ABSTRACT
This study deals with the efficacy of Origanum onites, Salvia officinalis, and Mentha piperita essential oils (EOs) and their mixture (1:1:1, v/v/v) against ochratoxigenic P. verrucosum D-99756 and modelling the effects of EOs on fungal growth using modified Gomperz and logistic models. O. onites, S. officinalis, and M. piperita EOs were used at their minimal lethal concentration levels at 65, 125, and 250 µL/mL, respectively, which were determined in our preliminary studies. Inactivation of fungi sporulation by EO treatments was higher than 50–60% within 48 h, while 78.50–81.41% lethality was observed at the end of the 168 h for all of the tested EOs. The most effectiveness time for lethal effect was found at 84 h for O. onites and S. officinalis, while it was 168 h for mix EOs (p < 0.05). The modified Gompertz model successfully produced better fits than the logistic model as evidenced by the root mean square error, the modelling efficiency, and accuracy efficiency. In conclusion, data confirmed the potential use of plant and spice EOs for their fungistatic and fungicidal effects in food systems.
Introduction
Foods and feeds are frequently contaminated with fungi and the associated toxins generated by some of them during storage, transport, and post-harvest processing, and they are responsible for the loss of quality, quantity, and nutrition composition.[Citation1] Aspergillus, Penicillium, and Fusarium are the most known fungal genera which are associated with foodborne diseases or food spoilage. Penicillium species have been known to be the most important mycotoxin producers, while Penicillium verrucosum is the major producer of ochratoxin-A (OTA) and citrinin.[Citation2,Citation3] It is capable of growing in a variety of foods and beverages including beer, chocolate, coffee, dried fruits, grape juice, pork, poultry, cheese, and wine[Citation4] and has been linked to several health risks such as carcinogenic, teratogenic, and immunotoxic effects.[Citation5] Moreover, economic and health problems associated with fungi toxins are an important issue for food industry.[Citation6]Therefore, novel food preservation techniques as alternative to conventional techniques like heat treatment, drying, and chemical preservation have been investigated in food industry due to the increasing demand by consumers for tasty, nutritive, and natural food products.[Citation7] Recently, essential oils (EOs) have considerable attention as antifungal and antimicrobial agents in order to control toxin-producing microorganisms in foods, both for the increasing interest of consumers for additives from natural sources and for increasing concern about potentially harmful synthetic additives.[Citation8] EOs, containing plant secondary metabolites including monoterpens, sesquitepenes, phenyl propanoids, alcohols, aldehydes, esters, ketones, phenols, and oxides, are characterised with their antimicrobial activity.[Citation7] Most of them and their active components have been classified as Generally Recognized as Safe (GRAS) for food preparations.[Citation9]
Predictive food microbiology has gained interest worldwide for improving food safety and quality. Mathematical models have been used in describing the behaviour (growth, survival, inactivation, etc.) of microorganism in food systems.[Citation9–Citation11] Mathematical model enables the prediction of the effect of changing treatment conditions on the microbial evaluation when a suitable model has been constructed and properly validated. There are various models that are classified as primary, secondary, and tertiary for prediction of behaviour of microorganisms.[Citation12] Modified Gompertz, logistic, Weibull, and Baranyi equations are among the most widely used models that have been proposed to describe the nonlinear survival curves of microorganisms.[Citation12–Citation15] Recent studies have indicated that logistic and modified Gompertz models were successfully applied for the description of microbial survival, inactivation, and growth.[Citation13,Citation14,Citation16] Although there have been numerous studies about the inhibition of molds by EOs,[Citation6,Citation7,Citation17] only a few reports on modelling the effect of EOs on growth of toxigenic molds like P. verrucosum are available in the literature.[Citation5,Citation17] Therefore, the objective of the present study was (i) to evaluate the inhibition kinetics of fresh EOs from Origanum onites, Salvia officinalis, and Mentha piperita against P. verrucosum at minimal lethal effect levels determined in our preliminary studies, (ii) to determine the possible synergistic effects between EOs, (iii) to describe the inactivation kinetics of P. verrucosum treated with EOs using modified Gompertz and logistic models, and finally (iv) to compare the goodness of fit of applied models.
