane buffer of pH 5.4. Aw was altered by adding glycerol (w/v) using a water activity meter. The final glycerol concentrations were 0, 15, 30, and 45%, equivalent to Aw 0.93, 0.90, 0.88, and 0.86, respectively.
Determination of esters in Suan yu samples
Solid phase micro extraction (SPME) combined with gas chromatography mass spectrometry (GC-MS) was used to determine the esters of Suan yu samples.[Citation2] About 2 g of sample was mixed with 8 mL of NaCl saturated solution. The supernatant was introduced with 10 ppm of the internal standard 2,4,6-trimethyl pyridine (TCI, Tokyo, Japan) in a 20 mL vial fitted with a silicone septum. The fibre (65 μm, PDMS/DVB; Supelco, Bellefonte, PA, USA) was inserted into the head space of the sample vial and adsorbed at 60°C for 30 min, and then desorbed at 250°C for 3 min in the injection port.
TSQ Quantum XLS (Thermo Fisher Scientific, USA) was used for GC-MS analysis. The GC conditions were as follows; column: DB-WAX; 30 m × 0.25 mm × 0.25 μm; initial temperature: 40°C; initial time: 3 min; Rate1: 5°C/min; final temperature: 90°C; hold time: 0 min; rate 2: 10°C/min; final temperature 2: 230°C; and hold time 2: 7 min. The MS conditions were as follows: ionization mode: EI+; emission current: 80 µA; electron energy: 70 eV; scan range: 30–500 m/z; interface temperature: 250°C; source temperature: 200°C; carrier: He, 0.90 ml/min; and detector voltage: 1000 V. The esters were identified according to NIST2005 and Willey 7 standard libraries and retention index (RI). The concentration of the volatile compounds in the samples was calculated by comparing the peak area of each compound with that of the internal standard, expressed as μg/kg.
Statistical analysis
The data obtained were subjected to the analysis of variance. Mean differences were also evaluated by Duncan’s multiple range test (p < 0.05). Statistical analysis was performed using the SPSS statistic program (Version 19.0, SPSS Inc., Chicago, IL).
Results and discussion
Esterase activity of strains
The results for determining the esterase activity of different cell fractions in the dominate strains isolated from Suan yu are shown in . Short-chain fatty acids (≤C8) esters (p-NP-acetate, p-NP-butyrate and p-NP-octanoate) were selected as substrates according to the definition of esterase.[Citation7] All strains exhibited activities in both intracellular and extracellular fractions against different substrates. For each strain, the value of activity was significantly higher in the intracellular fraction when p-NP-octanoate was used as the substrate, while the extracellular fraction showed higher activity values with p-NP-acetate and p-NP-butyrate. These results suggested the presence of more than one esterase at each strain. A similar result was reported by Casaburi et al.[Citation16] who found that the intracellular fractions of S. xylosus and S. equorum isolated from Southern Italian fermented sausages were mainly active against p-NP-butyrate and p-NP-octanoate, while the extracellular extracts of most strains were only active against p-NP-butyrate. Moreover, the maximum activity (12.61, 15.01, and 10.90 U/mg protein, respectively) was observed in the intracellular fraction with p-NP-octanoate, in accordance with the result by Perez-Martin et al.,[Citation21] wherein 13 LAB strains from red wines showed the highest activity of esterase in the intracellular fraction.
Table 1. Results for esterase activity (U/mg protein) against different substrates in different cell fractions.
Influence of pH on esterase activity
Studies on the influence of pH, temperature, and water activity on the esterase activity were carried out in both intracellular and extracellular fractions from the three strains. Significant differences in esterase activities between the three strains and each strain at different pH values, temperatures, or water activities were observed (p < 0.05). The results are shown in –).
Figure 1. Effect of pH (1), temperature (2), and Aw (3) on the intracellular (a) and extracellular (b) esterase activity of the three strains (p < 0.05).
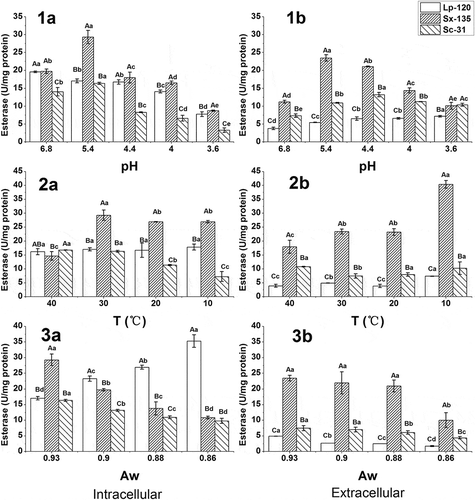
The effect of pH on the esterase activity against p-NP-octanoate is shown in 1). Significant differences in esterase activity, intracellular and extracellular, between three strains were observed. For intracellular fractions, each strain presented a trend to a decrease in activity, with pH value ranging from 6.8 to 3.6 (1a)). In addition, S. xylosus 135 showed the maximum activity at pH 5.4, reaching 29.32 U/mg protein. Compared with a rapid decrease in activities of S. xylosus 135 and S. cerevisiae 31 from pH 5.4 to 4.0, a slight decrease was observed in L. plantarum 120, which could be attributed to its stronger acid resistance. However, L. plantarum 120 showed a marked decrease in activity at pH 3.6, which meant this activity was also strongly inhibited at a low pH.
