ABSTRACT
The present study reports the antioxidant and anti-proliferative activities of Polygonum minus leaf extracts obtained through sequential extraction using four solvents of varying polarities (i.e. hexane (HX), ethyl acetate (EA), methanol, and water). The antioxidant potential was evaluated by measuring the total phenolic content (TPC), total flavonoid content (TFC), ferric reducing antioxidant power (FRAP), 2,2′-azinobis-3-ethylbenzothiazoline-6-sulphonic acid (ABTS) radical-scavenging, 1,1-diphenyl-2-picryl hydrazyl (DPPH) radical-scavenging, superoxide anion and nitric oxide scavenging, ferrous ion-chelating (FIC), and cellular antioxidant activity (CAA) assays. The highest antioxidant potential was generally shown by the methanol extract (PM-MeOH). PM-MeOH exhibited the highest values for TPC (174.00 ± 0.18 mg GAE/g), TFC (53.19 ± 0.71 mg GAE/g), FRAP (1728.33 ± 0.96 µmol Fe2+/g), ABTS (226.25 ± 4.25 µmol TE/g), DPPH (1276.81 ± 7.08 µmol TE/g), and nitric oxide scavenging assays (IC50, 675 ± 32.33 µg/mL). In the CAA assay, PM-MeOH dose-dependently inhibited the peroxyl radical-induced oxidation of 2ʹ,7ʹ-dichlorodihydrofluorescein (DCFH2) to 2ʹ,7ʹ-dichlorofluorescein (DCF) in HCT116 cells, with an EC50 value of 263.92 ± 21.60 µg/mL. Liquid chromatography and mass spectrometry analyses of PM-MeOH suggested the presence of tannins and flavonoids including apigetrin, hyperoside, isoquercetin, astragalin, miquelianin, quercetin, and quercitrin. P. minus hexane (PM-HX) and ethyl acetate (PM-EA) extracts showed selective cytotoxicity towards HCT116 with IC50 values of 40.00 ± 0.83 µg/mL and 43.18 ± 0.67 µg/mL, respectively. Taken together, these results highlight the potential of P. minus as a source of bioactive phytochemicals that may be useful in cancer therapeutics and nutraceutical industry.
Introduction
Chronic diseases, which include cardiovascular diseases, cancers, respiratory diseases, and diabetes, are the leading cause of disability and mortality globally. According to the World Health Organization (WHO), chronic diseases were responsible for 38 million (68%) of the world’s 56 million deaths in 2012.[Citation1] These diseases may develop from prolonged cellular redox imbalances in the body. Metabolic reactions in the living cells can produce reactive oxygen species and reactive nitrogen species (ROS/RNS), which when present in excessive amounts can oxidize and cause damage to various biological molecules such as lipid, DNA and proteins.[Citation2,Citation3] This particularly happens during oxidative stress, arising from the disruption of cellular redox signalling and control, which causes increase in the pro-oxidant:antioxidant ratio of the body.[Citation4,Citation5]
Among the chronic diseases, cancer, which caused 21.7% of the deaths worldwide in 2012, is of global challenge. Severe oxidative stress condition is a known factor that can contribute to the development of cancer.[Citation1] The presence of excessive ROS/RNS can cause DNA oxidation, leading to chromosomal defects, oncogene activation, abnormal gene transcription alteration, and ultimately malignant transformation.[Citation6,Citation7] Cancers are generally treated by radiation therapy or radical surgery, which is often combined with adjuvant chemotherapy. However, these treatments present toxic side effects and could also exacerbate the disease condition by inducing an increase in the ROS/RNS levels, leading to poor antioxidant status in the body. This can further lead to severe oxidative stress, which could promote apoptosis resistance in cancer cells.[Citation8]
The uses of medicinal plants in complementary and alternative medicine have gained considerable attention lately, particularly for the treatment of chronic diseases. Plant-sourced foods are of particular significance, as they are rich in antioxidant molecules that could help prevent oxidative stress or redox imbalances and reduce the risk of developing chronic diseases.[Citation9,Citation10] Antioxidants are defined as “any substance that can prevent, reduce, or repair the ROS/RNS-induced damage to a target biomolecule”.[Citation11] Dietary plant antioxidants have the potential to exert anticancer effects through several mechanisms, which include their ability to interfere with the various stages of carcinogenesis.[Citation9]
Polygonum minus Huds. (syn. Persicaria minor [Huds.] Opiz) is a tropical herb from the family Polygonaceae, locally known as “Kesum”, “Cenohom”, or “Daun Laksa” in Malaysia.[Citation12,Citation13] It is frequently used as vegetable salad and as flavouring enhancer in many dishes. Traditionally, the leaves have been used to treat skin fungal infection, indigestion, dandruff, postnatal tonic, sprains, and alleviating body aches.[Citation12–Citation14] The fragrant nature of P. minus is due to its high contents (72.54%) of volatile aromatic oils, with at least 48 different essential oils identified.[Citation15] Approximately 77 metabolites have been identified in P. minus by Gas Chromatography Mass Spectrometry (GC-MS) and these were mainly sesquiterpenes and aliphatic aldehyde compounds.[Citation16] The major essential oils were decanal (16.26%), 1-decanol (12.68%), and dodecanal (43.47%).[Citation15–Citation17] The variation of dodecanal content among four different accessions of P. minus was reported to be about 10% (37.83–48.28%).[Citation18] The phytochemicals identified from P. minus include myricetin, quercetin, flavone, and methyl flavonol.[Citation19] P. minus methanolic extract was non-toxic to Sprague Dawley rats at a dosage of 2 g/kg[Citation20] and only caused moderate DNA strand breaks (16–31.4%) in lymphocytes at 250–2000 µg/mL.[Citation21] The n-hexane extract of P. minus leaf showed antimicrobial activity against methicillin-resistant Staphylococcus aureus.[Citation16] The water and methanol extracts of P. minus leaf showed antioxidant and acetylcholinesterase enzyme inhibition activities.[Citation16,Citation22] The ethanolic extract of P. minus demonstrated the complete inhibition of 5-LOX and COX-1 activities at 100 µg/mL. The aqueous extract also demonstrated anti-inflammatory activity in a rat animal model through the inhibition of carangeenan-induced paw oedema.[Citation23] The ethyl acetate (EA) fraction of P. minus was cytotoxic and capable of inducing apoptosis and S-phase cell-cycle arrest in HepG2 cells.[Citation24] P. minus extracts have also been reported to show the following activities: antioxidant,[Citation25,Citation26] anti-HSV1, anti-vesicular stomatitis virus,[Citation27] anti-fungal, anti-ulcer,[Citation28] anti-hyperlipidemic,[Citation29] anti-acetylcholinesterase,[Citation30] antimicrobial, and cytotoxicity towards HeLa cells.[Citation27,Citation31] Other members of Polygonaceae have also been reported to show antimicrobial, antioxidant, anticancer, cytotoxic, anti-angiogenic, and anticholinesterase activities.[Citation32–Citation34] The ethno-medicinal uses of P. minus and published data on the bioactivities of Polygonaceae family members provide justifications for the undertaking of the present study, to further characterise the antioxidant and anti-proliferative activities of P. minus. This may provide scientific insights of its benefits and justification for its ethno-medicinal uses.