Material and methods
Essential oils
Salvia officinalis L., Mentha piperita L., and Origanum onites L. EOs were obtained from Botalife, İzmir-Turkey. The plant species were selected on the basis of their antifungal activities which have been demonstrated by previous studies.[Citation18] Minimal Lethal Concentrations (MLC) for O. onites, S. officinalis, and M. piperita against P. verrucosum were 65, 125, and 250 µL/mL, respectively, which were determined in our preliminary studies.[Citation19,Citation20] MLC was selected as the lowest concentration at which no growth occurred on the plates. The concentrations of the EOs were prepared using methanol. The mixes of the EOs were obtained by their incorporation in equal volumes (1:1:1, v/v/v).
Culture media and preparation of inoculums
In the present study, a toxigenic strain of P. verrucosum (D-99756) was chosen since it is responsible for production of ochratoxin A (OTA). The microorganism isolated from Kashar cheese was obtained from Istanbul Technical University, Faculty of Chemical and Metallurgical Engineering, Food Engineering Department, Turkey. P. verrucosum was cultured in Yeast Extract Agar (Merck, Darmstadt, Germany) at 25°C in the dark for 8 days. The conidial suspension was prepared according to procedure followed by Cabanas et al.[Citation21] It was adjusted to approximately 106–107 viable spores per mL. The pour plate method was used in order to confirm the initial spore counts.
Kinetics of inhibition by EOS
Kinetic assay was carried out according to the method described by Rasooli et al.[Citation22] with some modifications. Slants were scraped gently using a sterile needle and washed with 7 mL of sterile physiological saline solution (0.85%) containing Tween-80 (0.1% v/v). Then the suspension was collected in sterile tubes. The viable spore counts were controlled using pour plate method after determining of spore numbers approximately by haemocytometer. Five millilitres of the spore suspensions including 106–107 spores/mL were transferred to sterile tubes and 0.05 mL of EOs at MLC levels. These tubes were shaken and incubated at 25°C with 12-h intervals for 168 h. One mL of the reaction mixtures was taken and diluted until two-fold. Three parallel inoculations were made on sterile Yeast Extract agar plates and then incubated for 48 h at 25°C. After the incubation period, fungal colonies were counted and expressed as percent inhibition according to the following formula:
where FC is the average fungal colony in control group (log cfu/g); FT is the average fungal colony in EOs treated group (log cfu/g).
Modelling of fungal growth
The nonlinear equations were used to fit the growth data of P. verrucosum by nonlinear regression with a Marquardt algorithm, to minimise the sum of the squares of the differences between the experimental and predicted values, which were used to calculate the set of parameters and regression coefficient (R2).[Citation16] Fungal growth modelling and growth parameters were performed with the modified Gompertz (Eq. 2) and logistic (Eq. 3) model proposed by Zwietering et al.[Citation16] and Tornuk et al.[Citation12]
where N is the number of P. verrucosum at time t; N0 is the initial number (t = 0) of P. verrucosum; a is the count increment as time increases indefinitely, that is, the number of log cycles of fungal growth (log cfu/g); t is incubation time (hour); B is the specific growth rate at time m (1/hour); m is the time at which the absolute growth rate is at a maximum (hour).
where N is the number of P. verrucosum at time t; N0 is the initial number (t = 0) of P. verrucosum; a is the count increment as time increases indefinitely; d is a dimensionless parameter; c is specific growth rate (1/hour). Additionally, the maximum specific inactivation rates (µ) (log cfu/g) for modified Gompertz and logistic model were calculated using Eqs. 4 and 5, respectively.
