ABSTRACT
To comprehensively examine the antioxidant capacity of Perilla frutescens leaves extract, eight assays including hydroxyl radical, hydrogen peroxide, ferrous ion-chelating, total antioxidant capacity, nitric oxide, 2,2′-azino-bis(3-ethylbenzothiazoline-6-sulfonic acid), 2,2′-diphenyl-1-picrylhydrazyl, and ferrous reducing antioxidant assays were employed. The results indicated that the ethyl acetate–soluble fraction of P. frutescens leaves extract displayed the highest antioxidant activities. The antioxidant constituents from ethyl acetate–soluble fraction of P. frutescens leaves extract were screened by 2,2′-diphenyl-1-picrylhydrazyl high-performance liquid chromatography, and 11 compounds were identified after isolation using step-wise high-speed countercurrent chromatography. Protocatechuic acid, chlorogenic acid, caffeic acid, rosmarinic acid, luteolin, methyl-rosmarinic acid, apigenin, and 4′,5,7-trimethoxyflavone are the major antioxidant constituents in ethyl acetate–soluble fraction of P. frutescens leaves extract.
Introduction
Free radicals play an important role in disease pathogenesis. The reactive oxygen species (ROS) and reactive nitrogen species (RNS) produced in response to inflammation, oxidation of proteins, lipids, and DNA are the most important free radicals produced as a result of cellular metabolism. Free radical ROS and RNS include superoxide, hydroxyl, and nitric oxide radicals; and non-radical ROS and RNS include singlet oxygen, hydrogen peroxide, hypochlorous acid, and peroxynitrite. The body maintains a balance of ROS and RNS by various radical scavenging mechanisms,[1] however, imbalance of ROS and RNS can result in diseases, such as aging, cancer, Parkinson’s disease, blood vessel disorders, heart failure, etc. Thus, the antioxidant, the substance which scavenges free radicals and inhibits the oxidation of other molecules in the human body, is necessary. Various methods are used to investigate the antioxidant property of samples. Normally, the in vitro antioxidant assays can be divided into two main categories. One category is based on electron transfer (ET) from the antioxidant resulting in a color change that can be measured spectrophotometrically, such as ferric reducing/antioxidant power (FRAP), 2,2′-diphenyl-1-picrylhydrazyl (DPPH), 2,2′-azino-bis(3-ethylbenzothiazoline-6-sulfonic acid) (ABTS), metal-chelating activity (FIC), and total antioxidant capacity (TAC) assays; the other category includes the assays that measure the ROS/RNS scavenging capacity, such as hydroxyl radical (HO•), hydrogen peroxide (H2O2), and nitric oxide (NO) assays. In the past several decades, a number of synthetic antioxidants, such as butylated hydroxyanisole and butylated hydroxytoluene (BHT) were discovered. Because of concerns about the safety of synthetic antioxidant,[2] there is increasing interest in the development of non-toxic and healthier antioxidant from natural sources in food industry.[3,4]
Perilla frutescens (L.) Britt (PF), commonly referred to as perilla, is an annual short-day plant belonging to the mint family Lamiaceae, which is primarily cultivated and consumed in Asian countries such as Korea, China, and Japan.[5–7] Perilla is an important crop source of oil and edible vegetable with many beneficial properties including anti-inflammatory, anti-allergic, anti-carcinogenic effects, and antioxidant.[8–10] The seeds of perilla are commonly used for oil extraction.[5] Its young raw leaves are often used in salads, sushi, and soup, as well as for pickles, condiment, garnishes, and food colorants.[8,11] Its essential oils show anti-inflammatory, anti-depression, anti-hyperlipidemia, and antimicrobial activities and are also used in perfumes, soaps, detergents, and cosmetics.[11–15] Perilla is also a traditional Chinese medicinal herb, which has been used in China for centuries to treat various diseases including depression, anxiety, tumors, chronic coughs, bacterial and fungal infections, intoxication, and some intestinal disorders. Today, perilla is introduced into Europe and North America because of its economic importance, and its seed oil and dried leaf extracts are used for a variety of health-promoting purposes.[16] The recent work of our research team reported that the perilla leaves extract (PLE) showed inhibitory activities on aldose reductase and xanthine oxidase.[17,18] Furthermore, literature has indicated enhanced cholesterol efflux from oxidized low-density lipoprotein (LDL)-exposed macrophases, and α-asarone was isolated from PLE and characterized as a major component enhancing the induction of adenosine triphosphate (ATP)-binding cassette sub-family A member 1 and ATP-binding cassette sub-family G member 1 in macrophages exposed to oxidized LDL.[19] We also reported information regarding α-asarone content and the most appropriate extraction conditions.[20] Previously, perilla was reported to contain various flavonoids: apigenin, luteolin, and scutellarein; triterpene acids: ursolic acid, oleanolic acid, and tormentic acid; phenolic acids: rosmarinic acid, caffeic acid, ferulicacid; anthocyanin: shisonin; sterols: β-sitosterol, stigmansterol, and campesterol among others.[21–23] Recent studies have revealed that perilla possesses excellent antioxidant activities which can be attributed to its phenolic and flavonoid content.[24] Owing to the various types of free radicals, the mechanism by which antioxidants exert their activity is complex. Therefore, it is difficult to assess the antioxidant activity of PLE using a single assay. Alternatively, we comprehensively examined the antioxidant activity of PLE via eight assays in this study.
The profiling of antioxidants using LC-MS has limitations on structure elucidation and may be erroneous. Therefore, we isolated and identified the antioxidant constituents from PF using step-wise high-speed countercurrent chromatography (HSCCC) guided by DPPH-high performance liquid chromatography (HPLC) assay. DPPH-HPLC is a simple and rapid screening method that aids in the rapid selection of target components in complex extracts and has been widely used in many studies.[25–27] HSCCC is a unique separation technique based on the liquid–liquid partition chromatography without any solid support matrix. Therefore, it prevents the loss of sample observed in the conventional adsorption chromatography.[28] The centrifugal force provided by rotation retains the stationary phase in the coil tubes.[29] Recently, gradient elution has been used in HSCCC for separation of natural products; however, little attention has been given to the use of solvent system gradients, in contrast to the usual isocratic elution.[30] Step-wise elution applied to HSCCC may be the choice for the purification of mixtures containing compounds with similar polarities, where slight differences in their partition coefficients must be exploited.[31] Moreover, some solvent systems are stable to the drastic changes in the mobile phase composition during separation. Therefore, a step-wise elution mode applied to HSCCC appears to simplify the separation of crude plant extracts, whose constituents vary over a wide range of polarity.[32] Thus, the aim of this study is to comprehensively examine the antioxidant capacity of PLE and isolate its free radical scavengers using step-wise HSCCC guided by DPPH-HPLC.