Materials and methods
Reagents, solvents, and chemicals
The main reagents and chemicals used in this study were of analytical grade and mostly purchased from Sigma-Aldrich. Solvents used for the extraction of plant materials were obtained from Fisher Scientific and Merck Millipore. The following are some of the reagents used: gallic acid (Sigma, G7384); quercetin (Sigma, Q4951); trolox (Merck, 648471); DMSO (Sigma, D4540); DPPH reagent (Sigma, D9132); Ferrozine (Sigma, 82950); MTT reagent (Thermofisher Scientific, M6494); 2,4,6-Tri(2-pyridyl)-1,3,5-triazine (TPTZ) (Sigma,T1253); Folin–Ciocalteu’s phenol reagent (Merck, Cat.# 1.09001); ABTS (Merck, 194430); and p-nitroblue tetrazolium chloride (NBT) (Merck, 484235).
Samples preparation
Fresh and healthy P. minus leaves were purchased from the local wet market and processed on the same day. The identity of the sample was confirmed by Dr Sugumaran Manickam of Rimba Ilmu, Institute of Biological Sciences, University of Malaya. The leaves were cleaned with water, sliced into smaller pieces, and dried in a 40 °C oven until no weight reduction was observed. The dried leaves were powdered using a table blender and stored at –20°C until further analysis.
P. minus extracts preparation
The dried leaves were extracted sequentially, using the following solvents in the order indicated: HX > EA > methanol > water.[Citation35] Dried leaves of 30 g weight were extracted using 300 mL of HX in an incubator shaker at 37°C for 8 h and centrifuged at 200G for 5 min. The supernatant was filtered through Whatman filter paper (No. 4). The extraction was repeated twice, and the residues were dried. The 900 mL of HX solvent that was collected after the extraction was evaporated at 40°C using a rotary evaporator (BUCHI Rotavapor R-215). The extraction was continued using the same method for each of the remaining solvents (EA, methanol, and water) following the method as indicated above. The filtrate of the water extract was dried using a freeze-dryer. The plant extracts were dissolved in 10% dimethyl sulfoxide (DMSO) at 2 mg/mL and kept at –20°C until use.
Antioxidant potential assays
Total phenolic content
Total phenolic content (TPC) was determined using Folin–Ciocalteu reagent (FCR).[Citation36] A total of 50 μL of aqueous FCR (10% v/v) was mixed with 100 μL of the plant extract and incubated for 5 min. Next, 350 μL of 1M Na2CO3 was added to the mixture and incubated at room temperature for 2 h, followed by absorbance measurement at 765 nm, using a polystyrene cuvette (BRAND®, Sigma-Aldrich, product #Z330418) and a VARIAN Cary®50 UV-VIS spectrophotometer. A standard calibration curve was constructed using gallic acid, using concentrations up to 0.2 mg/mL. Results were expressed as mg gallic acid equivalents (GAE) per gram of dried extract (DE).
Total flavonoid content
Total flavonoid content (TFC) was determined according to Chang et al.[Citation37] with minor modifications. Aluminium trichloride (10% w/v) and potassium acetate (1M), both at 5 μL volumes, were mixed with 25 μL of plant extract in a 96-well microplate. A total of 75 μL of ethanol (95% v/v) and 140 μL of distilled water were added to the mixture successively and mixed. The mixture was incubated for 30 min at room temperature followed by absorbance reading at 415 nm using Tecan Infinite® M1000 Pro Multimode reader (Tecan Trading AG, Switzerland) (Tecan-I). Quercetin was used as a standard and flavonoid values were expressed as milligram quercetin per gram of DE.
Ferric reducing antioxidant power
Ferric reducing antioxidant power (FRAP) was determined according to Benzie and Strain[Citation38] with minor modifications. FRAP reagent was freshly made by mixing the following solutions in a 10:1:1 volume ratio (v/v/v): acetate buffer (300 mM, pH 3.6), 10 mM TPTZ in 40 mM HCl, and FeCl3.6H2O (20 mM). Ten microliters of the plant extract was mixed with 300 μL of FRAP reagent in a 96-well microplate and absorbance reading at 593 nm was taken 30 min thereafter using a Tecan-I microplate reader. A calibration curve was plotted using FeSO4 solution (0–1 mM). The results were expressed as μmol FeCitation2+ per gram of DE. Quercetin, gallic acid, ascorbic acid, and trolox were used as positive controls.
ABTS●+ radical-scavenging activity
ABTS●+ (2,2′-azinobis-3-ethylbenzothiazoline-6-sulphonic acid) radical-scavenging activity was determined according to Re et al.[Citation39] with minor modifications. ABTS●+ radical cations were made by mixing 7 mM ABTS solution with 2.45 mM potassium persulfate and left in the dark for 12–16 h at room temperature. The resulting solution absorbance was measured at 734 nm and adjusted to 0.7 using ethanol. Around 20 μL of plant extract was mixed with 180 μL of ABTS●+ reagent in a 96-well microplate, and the absorbance at 734 nm was taken after 30 min using a Tecan-I microplate reader. Quercetin, gallic acid, ascorbic acid, and trolox were used as positive controls. The TEAC values were calculated for the extracts using the trolox calibration curve (0–1.6 mM). Results were expressed as μmol trolox equivalents (TE)/g DE.
DPPH• radical-scavenging activity
The 1,1-diphenyl-2-picryl hydrazyl (DPPH) free radical-scavenging activity was determined according to Brand-Williams et al.[Citation40] with minor modifications. One hundred micromolar DPPH solution was prepared in methanol and left in the dark for at least 30 min. Next, 20 μL of plant extracts, at different concentrations (up to 1000 μg/mL), was mixed with 150 μL of 100 μM DPPH solution in a 96-well microplate. After 30 min, the absorbance of the reaction mixture was taken at 515 nm using a Tecan-I microplate reader. Quercetin, gallic acid, ascorbic acid, and trolox at concentrations up to 1000 μg/mL were used as positive controls. A calibration curve was constructed using trolox at concentrations up to 400 mM. DPPH radical-scavenging activity of the extracts was expressed as μmol TE per gram of DE. The DPPH radical-scavenging activity was calculated using the formula [(A0—Ax) /A0] x 100%, where Ax and A0 are the absorbances in the presence and absence of test compounds, respectively. When significant scavenging activity was detected, the EC50 value was also estimated.
Superoxide anion radical (O2•‒)-scavenging activity
Superoxide anion radical-scavenging activity was evaluated according to Robak and Gryglewski[Citation41] with slight modifications. Reagents consisted of 150 μM NBT, 468 μM nicotinamide adenine dinucleotide (NADH), and 60 μM phenazine methosulphate (PMS) in 0.1M phosphate buffered saline. Around 50 μL of extract, at different concentrations (0–1000 μg/mL), was mixed with 50 μL of NBT, 50 μL of NADH, and 50 μL of PMS in a 96-well microplate. After incubation in the dark for 10 min at room temperature, the absorbance was read at 570 nm using a Tecan-I microplate reader. Gallic acid was used as a positive control. The results are expressed as percentage inhibition of O2• ‒ radicals. EC50 was estimated when significant O2• ‒ scavenging activity was observed.