Model comparison
The root mean square error (RMSE) and the modelling efficiency (Ef), accuracy factor (Af), and bias factor (Bf) were used to compare the performance of mathematical models used in this study for inactivation kinetics of P. verrucosum. These parameters provide information to identify the differences between experimental data and the model estimates.[Citation14,Citation23,Citation24] These parameters were calculated as follows:
where Nexp,i (log cfu/g) is the number of P. verrucosum at time t; Npre,i (log cfu/g) is the predicted number of P. verrucosum at time t; Nexp,ave (log cfu/g) is the average of the number of P. verrucosum at time t; n is the number of data points; nu is the number of model parameters.
Data analysis and statistics
Each parameter was tested in duplicate samples with seven replications. Modified Gompertz and logistic model parameters were calculated using Sigma Plot software version 12.0 (SysStat Software, Inc., Point Richmond, California, USA). Collected data were subjected to statistical analyses using SPSS statistical package software (SPSS Inc. Chicago, Illinois). Two way analysis of variance (ANOVA) was used to evaluate the effect of EOs (O. onites, S. officinalis, and M. piperita) and incubation periods (from 0 to 168 h). When significant (p < 0.05) effect was found, the mean values were further analysed using Duncan.
Results and discussion
Fungicidal kinetic of the essential oils
Fungicidal kinetics (MLC levels) of O. onites, S. officinalis, and M. piperita EOs were 65, 125, and 250 µL/mL, respectively, against P. verrucosum after 7 days (). Methanol (control) did not show any inhibitory effect on P. verrucosum. The EOs of O. onites, S. officinalis, and mix (the mixture EOs of O. onites, S. officinalis, and M. piperita) had higher antifungal activity than M. piperita EO at the end of incubation period (p < 0.05). Average 80% lethal effect was observed within 84 h in the case of treatment with O. onites and S. officinalis EOs. The antifungal kinetics of O. onites and S. officinalis EOs for a period of 84–168 h weren’t significantly different (p > 0.05). The rapid killing rate was observed at exposure time of 84 h for treatment of O. onites and S. officinalis EOs, respectively, and O. onites EO had stronger antifungal activity than S. officinalis EOs against the tested fungi. High antifungal efficiency of O. onites oil could be attributed to high proportion of oxygenated monoterpenes. EOs rich in relatively oxygenated monoterpenes have stronger antifungal activities when compared to EOs rich in monoterpene hydrocarbons as well as sesquiterpenes.[Citation25] Additionally, O. onites contains phenolic components, such as carvacrol and its precursors, terpinene, and cymene.[Citation26] These phenolic components may also contribute to its antifungal activity since they may interfere with some cell wall enzymes like chitin synthase/chitinase as well as α- and β-glucanases of the fungus.[Citation27] Regarding the S. officinalis oil, the composition and antifungal spectrum of EOs of the Salvia species have also been studied.[Citation18,Citation28] Antifungal activity of Salvia genus oils could be attributed to the presence and synergistic effect of 1,8-cineole, camphor, and borneol as major constituents.[Citation29]
Figure 1. Percent inhibition (%) of Penicillium verrucosum treatment with M. piperita, O. onites, S. officinalis EOs, and their mix (1:1:1, v/v/v) during 168 h.
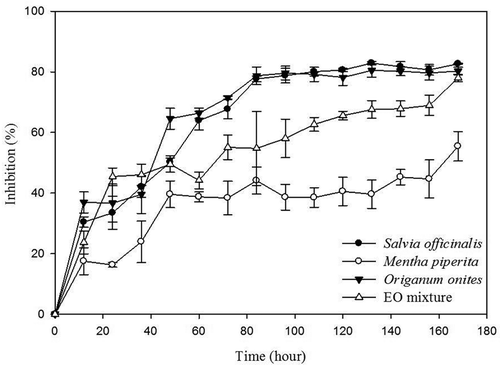
M. piperita oil at 250 μL/mL resulted in approximately 50% inhibition at the end of the incubation time. The antifungal effect of this plant extract may possibly be due to the presence of menthol, menthone, and neomenthol that are oxygenated monoterpenes.[Citation30] Antimicrobial activities of M. piperita oil have also been previously investigated by Iscan et al.[Citation31] who reported that menthol as a major compound of the EO showed weak antifungal activity at a concentration of 5 mg/mL.