Materials and methods
Chemicals
Dried leaves of PF were purchased from a local market in Chuncheon, Korea. A voucher sample (RIC-2012-5) has been deposited at the Center for Efficacy Assessment and Development of Functional Foods and Drugs, Hallym University, Chuncheon, Korea. Methanol, n-hexane (n-Hex), methylene chloride (MC), ethyl acetate (EtOAc), and n-butanol (n-BuOH) were purchased from J.T. Baker (Phillipsburg, NJ, USA). Ultrapure water, used for all solutions, dilutions, and HPLC analysis, was generated using a Milli-Q laboratory water purification system (Millipore, Bedford, MA, USA) with a resistivity over 18.2 MΩ cm. ABTS diammonium salt, ammonium ferrous sulfate, ammonium molybdate, BHT, DPPH, ferrous chloride, ferrous sulfate, ferrozine, Griess reagent, hydrogen peroxide, potassium ferricyanide, sodium nitroprusside, sodium phosphate, sulfuric acid, potassium persulfate, sodium salicylate, sulfuric acid, trichloroacetic acid, and xylenol orange were purchased from Sigma-Aldrich Chemical Co. (St. Louis, MO, USA).
Preparation of plant extracts
Dried leaves of PF (2 kg) were extracted three times with methanol for 3 h. The PLE was suspended in distilled water and partitioned with n-Hex, MC, EtOAc, and n-BuOH to yield n-Hex (40.84 g), MC (25.21 g), EtOAc (22.24 g), n-BuOH (116.88 g), and water (27.43 g) fractions.
HO• scavenging assay
HO• scavenging activity was measured according to the modified procedure used by Su et al.[33] Briefly, the reaction mixture which consisted of 0.5 mL of ferrous sulfate (8 mM), 0.8 mL of hydrogen peroxide (6 mM), 0.5 mL of distilled water, 1.0 mL of the samples (final concentration: 0.33 mg/mL), and 0.2 mL of sodium salicylate (20 mM) was incubated at 37°C for 1 h. Then the absorbance of the mixture was recorded at 562 nm. Ascorbic acid was used as a positive control. HO• scavenged (%) was calculated as follows: [1 – {(A1 – A2)/A0}] × 100%, where A0 is the absorbance of the control (without extract), A1 is the absorbance after the addition of the extract, and A2 is the absorbance without sodium salicylate.
H2O2 scavenging assay
The H2O2 scavenging activity of PF was determined according to a previously described method with minor changes. [34] The ferrous ion oxidation xylenol orange (FOX) reagent was first prepared by mixing BHT (87.3 mg), sulfuric acid (10 μL), xylenol orange (7.6 mg), ammonium ferrous sulfate (10 mg) in 100 mL of 90% methanol–water solution. Aliquots of 50 mM H2O2 and the samples (final concentration: 0.2 mg/mL) were mixed (1:1, v/v) and incubated for 30 min at room temperature. After incubation, 90 μL of the H2O2 sample solution was mixed with 10 μL of HPLC-grade methanol. Then, 0.9 mL of FOX reagent was added. The reaction mixture was then vortexed and incubated at room temperature for 30 min. The absorbance of the ferric–xylenol orange complex was measured at 560 nm. Ascorbic acid was used as a positive control. H2O2 scavenged (%) was calculated as follows: [1 – {(A1 – A2)/A0}] × 100%, where A0 is the absorbance of the control (without extract), A1 is the absorbance after the addition of the extract, and A2 is the absorbance without FOX reagent.
FIC assay
The FIC assay was carried out as described by Hsu et al.[35] Briefly, 0.5 mL of BHT (1 mmol) or extract samples (final concentration: 0.5 mg/mL) were mixed with 100 μL of ferrous chloride solution (0.6 mM) and 0.9 mL of methanol. The mixture was left at room temperature for 10 min. Then, 0.1 mL of ferrozine solution (5 mM) was added and the reaction mixture was left at room temperature for 5 min. The absorbance of the mixture was determined at 562 nm. The FIC effect (%) was calculated from the decrease in the absorbance against the control.
NO radical scavenging assay
NO, generated from sodium nitroprusside, was measured according to Nitha et al.[36] A final volume of 0.1 mL of extract samples (final concentration: 66.67 µg/mL) in 0.2 mL of phosphate buffered saline containing sodium nitroprusside (5 mM) was incubated at 25°C for 150 min. Thereafter, aliquots of the mixture (0.5 mL) were removed and diluted with 0.5 mL of Griess reagent. The absorbance was measured at 546 nm and the inhibition of NO generation was estimated by comparing the absorbance values to that of the control without the extract. NO scavenged (%) was calculated as follows: [1 – (A1/A0)] × 100%, where A0 is the absorbance of the control (without extract), A1 is the absorbance of the sample after the reaction.
TAC assay
The TAC was determined according to Oliveira et al.[37] The samples (final concentration: 90.91 µg/mL; 0.1 mL) were added in eppendorf tubes and mixed with 1 mL of the reagent solution containing sulfuric acid (0.6 M), sodium phosphate (28 mM), and ammonium molybdate (4 mM). The tubes were capped and incubated in a thermal block heater at 95°C for 90 min. The absorbance was measured at 695 nm after the samples were allowed to cool to the room temperature. Ascorbic acid (final concentration: 4.55, 9.09, 22.73, 45.45, 68.18, and 90.91 µg/mL) was used as a positive control.