Nitric oxide (●NO) radical-scavenging activity
Nitric oxide scavenging activity was measured according to Awah and Verla[Citation42] with minor modifications. This assay uses sodium nitroprusside (SNP) to generate ●NO. Griess reagent was used to measure nitrite (NO2−) that was formed from the oxidation of ●NO. Now, 25 μL of the extract, at different concentrations (0–1000 μg/mL), was mixed with 25 μL of freshly prepared 5 mM SNP solution in phosphate buffered saline (pH 7.3) in a 96-well microplate. The mixture was then incubated for 60 min under a visible polychromatic light (150 W). Griess reagent (50 μL), containing equal volumes of 1% sulfanilamide in 5% phosphoric acid (H3PO4) and 0.1% of naphthylethylenediamine dihydrochloride, was added to the mixture and incubated for 5 min before absorbance was read at 550 nm using Tecan-I microplate reader. Gallic acid was used as a positive control. Results were expressed as a percentage of NO radical-scavenging activity. EC50 was estimated when a significant scavenging activity was observed.
Ferrous (Fe2+) ion-chelating ability
The ferrous (Fe2+) ion-chelating FIC ability was determined according to the method of Decker and Welch[Citation43] with minor modifications. For each determination, the following solutions were added and mixed sequentially in a 96-well microplate: 50 μL of each of the extracts (0–2000 μg/mL), 20 μL of 0.5 mM FeCl2, 160 μL of distilled water, and 20 μL of 2.5 mM ferrozine. The mixture was allowed to stand for 10 min at room temperature. The ferrous iron–ferrozine complex formation was then monitored by taking the absorbance at 562 nm against a blank reaction mixture, which substituted the tested extract with 50 μL of distilled water. Ethylenediaminetetraacetic acid (EDTA) was used as a positive control. The EC50 value is the concentration (mg/mL) at which the chelating activity of the tested compound was at 50%.
Cellular antioxidant activity assay
The cellular antioxidant activity (CAA) assay was carried out according to Wolfe and Liu[Citation44] with minor modifications. American Type Culture Collection (ATCC) human colon cancer cells HCT116 were seeded at a density of 5 x 104 cells/well on a 96-well black microplate in 100 µL of growth medium. The cells were then incubated for 24 h, after which the growth medium was removed and the wells were washed with Phosphate Buffered Saline (PBS). Triplicate wells were treated with 100 µL of various concentrations of P. minus methanol extract (0–500 µg/mL) containing 25 µM DCFH-DA dissolved in the treatment medium. After 1 h of incubation, the media was removed and the cells were once again washed with 100 µL of PBS. Then, 600 µM of ABAP was applied to the cells in 100 µL of Hank's Balanced Salt Solution (HBSS) and the fluorescence intensity was measured with the Tecan-I microplate reader. Emission at 538 nm was measured with excitation at 485 nm every 5 min for 1 h. Each plate included a triplicate control and blank. The control wells consisted of cells treated with DCFH-DA and 2,2′-azobis (2-amidinopropane) dihydrochloride (ABAP), while blank wells contained cells treated with the dye and HBSS only. After blank subtraction from the fluorescence readings, the area under the curve of fluorescence versus time was integrated to calculate the CAA value according to the following formula:
where ʃ SA is the integrated area under the sample fluorescence versus time curve and ʃ CA is the integrated area from the control curve. The median effective dose (EC50) was determined from the median effect plot of log (ƒa/ƒu) versus log (dose), where ƒa is the fraction affected and ƒu is the fraction unaffected by the treatment.
Mass spectrometry analyses: Liquid chromatography ion-trap time-of-flight mass spectrometry (LCMS-IT-TOF)
Two mg of the DE was dissolved in 1 mL of HPLC-grade methanol and filtered using a 0.25 μm nylon filter membrane. The extract was analysed on a Shimadzu liquid chromatography ion-trap time-of-flight
(LCMS-IT-TOF) system, equipped with a Photodiode Array (PDA) UV detector (220, 254, and 350 nm) and an ion trap TOF mass spectrometer. The analysis was performed in positive and negative ion modes, using the Waters XBridge BEH C18 column (PN 186003085, 2.1 × 50 mm, 2.5 μm) with the following parameters: solvent A: 0.1% formic acid; solvent B: acetonitrile with 0.1% formic acid; flow rate: 0.25 mL/min; column temperature: 40 °C; injection volume: 25 µL; gradient: 0–100% over 14 min. The compounds were identified by comparison of the MS data to those reported in the literature.
Anti-proliferative activity assay
The anti-proliferative activity of the extracts was evaluated through cell viability (MTT) (3,(4,5-dimethythiazol-2-yl)-2,3-diphenyl tetrazolium bromide) assay, according to Twentyman and Luscombe[Citation45] with minor modifications. HCT116 (passage #30) and CCD841 (passage #10) cell lines were cultured and maintained according to the protocol specified by ATCC. The cells (~5.0 × 103) were incubated overnight in a 100 μL culture media/96-well plate and thereafter treated with 20 μL of plant extracts at different concentrations. After 48 h of incubation, 20 μL of MTT solution was added into each well and incubated for another 4 h. The culture solution was then discarded and 150 μL DMSO was added to solubilize the formazan crystals. Absorbance was then taken at 595 nm using a Tecan-I microplate reader. Each determination was performed in six replicates. When significant anti-proliferative activity was observed, the IC50 value was determined.
Statistical analyses
Unless otherwise specified, each determination was performed in triplicate. Results were presented as mean ± standard error of mean (SEM) and analysed using SPSS statistical software (version 20.0, SPSS Inc.). One-way analysis of variance (ANOVA) and Tukey test were used to compare means among groups (three and more groups). Independent t-test was calculated to compare the mean between two groups. Pearson correlation was used to investigate the correlation between antioxidant components and the antioxidant activities. An observation is considered statistically significant if the p value was less than 0.05 (p < 0.05).
Results and discussion
Extraction yield
The types of solvents used for the extraction of phytochemicals from herbs are known to affect the yield and bioactivity of the resulting extract.[Citation35,Citation46] The use of solvents with varying polarities can provide a better mass transfer medium, with a wider coverage of extraction efficiency. The present study is the first to report the sequential extraction of P. minus leaves using HX, EA, methanol (MeOH) and water (W), to characterize its anti-proliferative and antioxidant activities.
Extraction yields obtained for the various solvents are shown in . PM-MeOH extract demonstrated the most significant yield of 15.41%, while PM-W showed the second highest yield, which was 7.09%. When combined, the yield using polar solvents (i.e. MeOH and W) was 22.05%, while the yield from nonpolar solvents (i.e. HX and EA) was only 3.41%, suggesting that P. minus leaf extracts had more polar components. The distinctive structures and functional groups of plant phytochemicals are known to affect their polarity and solubility in the different extraction solvents used, and thus the yields would be dependent on such characteristics. Methanol is frequently reported to generate the highest yields when used as an extracting solvent.[Citation35]
Table 1. Extraction yields and antioxidant components of P. minus leaf extracts.