Average 60–80% lethal effects were observed after 96 h of the EO mix treatment, and it was permanent up to the incubation period. The lethality (79–87%) was observed at the end of the 168 h for O. onites, S. officinalis, and mix EOs. The individual lethality powers of S. officinalis or O. onites were found higher than the lethality of the mixture at 156 h (p < 0.05); however, percent inhibition of individual EO and mixture was found similar at the end of incubation period (p > 0.05). Synergistic effects of some compounds in EOs have been shown in some studies. Hossain et al.[Citation5] reported that the highly effective oregano and thyme combination exhibited a synergistic effect against all the fungi except for Aspergillus niger. Another study carried out by Stevic et al.[Citation32] stated that a synergistic effect between carvacrol and thymol found in oregano and thyme showed antifungal activity against Penicillium and Fusarium species. Application of a certain combination of EO constituents can improve the efficacy of EOs against pathogenic microorganisms.[Citation33]
Modelling of fungal growth
The inactivation of P. verrucosum growth in the presence of the EOs during 168 h was fitted with modified Gompertz and logistic models, and model parameters from models were computed (). The predicted data from obtained model parameters were compared with the actual observed experimental data in . The stated models reasonably described the growth curve exhibited by P. verrucosum in the presence of EOs considering R2 values except the inactivation treatment using EOs mix (R2 = 0.844 and 0.870). According to modified Gompertz model, O. onites essential oil and mixture of EOs had the highest negative impact on the growth of P. verrucosum by decreasing the specific growth rate (B), whereas, S. officinalis and M. piperita were found to be less effective EOs due to increase the B values. Regarding the absolute growth rate (m), M. piperita and O. onites EOs were found to be the most effective due to increasing their lag time up to 20 h. The combination of EOs was found less effective on the extended of lag phase compared to individual EO. Regarding of logistic model parameters, the values of parameters a, d, and c of the model changed depending on the types of EOs.
Table 1. Modified Gompertz and logistic model parameters derived for survival of P. verrucosum treated to three different EOs and mixture of EOs and performance parameters of models.
Figure 2. Fitting of the modified Gompertz and logistic models to the experimental data obtained from the survival of P. verrucosum treatment with M. piperita (a), O. onites (b), S. officinalis (c) EOs, and their mix (d) (1:1:1, v/v/v) during 168 h.
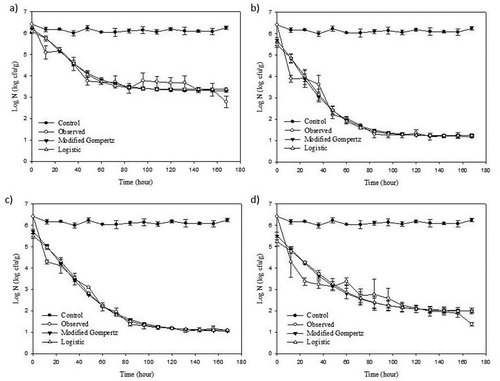
The maximum specific inactivation rate (µ) values obtained from modified Gompertz and logistic models were found between -0.052 to -0.081 and -0.045 to -0.074, respectively. The negative sign of µ expresses inactivation. The µ values obtained from both of models generally showed a similar trend for three individual EOs, but considering the growth of mold in the presence of combination of EOs, µ value obtained with modified Gompertz was found lower than that obtained with logistic model. When S. officinalis was used for inhibition of P. verrucosum, the lowest µ value obtained with both of models was found.