FRAP assay
The FRAP was determined according to Amarowicz et al.[38] Briefly, each fraction of the extract (1.18, 58.82, 294.12, and 588.24 µg/mL) was dissolved in 1.0 mL of distilled water to which 2.5 mL of a 0.2 M phosphate buffer (pH 6.6) and 2.5 mL of 1% (w/v) potassium ferricyanide solution were added. The mixture was incubated in a water bath at 50°C for 20 min. After that, 2.5 mL of 10% (w/v) trichloroacetic acid solution was added and the mixture was then centrifuged at 1750g for 10 min. A 2.5 mL aliquot of the upper layer was mixed with 2.5 mL of distilled water and 0.5 mL of 0.1% (w/v) ferric chloride solution. The absorbance of the reaction mixture was measured spectrophotometrically at 750 nm; an increased absorbance of the reaction mixture indicates greater reducing power. The mean values of three independent samples were calculated for each fraction of the extract.
ABTS assay
The ABTS assay was performed according to Wang et al.[39] with slight modification. In brief, 10 µL of the diluted sample was mixed with 290 µL ABTS solution (2 mM ABTS diammonium salt, 3.5 mM potassium persulfate) and then completed with methanol to a final volume of 300 µL. The ABTS solution served as a control. Then, the absorbance was measured at 750 nm after the mixture was incubated for 10 min. The antioxidant activity was expressed as the percentage of ABTS radical elimination and calculated according to the following formula: [(Ablank – Asample)/Ablank] × 100%, where Ablank is the absorbance of the blank ABTS solution, and Asample is the absorbance of the ABTS solution after the addition of the sample. The sample concentration providing 50% inhibition (IC50) was calculated from the graph of the inhibition percentage versus the concentration.
DPPH assay
The free radical scavenging activity of the crude extract and the isolated compounds was determined by the decolorization of the DPPH radical. In brief, 30 µL of the diluted sample was mixed with 180 µL DPPH solution (0.13 mg/mL) and then completed with methanol to a final volume of 210 µL. The DPPH solution served as a control. Then, the absorbance was measured at 517 nm after the mixture was incubated for 20 min. The antioxidant activity was expressed as the percentage of DPPH radical elimination and calculated according to the following formula: [(Ablank – Asample)/Ablank] × 100%, where Ablank is the absorbance of the blank DPPH solution, and Asample is the absorbance of the DPPH solution after the addition of the sample. The IC50 was calculated from the graph of the inhibition percentage versus the concentration.
DPPH-HPLC experiment
The EtOAc fraction of the PLE was treated with DPPH according to the same procedure described for the colorimetric analysis of the DPPH radical (DPPH assay section). The mixture was then passed through a 0.45 µm filter and subjected to HPLC analysis according to the methods described in “HPLC analysis” section. A blank of the aqueous extract was used as a control. The peaks corresponding to the antioxidant compounds were supposed to decrease in magnitude or even disappear after the reaction.
HPLC analysis
The HPLC analysis of each filtrate was performed on an Agilent 1200 series instrument (Agilent Technologies, Seoul, Korea). Separation was achieved on an Eclipse SB-C18 Rapid Resolution column (150 mm length, 4.6 mm inner diameter (ID) and 3.5 µm particle size; Agilent), coupled with a guard column, at 30°C. Briefly, the samples (10 µL) were injected into the system and eluted with acidified water (0.1% trifluoroacetic acid, A) and methanol (B), at a flow rate of 0.7 mL/min. The gradient elution system was modified as follows: 0–35 min, A-linear gradient starting with 5% B-line, was programmed installed to reach 50% B-line at 15 min using a linear gradient, followed by 100% B-line from at 15 to 23 min. The eluent was monitored using a UV detector at a wavelength of 254 nm.
Step-wise HSCCC
The instrument employed for HSCCC was a TBE-300C HSCCC (Tauto Biotechnique Company, Shanghai, China). The apparatus, with a maximum rotational speed of 1000 rpm, was equipped with three polytetrafluoroethylene preparative coils (ID: 2.6 mm, total volume: 300 mL) and a 20 mL sample loop. The β values of the multilayer coil varied from 0.5 at the internal terminal to 0.8 at the external terminal. Solvents were delivered by an Isolera FLASH purification system (Biotage, Uppsala, Sweden), which was used to pump the two-phase solvent system and to carry out the UV absorbance measurement. HSCCC was performed as follows: the multilayer-coiled column was first entirely filled with the lower phase of the selected solvent system as a stationary phase at a flow rate of 20 mL/min for 15 min. Then, the column rotor was started at 700 rpm, and the upper phase was pumped through the column at a flow rate of 4 mL/min entering in a tail-to-head direction. After a hydrodynamic equilibrium was reached, as indicated by a clear mobile phase, the sample solution (200 mg in 3 mL of the stationary phase; n-BuOH:water = 1:1, v/v) was injected. The effluent was monitored with a UV detector at 280 nm, and fractions were collected in test tubes every 8 min (32 mL) by the fraction collector. The peak fractions were pooled according to the elution profile and the analytical HPLC detection. The fractions were then separated by solvent system 1 to obtain compound 11 (2.2 mg), compound 10 (3.3 mg) at solvent system 2, compound 9 (10.0 mg) and compound 3 (3.6 mg) at solvent system 3, compound 4 (2.2 mg) at solvent system 4, and compound 2 (4.4 mg) and compound 7 (20.4 mg) at solvent system 5. The solvent system is shown in () In addition, based on reference papers, another four compounds (protocatechuic acid, oleanolic acid, kaempferol-3-O-rutinoside, and luteolin) were identified by standards that were purchased from Sigma-Aldrich using HPLC.[40–43]
Structure identification
The 1H and 13C nuclear magnetic resonance (NMR) spectra of the seven isolated pure compounds were recorded using the Bruker AV 600 instrument, using MeOH-d4 as a solvent. Moreover, the isolated compounds were analyzed by electron ionization mass spectrometry (EI-MS) performed in a LR-mass equipped with JEOL JMS-700. The mass spectrometer was operated in the negative-ion mode with an electron impact of 70 eV with direct insertion probe. The ion source was set at 250°C and the mass range was 50–600 m/z.
Results and discussion
Comprehensive evaluation of PLE antioxidant activity
Owing to the various types of free radicals, the mechanism by which antioxidants exert their activity is complex. Normally, the in vitro antioxidant assays can be divided into two main categories. One category is based on ET from the antioxidant resulting in a color change that can be measured spectrophotometrically; the other category includes the assays that measure the ROS scavenging capacity. In this study, we performed five assays (FRAP, DPPH, ABTS, FIC, and TAC) of the ET category and three assays (HO∙, H2O2, NO) of the ROS category to evaluate the antioxidant activity of PF.