Total phenolic content
Phenolics are a major group of phytochemicals responsible for the nutritional and antioxidants activities in plants. They are able to become electron/hydrogen donor, reducing agent or metal chelator, which underlies the health benefits associated with the dietary consumption of plants. Thus, profiling of phenolic content is frequently carried out to justify the potential health benefits and bioactivity of plant-sourced foods.[Citation47,Citation48] In the present study, the range of TPC values obtained among the extracts was from 2.45 ± 0.05 to 174.00 ± 0.18 mg GAE/g DE (). The TPC value for PM-MeOH in the present study was apparently higher than that reported by Huang et al.,[Citation49] which used freeze dried leaf/stem or whole plant materials from four different Polygonum species. The TPC values for PM-MeOH and PM-W extracts in the present study were also higher compared to those previously reported: 55.1 ± 0.9 mg GAE/g (water extract)[Citation21] and 12.9 ± 2.8 mg GAE/g extract (acetone extract),[Citation50] although they were lower compared with the ethanol extract (207 ± 0.011 mg GAE/g extract) that was reported by Qader et al.[Citation25]
Total flavonoid content
Flavonoids are phenolic compounds with diverse beneficial health-promoting activities, including reducing the risk of dementia, preventing LDL-oxidation and atherosclerosis, lowering cholesterol, and antioxidant and antihypertensive activities. The hydroxyl groups of flavonoids contribute to the radical-scavenging ability.[Citation9] In the present study, flavonoids were detected in all the extracts tested (), and their values ranged from 10.18 ± 0.20 to 53.19 ± 0.71 mg QE/g DE. PM-MeOH again showed the highest TFC value (53.19 ± 0.71 mg QE/g extract), followed by EA, W, and HX extracts, in descending order.
FRAP assay
This assay uses ferric tripyridyltriazine (FeCitation3+–TPTZ) complex that reacts with reducing agents or antioxidant molecules to form an intense blue FeCitation2+–TPTZ complex with a λmax at 593 nm, and the colour intensity generated is proportional to the amount of antioxidant molecules present in the reaction mixture. In the present study, the FRAP values of all the extracts ranged from 26.11 ± 0.28 to 1728.33 ± 0.96 µmol FeCitation2+/g extract (). PM-MeOH showed the highest FRAP value (1728.33 ± 0.96 µmol FeCitation2+/g extract), followed by (in descending order) PM-W, PM-EA, and PM-HX. Borneo et al.[Citation51] categorized the strength of ferric-reducing capacities of antioxidants into four groups: low antioxidant power (<100 μmol FeCitation2+/g), medium (100–250 μmol FeCitation2+/g), high (250–625 μmol FeCitation2+/g), and extremely high (>625 μmol FeCitation2+/g). Based on such a classification, PM-MeOH and PM-W are considered to have extremely high antioxidant power. The respective FRAP values for PM-MeOH and PM-W extracts were about two and thirteen times higher than the values that were previously reported: 781.32 ± 4.2 µmol FeCitation2+/g MeOH extract[Citation24] and 68.3 ± 3.3 µmol FeCitation2+/g water extract.[Citation21]
Table 2. In vitro antioxidant activity assays of P. minus leaf extracts.
ABTS●+ radical-scavenging activity
ABTS●+ reagent is a stable radical monocation that is directly produced from the oxidation of ABTS by potassium persulfate.[Citation39] The ABTS●+ blue/green chromophore decolorizes when it is scavenged by antioxidant molecules and this can be monitored at 734 nm. The decrease in ABTS●+ absorbance in the presence of the tested extract reflects the percentage of ABTS●+ inhibition. The ABTS●+ scavenging activity of P. minus has never been reported in the literature. The scavenging activities of PM leaf extracts are shown in . PM-MeOH and PM-W extracts showed very prominent ABTS●+ scavenging activity with IC50 values of 159.62 ± 14.51 and 198.36 ± 15.27 µg/mL, respectively. When the ABTS●+ scavenging was expressed as TEAC values (), they ranged from 25.43 ± 1.02 to 226.25 ± 4.25 µmol TE/g extract, with PM-MeOH and PM-W extracts showing the most prominent TEAC values.
Figure 1. In vitro antioxidant activities of P. minus extracts. (A) ABTS●+radical-scavenging. (B) DPPH radical-scavenging. (C) Superoxide anion (O2•-) radical-scavenging. (D) Nitric oxide (●NO) radical-scavenging. (E) Ferrous ion-chelating activity. The positive controls were: quercetin (Qn), gallic acid (GA) and ascorbic acid (AA). Values are means ± SD (n = 3). PM, P. minus; HX, hexane; EA, ethyl acetate; MeOH, methanol, W, water.
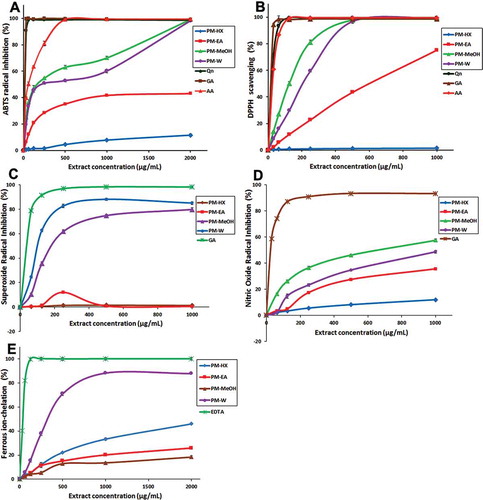
DPPH• radical-scavenging activity
DPPH•-scavenging activity is frequently used to evaluate the in vitro antioxidant activity of plant extracts. The scavenging of DPPH• by antioxidants causes the bleaching of the purple-coloured solution. This assay is compatible for the evaluation of hydrophilic and lipophilic antioxidants activities.[Citation30] The DPPH•-scavenging activity of the extracts is shown in . With the exception of the HX extract, all other extracts showed concentration-dependent DPPH•-scavenging activity. PM-MeOH and PM-W scavenging activities appeared to increase rapidly and reached their maximum values at ~500 μg/mL. The IC50 values for PM-MeOH and PM-W extracts were 125.51 ± 4.70 and 210.16 ± 1.35 μg/mL, respectively, and these values imply a higher scavenging activity compared with that reported by Ghazali et al.,[Citation24] which were 540.0 ± 10.8 μg/mL for PM-MeOH and 472.5 ± 15.2 μg/mL for PM-W.
Superoxide (O2•‒) anion-radical scavenging activity
Superoxide anions can be produced in the human body through normal cellular metabolism. However, excessive amounts can cause cellular damage.[Citation11] The O2•‒ scavenging activity was assayed using the PMS-NADH-NBT system, where O2•‒ was generated by the oxidation of NADH in the PMS/NADH coupling reaction, followed by the reduction of NBT. The presence of antioxidants that can scavenge O2•‒ is indicated by the decrease in absorbance at 570 nm. The O2•‒ scavenging activity for PM leaf extracts is shown in Fig. 1C. The present study is the first to report the O2•‒ scavenging activity of P. minus. PM-W and PM-MeOH extracts showed concentration-dependent inhibition of O2•‒. In this case, PM-W showed the strongest activity, reaching 90% O2•‒ inhibition at ~500 μg/mL, followed by PM-MeOH, reaching 80% O2•‒ inhibition at ~1000 μg/mL. The IC50 values for PM-W and PM-MeOH were 103.77 ± 3.86 and 195.64 ± 10.57 µg/mL, respectively ().