For the performance comparison of applied models, Toğrul and Arslan[Citation23] stated that the higher Ef and the lower RMSE values indicate the better goodness of an individual model. In this respect, the RMSE values (0.287–0.453) obtained with modified Gompertz model were lower than logistic model values (0.313–0.491) for all treatment. However, according to RMSE values, modified Gompertz and logistic models described the relationship with a good fit for O. onites and M. piperita EOs, respectively. Similar trend was shown for Ef values that were calculated with predictive values obtained by modified Gompertz model, which was found the highest for O. onites. The modified Gompertz model for the inhibition of P. verrucosum in the presence of EOs had higher Ef and lower RMSE indicating that the model provided reasonable good fits to the data. The accuracy factor (Af) and bias factor (Bf) values indicate the exact match between experimental data and model estimates, and a predictive model would ideally have Af = Bf = 1.[Citation24] Modified Gompertz and Logistic models showed better accuracy for O. onites and S. officinalis treatment. The Af values of models for M. piperita and EOs mixture were just out of the acceptable upper limit as 1.6, considering Af typically increases by 0.1–0.15 for every variable in the model.[Citation34] Bf values of the both models were found between 0.977 and 1.037, indicating that the models gave slightly ‘fail-safe’ predictions due to Bf value above the zero.[Citation24] For all that, Bf values of models were found within the proposed acceptable limits, that is, 0.75–1.25.[Citation35] Thus, applied models were ensured the best performance scores regarding Bf values.
Conclusion
The results of this work indicated that M. piperita, O. onites, and S. officinalis had antifungal properties. The mycelial growth of P. verrucosum could be controlled by O. onites, S. officinalis, and/or their mixture in the storage of foodstuffs, and nearly 84 h have been required for fungicidal activity of these plant oils. The application of S. officinalis and O. onites oils that possess antifungal activity on food can enhance their shelf life by controlling fungal growth. The need for protection of food and feedstuffs against Penicillium sp. should pay attention of food industries to conduct further experiments including minimal inhibitory and minimal fungicidal concentrations and safety data of EOs in food preservation. The modified Gompertz and logistic models had a good fit in order to describe the experimental data with relatively high R2 values except the treatment with EO mixture. Statistical indices suggested that the modified Gompertz model allowed more accurate description of the inactivation kinetic of P. verrucosum with EOs.
References
- Prakash, B.; Kedia, A.; Mishra, P.K.; Dubey, N.K. Plant Essential Oils as Food Preservatives to Control Molds, Mycotoxin Contamination and Oxidative Deterioration of Agri-Food Commodities–Potentials and Challenges. Food Control 2015, 47, 381–391.
- Rundberget, T.; Skaar, I.; Flaoyen, A. The Presence of Penicillium and Penicillium Mycotoxins in Food Wastes. International Journal of Food Microbiology 2004, 90, 181–188.
- Amezqueta, S.; Gonzales-Penas, E.; Murillo-Arbizu, M.; De Cerain, A.L. Ochratoxin A Decontamination: A Review. Food Control 2009, 20, 326–333.
- Clark, H.E.; Snedeker, S.M.; Ochratoxin, A. Its Cancer Risk and Potential for Exposure. Journal of Toxicology and Environmental Health 2006, 9, 265–296.
- Hossain, F.; Follett, P.; Vu, K.D.; Harich, M.; Salmieri, S.; Lacroix, M. Evidence for Synergistic Activity of Plant-Derived Essential Oils against Fungal Pathogens of Food. Food Microbiology 2016, 53, 24–30.
- Cisarova, M.; Tancinova, D.; Medo, J. Antifungal Activity of Lemon, Eucalyptus, Thyme, Oregano, Sage and Lavender Essential Oils against Aspergillus niger and Aspergillus tubingensis Isolated from Grapes. Potravinarstvo 2016, 10(1), 83–88.
- Asensio, C.M.; Gallucci, N.; Oliva, M.D.L.M.; Demo, M.S.; Grosso, N.R. Sensory and Bio‐Chemical Preservation of Ricotta Cheese Using Natural Products. International Journal of Food Science and Technology 2014, 49(12), 2692–2702.