HO• is one of the major ROS that is continuously formed in the process of reduction of oxygen to water. HO• causes oxidative stress; furthermore, it can lead to DNA strand breaks, oxidation of the protein amino acids, and glycation of proteins, among others.[44] As shown in , the HO• scavenging activity of PF methanol extract was evaluated first and a 6.05% inhibition was obtained at the concentration of 33.33 µg/mL. Even though the inhibitory activity of the crude extract is much lower than that of ascorbic acid (10.19% at 33.33 µg/mL), but it still indicates that potent HO• scavengers exist in the matrix. Therefore, we applied solvent fractionation to separate five fractions for enrichment of the active compounds and their inhibitory activities were tested again. It was found that the aqueous fraction exhibited the highest HO• scavenging activity (8.54% at 33.33 µg/mL), which was still a little lower than that of ascorbic acid.
Figure 1. Evaluation of Perilla frutescens antioxidant activity. (A) Hydroxyl radical scavenging activity of the fractions isolated from P. frutescens. The concentration of the fractions was 33.33 μg/mL. (B) Hydrogen peroxide scavenging activity of the fractions isolated from P. frutescens. The concentration of the fractions was 200 μg/mL. (C) Ferrous ion chelating activity of the fractions isolated from P. frutescens. The concentration of the fractions was 200 μg/mL. (D) Nitric oxide scavenging activity of the fractions isolated from P. frutescens. The concentration of the fractions was 66.67 μg/mL. The absorbance values were converted to scavenging effects (%). (E) Total antioxidant capacity of the fractions isolated from P. frutescens (expressed as equivalents of ascorbic acid). (F) Ferric reducing/antioxidant power of the fractions isolated from P. frutescens. (G) ABTS scavenging activity of the fractions isolated from P. frutescens. (H) DPPH scavenging activity of the fractions isolated from P. frutescens. Na is not active.
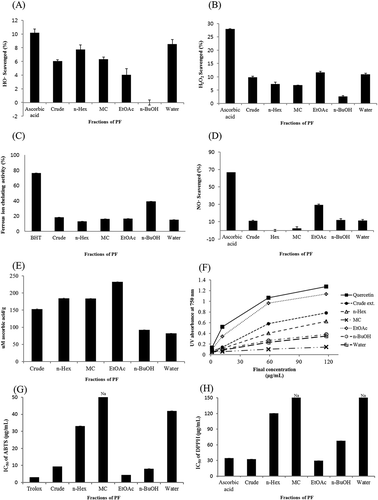
H2O2 is not very reactive; however, it induces cellular aging and can attack many cellular systems due to its high penetrability through the cell membranes.[45] The H2O2 scavenging activity of ascorbic acid, the crude extract, and five fractions (n-Hex, MC, EtOAc, n-BuOH, water residue) was approximately 27.91%, 9.87%, 7.33%, 6.95%, 11.64%, 2.69%, 10.97% at a concentration of 200 µg/mL, respectively (). Compared with ascorbic acid, the solvent fractions of the extract exhibited a lower H2O2 scavenging activity. The EtOAc-soluble fraction exhibited the highest H2O2 scavenging activity and accordingly was considered the active fraction.
Ferrozine can quantitatively form complexes with FeCitation2+. In the presence of chelating agents, the complex formation is disrupted, which results in a decreased red color of the complex. Therefore, measurement of color reduction allows the estimation of the chelating activity of the co-existing chelator. The FIC activity of the five solvent fractions isolated from the PF crude extract is presented in . In this assay, the crude extract, the five solvent fractions isolated from the crude extract and BHT, interfered with the formation of ferrous–ferrozine complex, suggesting that they have chelating activity and can capture the ferrous ions before ferrozine. It was found that the n-BuOH-soluble fraction showed a higher metal chelating activity.
NO is normally considered a vascular protective molecule. However, NO reacts with superoxide radical to generate the highly reactive molecule, ONOO–, which results in a cascade of harmful events as discussed below.[46] The nitric oxide scavenging activity of the fractions isolated from the PF crude extract is presented in . The EtOAc-soluble fraction showed the highest NO scavenging activity (29.38% at 66.67 μg/mL). Ascorbic acid showed a scavenging activity of 66.63% at the same concentration. Although the nitric oxide scavenging activity of our active sample was lower than that of the positive control, a higher nitric oxide scavenging activity is predicted if the experiment is performed on the pure active compounds isolated from the active fraction.
The TAC of the solvent fractions is presented in . As described in , the solvent fractions were found to have strong and increasing antioxidant capacity at concentrations of 1.18–588.24 μg/mL. The EtOAc-soluble fraction showed the highest TAC at a concentration of 181.18 μg/mL of ascorbic equivalent to 232.67 μM ascorbic acid/g.
FRAP assay measures the reduction of ferric iron (FeCitation3+) to ferrous iron (FeCitation2+) in the presence of antioxidants.[47] This assay is commonly used for the analysis of TAC of natural product extracts. Results of the ferric reducing power of the selected solvent fractions are presented in . The EtOAc-soluble fraction showed very strong ferric ion reducing activity (1.14 at 117.65 μg/mL; absorbance at 750 nm) and the MC-soluble fraction possessed the lowest ferric reducing capacity (0.14 at 117.65 μg/mL; absorbance at 750 nm).
ABTS and DPPH assays measure the antioxidant potential of natural products using single-electron transfer mechanism.[48] In addition, it is simple and common method for determination of the antioxidant activity. Results of ABTS and DPPH assays are presented in and . The EtOAc-soluble fraction showed the highest antioxidant activity similar to that of the positive control. Particularly, the EtOAc-soluble fraction of the PF crude extract has a higher antioxidant activity (IC50 29.85 μg/mL) than that of ascorbic acid (IC50 34.40 μg/mL) in the DPPH assay.
The correlation coefficients (r) of each two assays were calculated as shown in . The high correlations suggested the radical scavengers for these two radicals are same or similar; however, poor correlations suggested the components of PF fractions that act as antioxidants for these two radicals are not the same. Finally, DPPH scavenging activity showed correlations with ABTS (r = 0.99) and HO• (r = 0.28) scavenging activities; and FRAP antioxidant activity showed correlations with H2O2 (r = 0.46), NO∙ (r = 0.83), and TAC (r = 0.62) antioxidant activities. FIC showed no correlation with any other antioxidant activity. These results indicated that the results of DPPH and FRAP assays could be used to represent most antioxidant activities, and the EtOAC fraction of PF extract showed most potent antioxidant capacity.