For extracellular fractions, S. xylosus 135 activity was also affected by low pH, exhibiting the maximum activity (23.47 U/mg protein) at pH 5.4. Though the activity of L. plantarum 120 and S. cerevisiae 31 increased slightly with decline of pH, it is still markedly lower than that of S. xylosus 135 (1b)). Therefore, esterase activities of all the strains were inhibited at a low pH, of which S. xylosus 135 was the greatest. Talon and Montel[Citation22] also reported that esterase activities of cell-free extracts (intracellular) and extracellular fractions from Staphylococci (including S. xylosus) were dramatically inhibited at pH 5.0, corresponding to only 2–3% of the maximum activity measured at pH 7.0. Moreover, the impact of acidic condition was relatively not drastic on L. plantarum 120, which was in agreement with Matthews et al.[Citation14] that Oenococcus oeni from wine appeared least influenced by the range of pH 6.5‒4.0. However, a drastic decrease was found in the intracellular esterase of Streptococcus thermophilus with decreasing pH from 8.0 to 5.5.[Citation23]
Influence of temperature on esterase activity
Similar to what happened with changes in pH, the response to temperature was strain dependent (2)). For intracellular fractions, S. xylosus 135 and S. cerevisiae 31 were more affected by temperature. From 40°C to 10°C, S. xylosus 135 presented a trend of increase in activity, quickly reaching maximum activity at 30°C, while the activity of S. cerevisiae 31 decreased with inducing temperature, showing the minimum value (7.27 U/mg protein) at 10°C. In addition, no significant differences were observed in the activity of L. plantarum 120 across a range of temperature from 40°C to 10°C (p > 0.05).
For extracellular fractions, the esterase activity of S. xylosus 135 was significantly higher than those of L. plantarum 120 and S. cerevisiae 31 for all cases. An increase in activity was observed in S. xylosus 135 with decreasing temperature, which reached its maximum activity at 10°C, close to 40 U/mg protein. Moreover, L. plantarum 120 was less negatively affected by temperature, showing only a slight increase in activity with decreasing temperature, while temperature had a little effect on S. cerevisiae 31 esterase activity.
Therefore, S. xylosus 135 was the most negatively affected by temperature, showing a significant increase in activity with decreasing temperature, whilst L. plantarum 120 was less negatively affected, exhibiting only a slight increase in activity with decreasing temperature. Perez-Martin et al.[Citation21] reported a similar result that Enterococcus faecium 1 (Ent1) showed an increased trend in esterase activity with a range of 45‒15°C, but an opposite result was reported by Liu et al.[Citation23] that decreasing temperature from 37°C to 25°C considerably reduced the intracellular esterase activity identified in S. thermophilus. In addition, a positive influence was observed in the intracellular faction of S. cerevisiae 31.
Influence of Aw on esterase activity
Aw response also varied between strains, and a decrease in esterase activity was observed for all of them at all Aw values (3)), except for intracellular fraction of L. plantarum 120 which showed an increase in activity with decreasing Aw. For intracellular fractions, the esterase activities of S. xylosus 135 and S. cerevisiae 31 were positively affected, whereas the esterase activity of L. plantarum 120 was the most negatively affected. There was no significant difference in activity between L. plantarum 120 and S. cerevisiae 31 at Aw 0.93. However, comparatively greater esterase activity was observed in L. plantarum 120 than the others at the beginning of Aw 0.90. At Aw 0.86, the esterase activity of L. plantarum 120 was 35.33 U/mg protein, which is double the minimum value measured at Aw 0.93, while the specific activity of S. xylosus 135 and S. cerevisiae 31 was 10.87 U/mg protein and 9.80 U/mg protein, respectively, with 37% and 60% of their respective maximum values.
For extracellular fractions, a clear trend to a decrease in activity with decreasing Aw was observed for all strains. For all the cases, S. xylosus 135 showed the highest activity and S. cerevisiae 31 followed. At Aw 0.86, the specific activity of three strains was 1.75 U/mg protein, 10.04 U/mg protein, and 4.42 U/mg protein, respectively, corresponding to 36%, 43%, and 59% of their respective maximum activities measured at Aw 0.93.