- Wang, D.C.; Sun, S.H.; Shi, L.N.; Qiu, D.R.; Li, X.; Wei, D.S.; Zhang, Y.M.; Qin, J.C. Chemical Composition, Antibacterial and Antioxidant Activity of the Essential Oils of Metaplexis Japonica and Their Antibacterial Components. International Journal of Food Science and Technology 2015, 50(2), 449–457.
- Burt, S.; Essential Oils: Their Antibacterial Properties and Potential Applications in Foodsea Review. International Journal of Food Microbiology 2004, 94, 223–253.
- Swinnen, I.A.M.; Bernaerts, K.; Dens, E.J.; Geeraerd, A.H.; Van Impe, J.F. Predictive Modelling of the Microbial Lag Phase: A Review. International Journal of Food Microbiology 2004, 94(2), 137–159.
- Ferrer, J.; Prats, C.; Lopez, D.; Vives-Rego, J. Mathematical Modelling Methodologies in Predictive Food Microbiology: A SWOT Analysis. International Journal of Food Microbiology 2009, 134(1), 2–8.
- Tornuk, F.; Ozturk, I.; Sagdic, O.; Yilmaz, A.; Erkmen, O. Application of Predictive Inactivation Models to Evaluate Survival of Staphylococcus aureus in Fresh-Cut Apples Treated with Different Plant Hydrosols. International Journal of Food Properties 2014, 17(3), 587–598.
- Chen, H.; Use of Linear, Weibull, and Log-Logistic Functions to Model Pressure Inactivation of Seven Foodborne Pathogens in Milk. Food Microbiology 2007, 24(3), 197–204.
- Yilmaz, M.T.; Identifiability of Baranyi Model and Comparison with Empirical Models in Predicting Effect of Essential Oils on Growth of Salmonella typhimurium in Rainbow Trout Stored under Aerobic, Modified Atmosphere and Vacuum Packed Conditions. African Journal of Biotechnology 2013, 10(38), 7468–7479.
- Basak, S.; Guha, P. Modelling the Effect of Essential Oil of Betel Leaf (Piper betle L.) on Germination, Growth, and Apparent Lag Time of Penicillium expansum on Semi-Synthetic Media. International. Journal of Food Microbiology 2015, 215, 171–178.
- Zwietering, M.H.; Jongenburger, I.; Rombouts, F.M.; Van’t Riet, K. Modeling of the Bacterial Growth Curve. Applied and Environmental Microbiology 1990, 56(6), 1875–1881.
- Ibrahim, N.A.; El-Sakhawy, F.S.; Mohammed, M.M.; Farid, M.A.; Abdel-Wahed, N.A.; Deabes, D.A. Chemical Composition, Antimicrobial and Antifungal Activities of Essential Oils of the Leaves of Aegle marmelos (L.) Correa Growing in Egypt. Journal of Applied Pharmaceutical Science 2015, 5(2), 1–5.
- Pinto, E.; Salgueiro, R.; Cavaleiro, C.; Palmeira, A.; Goncalves, M.J. In Vitro Susceptibility of Some Species of Yeasts and Filamentous Fungi to Essential Oils of Salvia officinalis. Industrial Crops and Products 2007, 26, 135–141.
- Ozcakmak, S.; Gul, O.; Dervisoglu, M.; Yilmaz, A.; Sagdic, O.; Arici, M. Comparison of the Effect of Some Essential Oils on the Growth of Penicillium verrucosum and Its Ochratoxin a Production. Journal of Food Processing and Preservation 2017, DOI: 10.1111/jfpp.13006
- Ozcakmak, S.; Dervisoglu, M.; Yilmaz, A. Antifungal Activity of Lemon Balm and Sage Essential Oils on the Growth of Ochratoxigenic Penicillium verrucosum. African Journal of Microbiolog Research 2012, 6(12), 3079–3084.