Table 1. Correlation between free radical scavenging and different antioxidant activities of the different fractions of PF.
Screening and isolation of potential antioxidants
It is necessary to identify the antioxidant constituents from EtOAc fraction of PLE. Thus, DPPH-HPLC experiment was applied using the EtOAc-soluble fraction. The peak area of the active compound is expected to decrease after addition of the DPPH solution. If the compound does not possess an antioxidant activity, its peak will show no reduction. As shown in , compounds 1, 2, 3, 7, 9, 10, and 11 showed reduced peak areas. Consequently, compounds 7 and 9 showed greatest reduction of the peak area may possess an antioxidant activity.
Figure 2. HPLC-UV (red line) and DPPH-HPLC-UV (blue line) analyses of the EtOAc fraction of the Perilla frutescens leaves. The full chromatogram (A) and extracted peaks for compounds 1–11 (B–K). HPLC conditions: column, Eclipse SB-C18 Rapid Resolution column (150 × 4.6 mm, 5 μm, Agilent); mobile phase, consisted of A (0.1% trifluoroacetic acid in water) and B (methanol), which was programmed as follows: 0–15 min, 5–50% B, 15–23 min, 50–100% B; flow rate, 0.7 mL/min; UV wavelength, 254 nm; column temperature, 30°C.
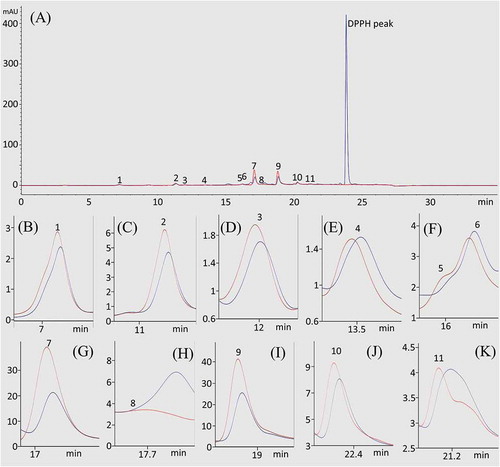
The step-wise HSCCC was subsequently performed to isolate the antioxidant constituents. The mobile phases and chromatography of step-wise gradient elution are shown in and , respectively. The purity of the isolated compounds, including chlorogenic acid (2), caffeic acid (3), 4-methyoxycinnamic acid (4), rosmarinic acid (7), methyl-rosmarinic acid (9), apigenin (10), and 4′,5,7-trimethoxyflavone (11), was checked by HPLC (). The structures of compounds () were identified by standard compounds, NMR, and EI-MS compared with previously reported data associated with or without MS analysis.[17, 49–55] The detailed structural information is listed in supporting information. Another four compounds (protocatechuic acid (1), oleanolic acid (5), kaempferol-3-O-rutinoside (6), and luteolin (8)) were identified by standard references. Moreover, as shown in , the contents of the major antioxidant constituents in EtOAc fraction of PLC were provided, of which chlorogenic acid (2), caffeic acid (3), 4-methyoxycinnamic acid (4), rosmarinic acid (7), methyl-rosmarinic acid (9), apigenin (10), and 4′,5,7-trimethoxyflavone (11) were calculated through their isolation yields, protocatechuic acid (1), oleanolic acid (5), kaempferol-3-O-rutinoside (6), and luteolin (8) were calculated by optimized HPLC.
Figure 3. Separation of antioxidants from Perilla frutescens using step-wise HSCCC. (A) Phase diagram for the n-Hex:EtOAc:n-BuOH solvent system; (B) step-wise elution chromatogram of the HSCCC; (C) HPLC analysis of the isolated compounds; (D) structures of compounds from P. frutescens; (E) the content of each compound in ethyl acetate fraction of P. frutescens leaves extract.
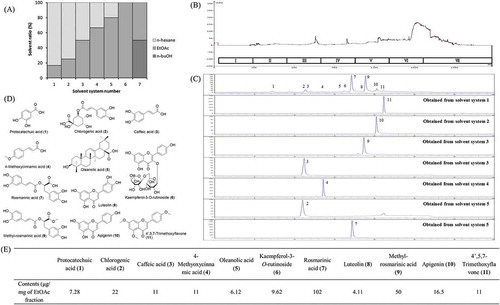
Antioxidant activity of the identified compounds
The identified compounds were evaluated for their antioxidant activity using DPPH assay and FRAP assay. For DPPH assay, as shown in , compound 7 (rosmarinic acid) has an IC50 of 27.31 μg/mL, which is similar to that of the well-known natural antioxidants, followed by compound 3 (caffeic acid) with an IC50 of 39.86 μg/mL, compound 8 (luteolin) with an IC50 of 43.52 μg/mL, and compound 9 (methyl-rosmarinic acid) with an IC50 of 44.28 μg/mL. For FRAP assay, as shown in and , compound 3 possessed the highest ferric reducing activity, which was even higher than that of the positive control, followed by compound 9, compound 1 (protocatechuic acid), and compound 7. The ferric reducing effects decreased in the order of compound 3, 9, 7, 1 (0.235, 0.226, 0.221, 0.218 µM quercetin/g at a concentration of 23.53 μg/mL). Compounds 1 and 7 showed nearly the same activity. Based on these results, the presence or absence of caffeoyl group can have an important impact on the antioxidant activity. According to the literature, the presence of the CH=CH–COOH group in cinnamic acid provides a greater antioxidant power than the COOH group in benzoic acid.[56] Accordingly, protocatechuic acid, with a similar structure, showed lower inhibitory activity because it does not have the CH=CH group. There are subtle differences between the results of the FRAP and DPPH assays; however, the caffeoyl group seems to have an important impact on the antioxidant activity as measured by the two assays. Compound 8 exhibited the third highest antioxidant activity because of existence of a catechol structure and 2,3-double bond conjugated with a 4-oxo function.[57] Compounds 7 and 9 might be the major contributors to the antioxidant activity of EtOAc fraction of PLE because they were the main components that had 102 and 50 μg/mg of EtOAc fraction (), respectively, although other compounds also showed considerable antioxidant capacity.