Nitric Oxide (●NO) Radical-scavenging Activity
Nitric oxide is an important signalling molecule in various biological functions, which include vasodilation, neurotransmission, regulating hormone secretion, destruction of pathogenic microbes and killing of tumour cells.[Citation3] However, ●NO can also pose undesired biological effects. It is pro-oxidant at high concentrations and could generate extremely reactive peroxynitrites, which damages biomolecules.[Citation3] Thus, the evaluation of ●NO-scavenging activity of plant extracts is frequently carried out to evaluate their antioxidant potential. The present study is the first to assess the •NO scavenging activity of P. minus, which is shown in Fig. 1D. Only two of the extracts showed robust ●NO-scavenging activity and they were PM-MeOH and PM-W, with IC50 values of 675 ± 32.33 and 1239 ± 42.15 µg/mL, respectively. The IC50 value for gallic acid was 49.45 ± 0.95 µg/mL. PM-MeOH and PM-W extracts were earlier observed to have among the highest TPC values (). Substantial amounts of tannins (natural plant polyphenols), the active components underlying the ●NO-scavenging activity in traditional crude drugs,[Citation52] have been previously reported in the MeOH extracts of Polygonum species.[Citation49] The presence of galloyl groups in polyphenols, especially tannins, plays an important role in ●NO-scavenging activities.[Citation53] This may provide an explanation on the prominent ●NO-scavenging activity of PM-MeOH extract that was observed in the present study.
FIC activity
Ferrous ions (FeCitation2+), which are involved in processes such as enzyme activity and oxygen transportation, are essential in biological systems. However, FeCitation2+ produces highly reactive hydroxyl radicals when reacting with hydrogen peroxide, and such reactive species can induce oxidative damage.[Citation11] Thus, the evaluation of the metal-chelating ability of antioxidant candidates is of significance to gauge their antioxidant potential. In this assay, the ferrozine reagent reacted with FeCitation2+ to form a stable FeCitation2+–ferrozine complex. The magenta-coloured complex can be disrupted in the presence of antioxidants that can act as a metal chelator. The bleaching of FeCitation2+–ferrozine complex can be spectrophotometrically monitored at 562 nm to deduce the coexisting metal chelator(s).[Citation43] In the present study, all extracts of P. minus showed concentration-dependent metal chelating activity, although their FIC activities differed (). PM-W extract showed robust FIC activity with an EC50 value of 341.54 ± 11.15 µg/mL extract () and reached > 70% chelation activity at less than 500 µg/mL. The EC50 of PM-W extract was recently reported to be 5400 µg/mL,[Citation26] which was ~15 times higher compared with the value obtained in the present study, suggesting that the sequential extraction employed in this study produced PM-W extract with a much better FIC activity.
Correlation analysis
When analysed together, TPC and TFC values of the extracts imply strong (r > 0.8) and positive correlations (r = 0.60–0.79) with FRAP, ABTS, DPPH, and ●NO-scavenging antioxidant activities (). This is suggestive of the effects of polyphenol and flavonoid constituents to the observed antioxidant activities of the PM leaf extracts. The polyphenol content showed a strong positive correlation with O2•‒ radical-scavenging activity, but negative correlation (r = −0.2 to −0.39) with FIC activity. Flavonoids demonstrated moderately positive correlation (r = 0.4–0.59) with O2•‒ radical-scavenging activity, as well as negative correlation with FIC activity.
Table 3. Pearson correlation analyses of antioxidant components and antioxidant activities.
Cellular Antioxidant Activity
The present study is the first report that measures the CAA activity of P. minus. CAA assay provides a complementary approach in assessing the antioxidant activity of compounds, since it takes into account biological factors in living systems such as membrane permeability, cellular uptake, metabolism, and antioxidant distribution.[Citation44] In the present assay, the ability of the PM-MeOH extract (and quercetin, as a positive control) to inhibit the oxidation of non-fluorescent 2ʹ,7ʹ-dichlorodihydrofluorescein (DCFH2) to highly fluorescent 2ʹ,7ʹ-dichlorofluorescein (DCF) inside HCT116 cells was measured, while ABAP acted as a peroxyl radical generator.
The results indicated that PM-MeOH extract and quercetin were able to inhibit the oxidation of DCFH2 in a dose-dependent manner ( and ). This suggests that the bioactive antioxidant(s) in the PM-MeOH extract was well absorbed into the HCT116 cells and exhibited antioxidant activity. The dose–response curves are shown in Figs. 2C and 2D, while the median effect plots are shown in and . The values of EC50, which is the concentration at which ƒa/ƒu = 1 as calculated from the linear regression of the median effect plot,[Citation44] for PM-MeOH and quercetin were 263.92 ± 21.60 µg/mL and 93.76 ± 5.28 µg/mL, respectively. Although the EC50 value of PM-MeOH extract was higher than that of quercetin, it was significantly lower than the values reported for extracts from common fruits (EC50 > 2 mg/mL) and vegetables (EC50 > 19 mg/mL).[Citation44,Citation54] As for quercetin, its EC50 value (310 ± 17.32 µM) in HCT116 cells was higher than HepG2 cells (EC50, 5.09 ± 0.19 µM),[Citation44] suggesting that the antioxidant activity of quercetin inside HCT116 cells was less prominent.
Figure 2. Cellular antioxidant activity of PM-MeOH extract. Inhibition of peroxyl radical-induced oxidation of DCFH2 to DCF in HCT116 cells by (A) PM-MeOH and (B) Quercetin. Dose–response curve for the inhibition of peroxyl radical-induced DCFH2 oxidation by (C) PM-MeOH and (D) Quercetin. Median effect plots for the inhibition of peroxyl radical-induced DCFH2 oxidation by (E) PM-MeOH and (F) Quercetin. The values shown are from a single experiment (mean ± SEM, n = 3).
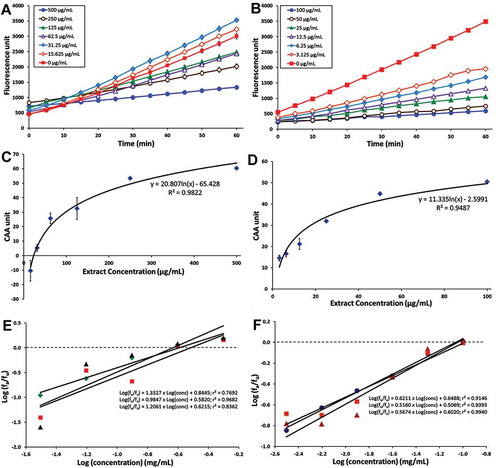
Liquid chromatography mass spectrometry analysis
When PM-MeOH extract was subjected to LCMS-IT-TOF analysis, distinctive m/z values were detected ( and ), with eight of the components deduced () based on published mass spectrometry data for five Polygonum species, including Persicaria hydropiper.[Citation30,Citation49] The compounds identified were tannins and the flavonoids, including apigetrin, hyperoside, isoquercetin, astragalin, miquelianin, quercetin, and quercitrin. These compounds are known to exhibit antioxidant and free radical-scavenging activities[Citation49,Citation52,Citation53] and thus may, in part, explain the prominent antioxidant activity of PM-MeOH extract. Flavonoids have been reported to be important bioactive constituents of Polygonum species, which includes quercetin-3-O-rhamnoside (quercitrin).[Citation49] Quercetin and quercetin-3-O-rhamnoside in the EA extract of Persicaria hydropiper have been previously reported to show significant antioxidant activity, with IC50 values of less than 20 µg/mL in the DPPH-scavenging assay.[Citation30]
Table 4. Liquid chromatography and mass spectrometry analyses (LCMS-IT-TOF) of PM-MeOH extract.