- Cabanas, R.; Abarca, M.L.; Bragulat, M.R.; Cabanes, F.J. In Vitro Activity of Imazalil against Penicillium expansum: Comparison of the CLSI M38-A Broth Microdilution Method with Traditional Techniques. International Journal of Food Microbiology 2009, 129, 26–29.
- Rasooli, I.; Mohammad, B.R.; Allameh, A. Growth Inhibition and Morphological Alterations of Aspergillus niger by Essential Oils from Thymus eriocalyx and Thymus X-Porlock. Food Control 2006, 17, 359–364.
- Toǧrul, H.; Arslan, N. Mathematical Model for Prediction of Apparent Viscosity of Molasses. Journal of Food Engineering 2004, 62(3), 281–289.
- Bover-Cid, S.; Belletti, N.; Garriga, M.; Aymerich, T. Model for Listeria monocytogenes Inactivation on Dry-Cured Ham by High Hydrostatic Pressure Processing. Food Microbiology 2012, 8(4), 804–809.
- Kotan, R.; Kordali, S.; Cakir, A.; Kesdek, M.; Kaya, Y.; Kilic, H. Antimicrobial and Insecticidal Activities of Essential Oil Isolated from Turkish Salvia Hydrangea DC. Ex Benth. Biochemical Systematics and Ecology 2008, 36, 360–368.
- Ocak, I.; Çelik, A.; Özel, M.Z.; Korcan, E.; Konuk, M. Antifungal Activity and Chemical Composition of Essential Oil of Origanum hypericifolium. International Journal of Food Properties 2012, 15(1), 38–48.
- Sokovic, M.; Tzakou, O.; Pitarokili, D.; Couladis, M. Antifungal Activities of Selected Aromatic Plants Growing Wild in Greece. Nahrung/Food 2002, 46, 317–320.
- Ozkan, G.; Sagdic, O.; Gokturk, R.S.; Unal, O.; Albayrak, S. Study on Chemical Composition and Biological Activities of Essential Oil and Extract from Salvia pisidica. LWT - Food Science and Technology 2010, 43, 186–190.
- Tepe, B.; Donmez, E.; Unlu, M.; Candan, F.; Daferera, D.; Vardar-Unlu, G.; Polissiou, M.; Sokmen, A. Antimicrobial and Antioxidative Activities of the Essential Oils and Methanol Extracts of Salvia Cryptantha (Montbret Et Aucher Ex Benth.) and Salvia multicaulis (Vahl). Food Chemistry 2004, 84(4), 519–525.
- Rohloff, J.; Monoterpene Composition of Essential Oil from Peppermint (Mentha× piperita L.) with Regard to Leaf Position Using Solid-Phase Microextraction and Gas Chromatography/Mass Spectrometry Analysis. Journal of Agriculture and Food Chemistry 1999, 47(9), 3782–3786.
- Iscan, G.; Kirimer, N.; Kurkcuoglu, M.; Baser, K.H.C.; Demirci, F. Antimicrobial Screening of Mentha piperita Essential Oils. Journal of Agriculture and Food Chemistry 2002, 50, 3943–3946.
- Stevic, T.; Beric, T.; Savikin, K.; Sokovic, M.; Godevac, D.; Dimkic, I.; Stankovic, S. Antifungal Activity of Selected Essential Oils against Fungi Isolated from Medicinal Plant. Industrial Crops and Products 2014, 55, 116–122.
- Tajkarimi, M.M.; Ibrahim, S.A.; Cliver, D.O. Antimicrobial Herb and Spice Compounds in Food. Food Control 2010, 21, 1199–1218.
- Ross, T.; Dalgaard, P.; Tienungoon, S. Predictive Modelling of the Growth and Survival of Listeria in Fishery Products. International Journal of Food Microbiology 2000, 62, 231–245.
- Dalgaard, P.; Fresh and Lightly Preserved Seafood. In Shelf-Life Evaluation of Foods; Man, C.M.D.; Jones, A.A.; Eds.; Aspen Publishers, Inc.: London, UK, 2000; 110–139 pp.