Conclusion
In this study, the antioxidant capacity of PLE was examined by HO•, H2O2, FIC, TAC, NO, ABTS, DPPH, and FRAP assays. The PLE showed considerable antioxidant activities on all assays. Moreover, EtOAc fraction of PLE showed highest antioxidant activity on H2O2, NO, TAC, FRAP, ABTS, and DPPH; n-Hex fraction showed highest antioxidant activity on HO•; n-BuOH fraction showed highest antioxidant activity on FIC. In order to identify the antioxidant constituents from EtOAc fraction of PLE, the DPPH-HPLC assay was performed. Thereafter, 11 compounds, namely protocatechuic acid (1), chlorogenic acid (2), caffeic acid (3), 4-methoxycinnamic acid (4), oleanolic acid (5), kaempferol-3-O-rutinoside (6), rosmarinic acid (7), luteolin (8), methyl-rosmarinic acid (9), apigenin (10), and 4′,5,7-trimethoxyflavone (11), were identified by reference standard compounds or NMR and EI-MS after isolation using step-wise HSCCC. Finally, protocatechuic acid (1), chlorogenic acid (2), caffeic acid (3), rosmarinic acid (7), luteolin (8), methyl-rosmarinic acid (9), apigenin (10), and 4′,5,7-trimethoxyflavone (11) are found to be the major antioxidant constituents in EtOAc fraction of PLE. These results suggested that P. frutescens can be a potent functional food ingredient with excellent antioxidant properties.
LJFP_A_1318289_Supporting_information.docx
Download MS Word (18.4 KB)Acknowledgments
We thank Soo Kyeong Lee for conducting experiments helpful in this study.
Funding
This study was supported by the High Value-added Food Technology Development Program, Korea Institute of Planning and Evaluation for Technology in Food, Agriculture, Forestry and Fisheries (112085-03-3-HD030).
Supplemental data
Supplemental data for this article can be accessed on the publisher’s website.
Additional information
Funding
References
- Halliwell, B.; Gutteridge, J.M.C. Free Radical in Biology and Medicine; Oxford Press: Oxford, UK, 1999.
- Kahl, R.; Kappus, H. Toxicology of the Synthetic Antioxidants BHA and BHT in Comparison with the Natural Antioxidant Vitamin E. Zeitschrift für Lebensmittel-Untersuchung and Forschung 1993, 19, 329–338.
- Elmastas, M.; Isildak, O.; Turkekul, I.; Temur, N. Determination of Antioxidant Activity and Antioxidant Compounds in wild Edible Mushrooms. Journal of Food Composition and Analysis 2007, 20, 337–345.
- Gülçin, I.; Mshvildadze, V.; Gepdiremen, A.; Elias, R. The Antioxidant Activity of a Triterpenoid Glycoside Isolated from the Berries of Hedera colchica: 3-O-(β-D-glucopyranosyl)-Hederagenin. Phytotherapy Research 2006, 20, 130–134.
- Hu, Y.; Sung, L.W.; Mokgolodi, N.C.; Zhang, Y.X.; Wen, C.X.; Xie X.L.; Liu, Y.J. Primary Identifications and Palynological Observations of Perilla in China. Journal of Systematics and Evolution 2010, 48, 133–145.
- Ha, T.J.; Lee, J.H.; Lee, M.H.; Lee, B.W.; Kwon, H.S.; Park, C.H.; Shim, K.B.; Kim, H.T.; Baek, I.Y.; Jang, D.S. Isolation and Identification of Phenolic Compounds from the Seeds of Perilla frutescens (L.) and Their Inhibitory Activities Against α-Glucosidase and Aldose Reductase. Food Chemistry 2012, 135, 1397–1403.
- Igarashi, M.; Miyazaki, Y. A Review on Bioactivities of Perilla: Progress in Research on the Functions of Perilla as Medicine and Food. Evidence-based Complementary and Alternative Medicine 2013, 925342.
- Meng, L.; Lozano, Y.F.; Gaydou, E.M.; Li, B. Antioxidant Activities of Polyphenols Extracted from Perilla frutescens Varieties. Molecules 2009, 14, 133–140.
- Ueda, H.; Yamazaki, C.; Yamazaki, M. Luteolin as an Anti-inflammatory and Anti-Allergic Constituent of Perilla frutescens. Biological and Pharmaceutical Bulletin 2002, 25, 1197–1202.
- Osakabe, N.; Yasuda, A.; Natsume, M.; Yoshikawa, T. Rosmarinic Acid Inhibits Epidermal Inflammatory Responses: Anticarcinogenic Effect of Perilla frutescens Extract in the Murine Two-Stage Skin Model. Carcinogenesis 2003, 25, 549–557.
- Liu, J.; Wan, Y; Zhao, Z.; Chen, H. Determination of the Content of Rosmarinic Acid by HPLC and Analytical Comparison of Volatile Constituents by GC–MS in Different Parts of Perilla frutescens (L.) Britt. Chemistry Central Journal 2013, 7, 61.
- Buchwald-Werner, S.; Fujii, H.; Schön, C.; Doebis, C. Investigation of a Perilla frutescens Special Extract - Anti-inflammatory and Immune-modulatory Properties. Agro Food Industry Hi Tech 2012, 23, 38–41.
- Feng, L.J.; Yu, C.H.; Ying, K.J.; Hua, J.; Dai, X.Y. Hypolipidemic and Antioxidant Effects of Total Flavonoids of Perilla frutescens Leaves in Hyperlipidemia Rats Induced by High-fat Diet. Food Research International 2011, 44, 404–409.
- Kang, R.; Helms, R.; Stout, M.J.; Jaber, H.; Chen, Z.; Nakatsu, T. Antimicrobial Activity of the Volatile Constituents of Perilla frutescens and Its Synergistic Effects with Polygodial. Journal of Agricultural and Food Chemistry 1992, 40, 2328–2330.
- Nakazawa, T.; Yasuda, T.; Ueda, J.; Ohsawa, K. Antidepressant-like Effects of Apigenin and 2,4,5-Trimethoxycinnamic Acid from Perilla frutescens in the Forced Swimming Test. Biological and Pharmaceutical Bulletin 2003, 26, 474–480.