Therefore, a low Aw could inhibit the esterase activities of both S. xylosus 135 and S. cerevisiae 31 from intracellular and extracellular fractions, with a greater inhibition on S. xylosus 135. At the meantime, Aw had different effects on intracellular and extracellular esterase of L. plantarum 120. A high Aw inhibited the intracellular esterase, while a low Aw inhibited the extracellular esterase. An opposite result about LAB was reported by Liu et al.[Citation23] who found that decreasing water activity (from 0.99 to 0.80) considerably reduced the intracellular esterase I activity from S. thermophiles. Holland et al.[Citation3] reported that esterase of LAB released short-chain FFA from milk fat at high water activity and synthesized short-chain ethyl esters at low water activity during ripening.
Esterase activity of Suan yu samples
As shown in , the esterase activity of Suan yu samples inoculated with the above strains in different stages was determined accordingly. Values in the figure represent the relative esterase activity (%) with respect to a fish before fermentation at pH 6.8 and at 30°C. At the end of the first week of fermentation., only samples inoculated with L. plantarum 120 showed activity above 100%, while the activities of the other samples were about 50%. The sharp decrease of pH below 5 () might lead to the smaller activity of S2 and S3, and the activity above 100% of S1 might attribute to the higher intracellular activity () of L. plantarum 120 at the declined water activity nearly 0.89 (). All samples exhibit activities above 100% at the end of third week of fermentation, owing to increasing numbers of colony. For 5 weeks of fermentation, the activity of S2 increased to 155%, while a varied decline was observed in other samples. S3 showed the same trend in variation of esterase activity with NS during the fermentation. In general, Suan yu exhibited higher activities in some fermentation stages after inoculation with the three strains, respectively, compared to Suan yu without inoculation.
Esters of Suan yu samples
The changes of ester concentrations in Suan yu samples inoculated with the above strains during the processing are shown in . A significant difference of ester concentration in every batch of Suan yu was observed during the processing (p < 0.05). There were no significant differences between all Suan yu samples before fermentation. For 5 weeks of fermentation, all inoculated samples possessed more esters than NS. The results in this study suggested that these strains were associated with the changes of ester concentrations during fermentation. As starter cultures, they could improve the ester contents to enhance the overall profile of Suan yu.
Table 2. Ester concentrations of Suan yu samples during the processing.
Conclusion
All strains tested in this study exhibit esterolytic activity in both intracellular and extracellular fractions that may release C2–C8 fatty acids during fermentation. The esterase activity in different cell fractions was significantly affected by the environmental conditions of Suan yu (p < 0.05), including pH, temperature, and Aw. The results implied that a low pH, a low Aw, and a high temperature would be more appropriate for Suan yu processing with the production of more flavour compounds.
Conflicts of interest
The authors declare no conflicts of interest.
Funding
This research was financially supported by the earmarked fund for China Agricultures Research System, CARS-46-22 and National Natural Science Foundation of China, NFSC 31371823 and Collaborative Innovation Center of Food Safety and Quality Control in Jiangsu Province and 2014GB2C100297.
Additional information
Funding
References
- Zeng, X.F.; Xia, W.S.; Jiang, Q.X.; Yang, F. Effect of Autochthonous Starter Cultures on Microbiological and Physico-Chemical Characteristics of Suan yu, a Traditional Chinese Low Salt Fermented Fish. Food Control 2013, 33 (2), 344–351.
- Gao, P.; Wang, W.; Jiang, Q.; Xu, Y.; Xia, W. Effect of Autochthonous Starter Cultures on the Volatile Flavour Compounds of Chinese Traditional Fermented Fish (Suan yu). International Journal Food Science and Technology 2016, 51, 1630–1637.
- Holland, R.; Liu, S.Q.; Crow, V.L.; Delabre, M.L.; Lubbers, M.; Bennett, M.; Norris, G. Esterases of Lactic Acid Bacteria and Cheese Flavour: Milk Fat Hydrolysis, Alcoholysis and Esterification. International Dairy Journal 2005, 15, 711–718.
- Yue, T.X.; Chi, M.; Song, C.Z.; Liu, M.Y.; Meng, J.F.; Zhang, Z.W.; Li, M.H. Aroma Characterization of Cabernet Sauvignon Wine from the Plateau of Yunnan (China) with Different Altitudes Using SPME-GC/MS. International Journal of Food Properties 2015, 18, 1584–1596.
- Liu, S.Q.; Holland, R.; Crow, V.L. Esters and Their Biosynthesis in Fermented Dairy Products: A Review. International Dairy Journal 2004, 14, 923–945.