Anti-proliferative activity of P. minus leaf extracts
In the present study, the effect of P. minus leaf extracts on the viability of normal colon (CCD841) and colon cancer (HCT116) cell lines was evaluated through MTT assay. Two of the extracts (i.e. PM-MeOH and PM-W) appeared to show a dose-dependent stimulation of CCD841 cells proliferation (). At 333 µg/mL, PM-MeOH and PM-W stimulated the proliferation of CCD841 cells, increasing the cell viability percentage to 139% and 160%, respectively. When the experiment was repeated using HCT116 cells, the PM-W extract was again observed to stimulate cell proliferation, increasing the percentage of HCT116 cell viability to 134% and 157%, at 167 µg/mL and 333 µg/mL, respectively. These observations suggest that PM-MeOH contains compound(s) that could preferentially encourage the healing of normal colon cells, without stimulating the proliferation of HCT116 colon cancer cells. In contrast, PM-W extract contains compound(s) that could promote the progression of colon cancer.
Figure 4. MTT anti-proliferative assay of P. minus extracts on HCT116 and CCD841 cell lines. The numbers beside the legends indicate concentration of P. minus extracts in µg/mL. The percentage of cell viability is the ratio of cell viability for the treated over untreated cells x 100%. Values are mean ± SD (n = 6) . The PM-EA extract had lower solubility in DMSO, thus lower range of concentrations (26.86, 53.72 and 107.44 µg/mL) were used. The values with different letters (a-c) are significantly different, p < 0.05 (Tukey’s test).
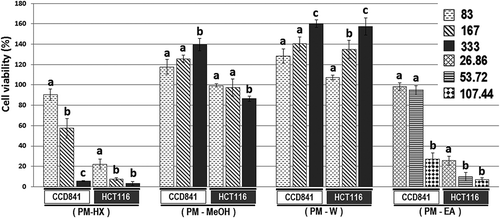
In the same experiment, PM-HX and PM-EA extracts also appeared to show prominent anti-proliferative activity towards HCT116 cells, causing more than 50% reduction in the cell viability percentage (). The anti-proliferative activity of these extracts on HCT116 was investigated further by determining their IC50 values, since these extracts were more cytotoxic towards HCT116 compared with CCD841. PM-HX and PM-EA were able to inhibit HCT116 cells proliferation in a dose-dependent manner (). The IC50 values for these two extracts on CCD841 were markedly higher than HCT116, indicating their selective cytotoxicity towards colon cancer cells (). A “Selectivity Index” (SX) was then calculated based on the following formula: SX = IC50 CCD841/IC50 HCT116 x 100.[Citation55] An SX value of >100 implies that the cytotoxic effect is more selective on the cancer cells. Both PM-HX and PM-EA extracts showed SX value >100, implying selective toxicity towards colon cancer cells.
Table 5. Anti-proliferative activity of PM-HX and PM-EA extracts on HCT116 and CCD841 cell lines.
Figure 5. Cell viability assay. Dose–response curves for PM-HX and PM-EA extracts on HCT116 cells (48 h treatment).
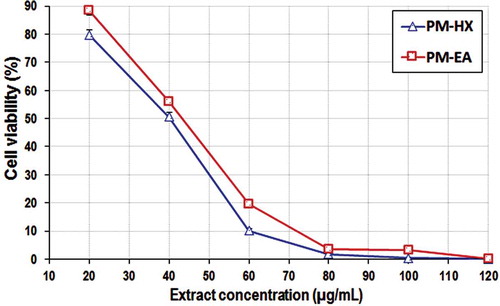
A previous study has reported that the water and ethanol extracts of P. minus did not show any cytotoxicity towards normal lung cell line (Hs888Lu) at 500 µg/mL.[Citation25] Ghazali et al.[Citation24] reported that P. minus exhibited anti-proliferative activity on the following cell lines, with the corresponding IC50 values: HepG2 (EA extract, 32.25 ± 3.72 µg/mL); HeLa (EA extract, 63.09 ± 6.70 µg/mL); WRL-68 (petroleum ether extract, 56.23 ± 3.2 µg/mL); and HCT116 (MeOH extract, 86.3 ± 3.5 µg/mL; EA extract, 199.52 ± 5.2 µg/mL). In the present study, the IC50 of PM-EA extract on HCT116 cells was significantly lower compared to that reported by Ghazali et al.,[Citation24] suggesting that the sequential extraction employed in this study was able to produce PM-EA extract with a higher anti-proliferative activity.
In an attempt to elucidate the cytotoxicity mechanism induced by PM-HX and PM-EA extracts, further analysis was performed using ApoTox-Glo™ Triplex Assay, Muse® MultiCaspase, Muse® Annexin V, and Dead Cell Assay Kits. However, the results suggested that the cytotoxic effect of the extracts on HCT116 cells was not caspase-dependent (data not shown). Hence, the extracts’ anti-proliferative activity might have been achieved through other programmed cell death mechanisms such as necroptosis or paraptosis.[Citation56]
Conclusion
In the present study, PM-MeOH extract was generally observed to have the highest antioxidant potential, which is most likely attributed to its relatively higher phenolic and flavonoid contents. LCMS-IT-TOF analyses of the PM-MeOH extract suggested the presence of tannins, apigetrin, hyperoside, isoquercetin, astragalin, miquelianin, quercetin, and quercitrin. Results from the CAA assay indicated that the PM-MeOH extract was well absorbed into HCT116 cells and it was able to provide protection against oxidative damage at the cellular level, with an EC50 value of 263.92 ± 21.60 µg/mL. PM-HX and PM-EA extracts showed selective cytotoxicity towards HCT116 with IC50 values of 40.00 ± 0.83 µg/mL and 43.18 ± 0.67 µg/mL, respectively. The present study suggests that P. minus is a promising source of bioactive phytochemicals that may be useful in cancer therapeutics and nutraceutical industry. Further research is warranted to isolate the anticancer and antioxidant components, to understand their health-promoting mechanisms of action.
LJFP_A_1315591_Supplementary_File.docx
Download MS Word (91.7 KB)Funding
This study was supported by the University of Malaya research grants RG469-12HTM, PG086-2012B, and FRGS grant FP016/2013B.
Supplemental data
Supplemental data for this article can be accessed on the publisher’s website.
Additional information
Funding
References
- Mendis, S.; Davis, S.; Norrving, B. Organizational Update the World Health Organization Global Status Report on Noncommunicable Diseases 2014; One More Landmark Step in the Combat against Stroke and Vascular Disease. Stroke 2015, 46(5), E121–E122.
- Lopez-Alarcon, C.; Denicola, A. Evaluating the Antioxidant Capacity of Natural Products: A Review on Chemical and Cellular-based Assays. Analytica Chimica Acta 2013, 763, 1–10.
- Hummel, S.G.; Fischer, A.J.; Martin, S.M.; Schafer, F.Q.; Buettner, G.R. Nitric Oxide as A Cellular Antioxidant: A Little Goes A Long Way. Free Radical Biology and Medicine 2006, 40(3), 501–506.
- Jones, D.P. Radical-free Biology of Oxidative Stress. American Journal of Physiology. Cell Physiology 2008, 295(4), C849–68.