- Nitta, M.; Lee, J.K.; Ohnishi, O. Asian perilla Crops and Their Weedy Forms: Their Cultivation, Utilization and Genetic Relationships. Economic Botany 2003, 57, 245–253.
- Paek, J.H.; Shin, K.H.; Kang, Y.H.; Lee, J.Y.; Lim, S.S. Rapid Identification of Aldose Reductase Inhibitory Compounds from Perilla frutescens. BioMed Research International 2013, 679463.
- Wang Z.; Kwon, S.H.; Hwang, S.H.; Kang, Y.H.; Lee, J.Y.; Lim, S.S. Competitive Binding Experiments can Reduce the False Positive Results of Affinity-based Ultrafiltration-HPLC: A Case Study for Identification of Potent Xanthine Oxidase Inhibitors from Perilla frutescens Extract. Journal of Chromatography B 2017, 1048, 30–37.
- Park, S.H.; Paek, J.H.; Shin, D.; Lee, J.Y.; Lim, S.S.; Kang, Y.H. Purple perilla Extracts with α-Asarone Enhance Cholesterol Efflux from Oxidized LDL-exposed Macrophages. International Journal of Molecular Medicine 2015, 35, 957–965.
- Hwang, S.H.; Kwon, S.H.; Kang, Y.H.; Lee, J.Y.; Lim, S.S. Rapid High Performance Liquid Chromatography Determination and Optimization of Extraction Parameters of the α-Asarone Isolated from Perilla frutescens L. Molecules 2017, 22, 270.
- Banno, N.; Akihisa, T.; Tokuda, H.; Yasukawa, K.; Higashihara, H.; Ukiya, M.; Watanabe, K.; Kimura, Y.; Hasegawa, J.I.; Nishino, H. Triterpene Acids from the Leaves of Perilla frutescens and Their Anti-inflammatory and Antitumor-promoting Effects. Bioscience, Biotechnology, and Biochemistry 2004, 68, 85–90.
- Wang, J.W.; Xia, Z.H.; Chu, J.H.; Tan, R.X. Simultaneous Production of Anthocyanin and Triterpenoids in Suspension Cultures of Perilla frutescens. Enzyme and Microbial Technology 2004, 34, 651–656.
- Meng, L.; Lozano, Y.; Bombarda, I.; Gaydou, E.M.; Li, B. Polyphenol Extraction from Eight Perilla frutescens Cultivars. Comptes Rendus Chimie 2009, 12, 602–611.
- Hazra, B.; Biswas, S.; Mandal, N. Antioxidant and Free Radical Scavenging Activity of Spondias pinnata. BMC Complementary and Alternative Medicine 2008, 8, 63.
- Dai, X.; Huang, Q.; Zhou, B.; Gong, Z.; Liu, Z.; Shi, S. Preparative Isolation and Purification of Seven Main Antioxidants from Eucommia ulmoides Oliv. (Du-zhong) Leaves Using HSCCC Guided by DPPH-HPLC Experiment. Food Chemistry 2013, 139, 563–570.
- Shi, S.; Ma, Y.; Zhang, Y.; Liu, L.; Liu, Q.; Peng, M.; Xiong, X. Systematic Separation and Purification of 18 Antioxidants from Pueraria lobate Flower Using HSCCC Target-Guided by DPPH–HPLC Experiment. Separation and Purification Technology 2012, 89, 225–233.
- Sun, L.Q.; Ding, X.P.; Qi, J.; Yu, H.; He, S.A.; Zhang, J.; Ge, H.X.; Yu, B.Y. Antioxidant Anthocyanins Screening Through Spectrum–Effect Relationships and DPPH-HPLC-DAD Analysis on Nine Cultivars of Introduced Rabbiteye Blueberry in China. Food Chemistry 2012, 132, 759–765.
- Yang, F.; Zhang, T.; Tian, G.; Cao, H.; Liu, Q.; Ito, Y. Preparative Isolation and Purification of hydroxyanthraquinones from Rheum officinale Baill by High-Speed Counter-Current Chromatography Using pH-modulated Stepwise Elution. Journal of Chromatography A 1999, 858, 103–107.
- Marston, A.; Hostettmann, K. Developments in the Application of Counter-Current Chromatography to Plant Analysis. Journal of Chromatography A 2006, 1112, 181–194.
- Oliveria, R.R.; Leitão, G.G.; Moraes, M.C.C.; Kaplan, M.A.C. Lopes, D.; Carauta, J.P.P. Gradient Elution for Triterpene Separation from Cecropia lyratiloba Miquel by HSCCC. Journal of Liquid Chromatography and Related Technologies 2005, 28, 1985–1992.
- da Silva, V.P.; Oliveira, R.R.; Figueiredo, M.R. Isolation of Limonoids from Seeds of Carapa guianensis Aublet (Meliaceae) by High-speed Countercurrent Chromatography. Phytochemical Analysis 2009, 20, 77–81.
- Du, Q.; Jerz, G.; Chen, P.; Winterhalter, P. Preparation of Ursane Triterpenoids from Centella asiatica Using High Speed Countercurrent Chromatography with Step-Gradient Elution. Journal of Liquid Chromatography Related Technologies 2004, 27, 2201–2215.
- Su, X.Y.; Wang, Z.Y.; Liu, J.R. In vitro and in vivo Antioxidant Activity of Pinus koraiensis Seed Extract Containing Phenolic Compounds . Food Chemistry 2009, 117, 681–686.
- Winterbourn, C.C.; Metodiewa, D. Reactivity of Biologically Important Thiol Compounds with Superoxide and Hydrogen Peroxide. Free Radical Biology and Medicine 1999, 27, 322–328.
- Hsu, B.; Coupar, I.M.; Ng, K. Antioxidant Activity of Hot Water Extract from the Fruit of the Doum palm, Hyphaene thebaica. Food Chemistry 2006, 98, 317–328.
- Nitha, B.; De, S.; Adhikari, S.K.; Devasagayam, T.P.A. Evaluation of Free Radical Scavenging Activity of Morel Mushroom, Morchella esculenta Mycelia: A Potential Source of Therapeutically Useful Antioxidants. Pharmaceutical Biology 2010, 48, 453–460.