- Carr, F.J.; Chill, D.; Maida, N. The Lactic Acid Bacteria: A Literature Survey. Critical Reviews in Microbiology 2002, 28, 281–370.
- Bornscheuer, U.T.;. Microbial Carboxyl Esterases: Classification, Properties and Application in Biocatalysis. FEMS Microbiology Reviews 2002, 26, 73–81.
- Bintsis, T.; Vafopoulou-Mastrojiannaki, A.; Litopoulou-Tzanetaki, E.; Robinson, R. Protease, Peptidase and Esterase Activities by Lactobacilli and Yeast Isolates from Feta Cheese Brine. Journal of Applied Microbiology 2003, 95, 68–77.
- Oliszewski, R.; Medina, R.; Gonzalez, S.; Perezchaia, A. Esterase Activities of Indigenous Lactic Acid Bacteria from Argentinean Goats’ Milk and Cheeses. Food Chemistry 2007, 101, 1446–1450.
- Gonzalez, L.; Sacristan, N.; Arenas, R.; Fresno, J.M.; Eugenia Tornadijo, M. Enzymatic Activity of Lactic Acid Bacteria (with Antimicrobial Properties) Isolated from a Traditional Spanish Cheese. Food Microbiology 2010, 27, 592–597.
- Abeijon Mukdsi, M.C.; Medina, R.B.; Katz, M.B.; Pivotto, R.; Gatti, P.; Gonzalez, S.N. Contribution of Lactic Acid Bacteria Esterases to the Release of Fatty Acids in Miniature Ewe’s Milk Cheese Models. Journal of Agriculture and Food Chemistry 2009, 57, 1036–1044.
- Macedo, A.C.; Tavares, T.G.; Malcata, F.X. Esterase Activities of Intracellular Extracts of Wild Strains of Lactic Acid Bacteria Isolated from Serra da Estrela Cheese. Food Chemistry 2003, 81, 379–381.
- Sumby, K.; Grbin, P.; Jiranek, V. Characterization of Estcoo8 and Estc34, Intracellular Esterases, from the Wine-Associated Lactic Acid Bacteria Oenococcus oeni and Lactobacillus hilgardii. Journal of Applied Microbiology 2013, 114, 413–422.
- Matthews, A.; Grbin, P.R.; Jiranek, V. Biochemical Characterisation of the Esterase Activities of Wine Lactic Acid Bacteria. Applied Microbiology and Biotechnology 2007, 77, 329–337.
- Mendoza, L.M.; Padilla, B.; Belloch, C.; Vignolo, G. Diversity and Enzymatic Profile of Yeasts Isolated from Traditional Llama Meat Sausages from North-Western Andean Region of Argentina. Food Research International 2014, 62, 572–579.
- Casaburi, A.; Villani, F.; Toldra, F.; Sanz, Y. Protease and Esterase Activity of Staphylococci. International Journal of Food Microbiology 2006, 112, 223–229.
- Hu, Y.J.; Xia, W.S.; Ge, C.R. Characterization of Fermented Silver Carp Sausages Inoculated with Mixed Starter Culture. Lwt-Food Science and Technology 2008, 41, 730–738.
- Liu, N.; Qin, Y.; Song, Y.Y.; Tao, Y.S.; Sun, Y.; Liu, Y.L. Aroma Composition and Sensory Quality of Cabernet Sauvignon Wines Fermented by Indigenoussaccharomyces cerevisiaestrains in the Eastern Base of the Helan Mountain, China. International Journal of Food Properties 2015, 1–15. DOI:10.1080/10942912.2015.1089278
- Bradford, M.M. A Rapid and Sensitive Method for the Quantitation of Microgram Quantities of Protein Utilizing the Principle of Protein–Dye Binding. Analytical Biochemistry 1976, 72, 248–254.
- Zeng, X.F.; Xia, W.; Jiang, Q.; Yang, F. Chemical and Microbial Properties of Chinese Traditional Low-Salt Fermented Whole Fish Product Suan yu. Food Control 2013, 30, 590–595.
- Perez-Martin, F.; Sesena, S.; Izquierdo, P.M.; Palop, M.L. Esterase Activity of Lactic Acid Bacteria Isolated from Malolactic Fermentation of Red Wines. International Journal of Food Microbiology 2013, 163, 153–158.
- Talon, R.; Montel, M.C. Hydrolysis of Esters by Staphylococci. International Journal of Food Microbiology 1997, 36, 207–214.
- Liu, S.Q.; Holland, R.; Crow, V.L. Purification and Properties of Intracellular Esterases from Streptococcus thermophilus. International Dairy Journal 2001, 11, 27–35.