- Pham-Huy, L.A.; He, H.; Pham-Huy, C. Free Radicals, Antioxidants in Disease and Health. International Journal of Biomedical Science 2008, 4(2), 89–96.
- Lobo, V.; Patil, A.; Phatak, A.; Chandra, N. Free Radicals, Antioxidants and Functional Foods: Impact on Human Health. Pharmacognosy Reviews 2010, 4(8), 118–126.
- Willcox, J.K.; Ash, S.L.; Catignani, G.L. Antioxidants and Prevention of Chronic Disease. Critical Reviews in Food Science and Nutrition 2004, 44(4), 275–295.
- Di Maso, V.; Mediavilla, M.G.; Vascotto, C.; Lupo, F.; Baccarani, U.; Avellini, C.; Tell, G.; Tiribelli, C.; Crocè, L.S. Transcriptional Up-regulation of APE1/Ref-1 in Hepatic Tumor: Role in Hepatocytes Resistance to Oxidative Stress and Apoptosis. PLoS One 2015, 10(12), e0143289.
- Abbas, M.; Saeed, F.; Anjum, F.M.; Afzaal, M.; Tufail, T.; Bashir, M.S.; Ishtiaq, A.; Hussain, S.; Suleria, H.A.R. Natural Polyphenols: An Overview. International Journal of Food Properties 2016, 1–11.
- Pietrzyk, Ł. Food Properties and Dietary Habits in Colorectal Cancer Prevention and Development. International Journal of Food Properties 2016, doi:10.1080/10942912.2016.1236813.
- Halliwell, B. Biochemistry of Oxidative Stress. Biochemical Society Transactions 2007, 35(Pt 5), 1147–1150.
- Ong, H.C.; Nordiana, M. Malay Ethno-medico Botany in Machang, Kelantan, Malaysia. Fitoterapia 1999, 70(5), 502–513.
- Wiart, C. Medicinal Plants of Asia and the Pacific; Boca Raton: CRC/Taylor & Francis, 2006; 306 p., 4 p. of plates.
- Burkill, I.H.; Birtwistle, W. A Dictionary of the Economic Products of the Malay Peninsula; Kuala Lumpur, Malaysia: Ministry of Agriculture Malaysia, 2002.
- Baharum, S.N.; Bunawan, H.; Ghani, M.A.A.; Mustapha, W.A.W.; Noor, N.M., Analysis of the Chemical Composition of the Essential Oil of Polygonum minus Huds. Using Two-dimensional Gas Chromatography-Time-Of-Flight Mass Spectrometry (GC-TOF MS). Molecules 2010, 15(10), 7006–7015.
- Ahmad, R.; Baharum, S.N.; Bunawan, H.; Lee, M.; Mohd Noor, N.; Rohani, E.R.; Ilias, N.; Zin, N.M. Volatile Profiling of Aromatic Traditional Medicinal Plant, Polygonum minus in Different Tissues and Its Biological Activities. Molecules 2014, 19(11), 19220–19242.
- Yaacob, K.B. Essential Oil of Polygonum minus Huds. Journal of Essential Oil Research 1990, 2(4), 167–172.
- Yaacob, K.B. Kesom Oil: A Natural Source of Aliphatic Aldehydes. Perfumer & Flavorist 1987, 12, 27–30.
- Christapher, P.V.; Parasuraman, S.; Christina, J.M.A.; Asmawi, M.Z.; Vikneswaran, M. Review on Polygonum minus. Huds, a Commonly Used Food Additive in Southeast Asia. Pharmacognosy Research 2015, 7(1), 1–6.
- Christapher, P.V.; Parasuraman, S.; Asmawi, M.Z.; Murugaiyah, V. Acute and Subchronic Toxicity Studies of Methanol Extract of Polygonum minus Leaves in Sprague Dawley Rats. Regulatory Toxicology and Pharmacology 2017, 86, 33–41.
- Wan-Ibrahim, W.I.; Sidik, K.; Kuppusamy, U.R. A High Antioxidant Level in Edible Plants Is Associated with Genotoxic Properties. Food Chemistry 2010, 122(4), 1139–1144.
- George, A.; Ng, C.P.; O’Callaghan, M.; Jensen, G.S.; Wong, H.J. In Vitro and Ex-Vivo Cellular Antioxidant Protection and Cognitive Enhancing Effects of an Extract of Polygonum minus Huds (Lineminus (TM)) Demonstrated in a Barnes Maze Animal Model for Memory and Learning. Bmc Complementary and Alternative Medicine 2014, 14(1), 161.
- George, A.; Chinnappan, S.; Chintamaneni, M.; Kotak, C.V.; Choudhary, Y.; Kueper, T. Anti-Inflammatory Effects of Polygonum minus (Huds) Extract (Lineminus (TM)) in In-Vitro Enzyme Assays and Carrageenan Induced Paw Edema. Bmc Complementary and Alternative Medicine 2014, 14(1), 355.
- Ghazali, M.A.M.; Al-Naqeb, G.; Krishnan Selvarajan, K.; Hazizul Hasan, M.; Adam, A. Apoptosis Induction by Polygonum minus Is Related to Antioxidant Capacity, Alterations in Expression of Apoptotic-related Genes, and S-phase Cell Cycle Arrest in Hepg2 Cell. Biomed Research International 2014. https://www.hindawi.com/journals/bmri/2014/539607/cta/
- Qader, S.W.; Abdulla, M.A.; Chua, L.S.; Najim, N.; Zain, M.M.; Hamdan, S. Antioxidant, Total Phenolic Content and Cytotoxicity Evaluation of Selected Malaysian Plants. Molecules 2011, 16(4), 3433–3443.
- Chan, E.W.C.; Tan, Y.P.; Chin, S.J.; Gan, L.Y.; Kang, K.X.; Fong, C.H.; Chang, H.Q.; How, Y.C. Antioxidant Properties of Selected Fresh and Processed Herbs and Vegetables. Free Radicals and Antioxidants 2014, 4(1), 39–46.
- Ali, A.M.; Mackeen, M.M.; El-Sharkawy, S.H.; Abdul Hamid, J.; Ismail, N.H.; Ahmad, F.; Lajis, M.N. Antiviral and Cytotoxic Activities of Some Plants Used in Malaysian Indigenous Medicine. Pertanika Journal of Tropical Agricultural Science 1996, 19(2–3), 129–136.
- Vikram, P.; Chiruvella, K.K.; Ripain, I.H.A.; Arifullah, M. A Recent Review on Phytochemical Constituents and Medicinal Properties of Kesum (Polygonum minus Huds.). Asian Pacific Journal of Tropical Biomedicine 2014, 4(6), 430–435.
- Christapher, P.V.; Parasuraman, S.; Raj, P.V.; Saghir, S.A.M.; Asmawi, M.Z.; Vikneswaran, M. Influence of Extracting Solvent on Pharmacological Activity and Cytotoxicity of Polygonum minus, a Commonly Consumed Herb in Southeast Asia. Pharmacognosy Magazine 2016, 12(Suppl 4), S424–S430.
- Hashim, N.H.N.; Abas, F.; Shaari, K.; Lajis, N.H. LC-DAD-ESIMS/MS Characterization of Antioxidant and Anticholinesterase Constituents Present in the Active Fraction from Persicaria Hydropiper. Lwt-Food Science and Technology 2012, 46(2), 468–476.