- Oliveira, D.R.; Schaffer, L.F.; Busanello, A.; Barbosa, C.P.; Peroza, L.R.; Freitaas, C.M.; Krum, B.N.; Bressan, G.N.; Boligon, A.A.; Athayde, M.L.; Menezes, I.R.A.; Fachinetto, R. Silymarin has Antioxidant Potential and Changes the Activity of Na+/K+-ATPase and Monoamine Oxidase in vitro. Industrial Crops and Products 2015, 70, 347–355.
- Amarowicz, R.; Pegg, R.B.; Rahimi-Moghaddam, P.; Barl, B.; Weil, J.A. Free-radical Scavenging Capacity and Antioxidant Activity of Selected Plant Species from the Canadian Prairies. Food Chem. 2004, 84, 551–562.
- Wang, Z.; Hwang, S.H.; Lim, S.S. Chemoenzymatically Synthesized Policosanyl Phenolates as Autoxidation Inhibitors. European Journal of Lipid Science and Technology 2015, 117, 300–310.
- Jun, H.I.; Kim, B.T.; Song, G.S.; Kim, Y.S. Structural Characterization of Phenolic Antioxidants from Purple Perilla (Perilla frutescens var. acuta) Leaves. Food Chemistry 2014, 148, 367–372.
- Chen, J.H.; Xia, Z.H.; Tan, R.X. High-performance Liquid Chromatographic Analysis of Bioactive triterpenes in Perilla frutescens. Journal of Pharmaceutical and Biomedical Analysis 2003, 32, 1175–1179.
- Sawabe, A.; Satake, T.; Alzawa, R.; Sakatani, K.; Nishinoto, K.; Ozeki, C.; Hamada, Y.; Komemushi, S. Toward Use of the Leaves of Perilla frutescens (L.) Britton var. Acuta Kuto (Red Perilla) with Japanese Dietary Pickled Plum (umeboshi). Journal of Oleo Science 2006, 55, 413–422.
- Masahiro, T.; Risa, M.; Harutaka, Y.; Kazuhiro, C. Novel Antioxidants Isolated from Perilla frutescens Britton var. crispa (Thunb.). Bioscience, Biotechnology, and Biochemistry 1996, 60, 1093–1095.
- Kalam, S.; Gul, M.Z.; Singh, R.; Ankati, S. Free Radicals: Implications in Etiology of Chronic Diseases and Their Amelioration Through Nutraceuticals. Pharmacologia 2015, 6, 11–20.
- Giese, E.C.; Gascon, J.; Anzelmo, G.; Barbosa, A.M.; Alves da Cunha, M.A.; Dekker, R.F.H. Free-Radical Scavenging Properties and Antioxidant Activities of Botryosphaeran and Some Other β-D-glucans. International Journal of Biological Macromolecules 2015, 72, 125–130.
- Johansen, J.S.; Harris, A.K.; Rychly, D.J.; Ergul, A. Oxidative Stress and the Use of Antioxidants in Diabetes: Linking Basic Science to Clinical Practice. Cardiovascular Diabetology 2005, 4, 5.
- Gliszczyńska-Świgło, A. Antioxidant Activity of Water Soluble Vitamins in the TEAC (Trolox Equivalent Antioxidant Capacity) and the FRAP (Ferric Reducing Antioxidant Power) Assays. Food Chemistry 2006, 96, 131–136.
- Ozgen, M.; Reese, R.N.; Tulio Jr, A.Z.; Scheerens, J.C.; Miller, A.R. Modified 2,2-Azino-Bis-3-Ethylbenzothiazoline-6-Sulfonic Acid (ABTS) Method to Measure Antioxidant Capacity of Selected Small Fruits and Comparison to Ferric Reducing Antioxidant Power (FRAP) and 2,2’-Diphenyl-1-Picrylhydrazyl (DPPH) Methods. Journal of Agricultural and Food Chemistry 2006, 54, 1151–1157.
- Leiss, K.A.; Maltese, F.; Choi, Y.H.; Verpoorte, R.; Klinkhamer, P.G.L. Identification of Chlorogenic Acid as a Resistance Factor for Thrips in Chrysanthemum. Plant Physiology 2009, 150, 1567–1575.
- Jung, H.A.; Park, J.C.; Chung, H.Y.; Kim, J.; Choi, J.S. Antioxidant Flavonoids and Chlorogenic Acid from the Leaves of Eriobotrya japonica. Archives of Pharmacal Research 1999, 22, 213–218.
- Li, H.J.; Luo, Y.G.; He, Z.H.; Zhang, G.L. Chemical Constituents from Lonicera saccata. Chinese Journal of Applied and Environmental Biology 2007, 13, 188–191.
- Sobolev, V.S.; Horn, B.W.; Potter, T.L.; Deyrup, S.T.; Gloer, J.B. Production of Stilbenoids and Phenolic Acids by the Peanut Plant at Early Stages of Growth. Journal of Agricultural and Food Chemistry 2006, 54, 3505–3511.
- Wang, M.; Li, J.; Rangarajan, M.; Shao, Y.; Lavoie, E.J.; Huang, T.C.; Ho, C.T. Antioxidative Phenolic Compounds from Sage (Salvia officinalis). Journal of Agricultural and Food Chemistry 2007, 46, 4869–4873.
- Kim, N.M.; Kim, J.; Chung, H.Y.; Choi, J.S. Isolation of Luteolin 7-O-Rutinoside and Esculetin with Potential Antioxidant Activity from the Aerial Parts of Artemisia montana. Archives of Pharmacal Research 2000, 23, 237–239.
- Machida, K.; Osawa, K. Quantitative Analysis of Methoxylated Flavonoids in iyo (Citrus iyo) Peels by High-Performance Liquid Chromatography. Yakugaku Zasshi 1989, 109, 192–194.
- Cuvelier, M.E.; Richard, H.; Berset, C. Comparison of the Antioxidative Activity of Some Acid-Phenols: Structure-Activity’ Relationship. Bioscience, Biotechnology, and Biochemistry 1992, 56, 324–325.
- Benavente-García, O.; Castillo, J.; Lorenta, J.; Ortuño, A.; Del Rio, J.A. Antioxidant Activity of Phenolics Extracted from Olea europaea L. Leaves. Food Chemistry 2000, 68, 457–462.