- Mackeen, M.M.; Ali, A.M.; El-Sharkawy, S.H.; Manap, M.Y.; Salleh, K.M.; Lajis, N.H.; Kawazu, K. Antimicrobial and Cytotoxic Properties of Some Malaysian Traditional Vegetables (Ulam). International Journal of Pharmacognosy 1997, 35(3), 174–178.
- Ayaz, M.; Junaid, M.; Ahmed, J.; Ullah, F.; Sadiq, A.; Ahmad, S.; Imran, M. Phenolic Contents, Antioxidant and Anticholinesterase Potentials of Crude Extract, Subsequent Fractions and Crude Saponins from Polygonum hydropiper L. BMC Complementary and Alternative Medicine 2014, 14, 145.
- Ayaz, M.; Junaid, M.; Ullah, F.; Sadiq, A.; Khan, M.A.; Ahmad, W.; Shah, M.R.; Imran, M.; Ahmad, S. Comparative Chemical Profiling, Cholinesterase Inhibitions and Anti-radicals Properties of Essential Oils from Polygonum hydropiper L: A Preliminary Anti- Alzheimer’s Study. Lipids in Health and Disease 2015, 14, 141.
- Ayaz, M.; Junaid, M.; Ullah, F.; Sadiq, A.; Subhan, F.; Khan, M.A.; Ahmad, W.; Ali, G.; Imran, M.; Ahmad, S. Molecularly Characterized Solvent Extracts and Saponins from Polygonum hydropiper L. Show High Anti-angiogenic, Anti-tumor, Brine Shrimp, and Fibroblast NIH/3T3 Cell Line Cytotoxicity. Frontiers in Pharmacology 2016, 7, 74.
- Abrahim, N.N.; Kanthimathi, M.S.; Abdul-Aziz, A. Piper Betle Shows Antioxidant Activities, Inhibits MCF-7 Cell Proliferation and Increases Activities of Catalase and Superoxide Dismutase. BMC Complementary and Alternative Medicine 2012, 12, 220.
- Singleton, V.L.; Rossi, J.A. Colorimetry of Total Phenolics with Phosphomolybdic-phosphotungstic Acid Reagents. American Journal of Enology and Viticulture 1965, 16(3), 144–158.
- Chang, C.C.; Yang, M.H.; Wen, H.M.; Chern, J.C., Estimation of Total Flavonoid Content in Propolis by Two Complementary Colorimetric Methods. Journal of Food and Drug Analysis 2002, 10(3), 178–182.
- Benzie, I.F.F.; Strain, J.J. The Ferric Reducing Ability of Plasma (FRAP) as a Measure of “Antioxidant Power”: The FRAP Assay. Analytical Biochemistry 1996, 239(1), 70–76.
- Re, R.; Pellegrini, N.; Proteggente, A.; Pannala, A.; Yang, M.; Rice-Evans, C. Antioxidant Activity Applying an Improved ABTS Radical Cation Decolorization Assay. Free Radical Biology and Medicine 1999, 26(9–10), 1231–1237.
- Brandwilliams, W.; Cuvelier, M.E.; Berset, C. Use of a Free-Radical Method to Evaluate Antioxidant Activity. Food Science and Technology-Lebensmittel-Wissenschaft & Technologie 1995, 28(1), 25–30.
- Robak, J.; Gryglewski, R.J. Flavonoids are Scavengers of Superoxide Anions. Biochemical Pharmacology 1988, 37(5), 837–841.
- Awah, F.M.; Verla, A.W. Antioxidant Activity, Nitric Oxide Scavenging Activity and Phenolic Contents of Ocimum Gratissimum Leaf Extract. Journal of Medicinal Plants Research 2010, 4(23), 2479–2487.
- Decker, E.A.; Welch, B. Role of Ferritin as a Lipid Oxidation Catalyst in Muscle Food. Journal of Agricultural and Food Chemistry 1990, 38(3), 674–677.
- Wolfe, K.L.; Liu, R.H. Cellular Antioxidant Activity (CAA) Assay for Assessing Antioxidants, Foods, and Dietary Supplements. Journal of Agricultural and Food Chemistry 2007, 55(22), 8896–8907.
- Twentyman, P.R.; Luscombe, M. A Study of Some Variables in A Tetrazolium Dye (Mtt) Based Assay for Cell-growth and Chemosensitivity. British Journal of Cancer 1987, 56(3), 279–285.
- Lee, Y.H.; Choo, C.; Waisundara, V.Y. Determination of the Total Antioxidant Capacity and Quantification of Phenolic Compounds of Different Solvent Extracts of Black Mustard Seeds (Brassica nigra). International Journal of Food Properties 2015, 18(11), 2500–2507.
- Zagmutt, S.; Guzmán, L.; Orrego, R.; Wehinger, S.; Leiva, E. Phenolic Compound Identification and Antioxidant Capacity of Alperujo Extracts from Region Del Maule, Chile. International Journal of Food Properties 2016, 19(9), 2016–2025.
- Mahayothee, B.; Koomyart, I.; Khuwijitjaru, P.; Siriwongwilaichat, P.; Nagle, M.; Müller, J. Phenolic Compounds, Antioxidant Activity, and Medium Chain Fatty Acids Profiles of Coconut Water and Meat at Different Maturity Stages. International Journal of Food Properties 2016, 19(9), 2041–2051.
- Huang, W.Y.; Cai, Y.Z.; Xing, J.; Corke, H.; Sun, M. Comparative Analysis of Bioactivities of Four Polygonum Species. Planta Medica 2008, 74(1), 43–49.
- Sulaiman, S.F.; Sajak, A.A.B.; Ooi, K.L.; Seow, E.M. Effect of Solvents in Extracting Polyphenols and Antioxidants of Selected Raw Vegetables. Journal of Food Composition and Analysis 2011, 24(4–5), 506–515.
- Borneo, R.; León, A.E.; Aguirre, A.; Ribotta, P; Cantero, J.J. Antioxidant Capacity of Medicinal Plants from the Province of Cordoba (Argentina) and Their in Vitro Testing in a Model Food System. Food Chemistry 2009, 112(3), 664–670.
- Yokozawa, T.; Chen, C.P.; Tanaka, T. Direct Scavenging of Nitric Oxide by Traditional Crude Drugs. Phytomedicine 2000, 6(6), 453–463.
- Nakagawa, T.; Yokozawa, T. Direct Scavenging of Nitric Oxide and Superoxide by Green Tea. Food and Chemical Toxicology 2002, 40(12), 1745–1750.
- Song, W.; Derito, C.M.; Liu, M.K.; He, X.; Dong, M.; Liu, R.H. Cellular Antioxidant Activity of Common Vegetables. Journal of Agricultural and Food Chemistry 2010, 58(11), 6621–6629.
- Popiolkiewicz, J.; Polkowski, K.; Skierski, J.S.; Mazurek, A.P. In Vitro Toxicity Evaluation in the Development of New Anticancer Drugs - Genistein Glycosides. Cancer Letters 2005, 229(1), 67–75.
- Diederich, M.; Cerella, C. Non-Canonical Programmed Cell Death Mechanisms Triggered by Natural Compounds. Seminars in Cancer Biology 2016, 40-41, 4–34.