ABSTRACT
The crystallization of lipids has important implications for the industrial processing of food products, such as chocolates, margarines, spreads, confectionery, as well as bakery and dairy products. Thus, blends of soft palm mid-fraction (PMF) and dipalmitin (PP) containing PP of 0.5 g/100 g and 1.0 g/100 g were investigated under stabilization time and conditions for controlling and improving the crystallization step of fat during fat modulation. Crystallization results showed by reducing the Avrami parameters tsfc, t1/2, and k, the addition of PP accelerated the crystallization process of PMF. Changes in the crystals morphology observed by polarized light microscopy gave a higher crystallized area and reduced crystals size in the microstructure of the PMF, which was also verified by the increase in melting point and solid fat content (SFC). The addition of PP caused changes in the arrangement of the PMF triacylglycerols (TAGs), by stabilizing the β´stable polymorphic form, thereafter stabilizing the melting profile and the consistency of PMF over time. These results have interesting implications in the fat and oil industry as they allow for the marketing of products with improved crystallization properties and the stabilization of more stable polymorphic forms, expanding the range of applications of slow-crystallizing fats.
Introduction
Palm oil is composed of high- and low-melting-point triacylglycerols (TAGs), resulting in a wide range of melting temperatures and industrial applications. To extend the range of applications for palm oil, it is either chemically or physically modified, and hydrogenation, interesterification, and fractionation are typically employed in the vegetable oil industry for the modification of palm oil. In dry fractionation, the different melting points of TAGs could result in liquid fractions (i.e., olein, super oleins, and top oleins), which are normally used for cooking and salad oils, and hard fractions (stearin and mid-fractions), which are fat sources used for frying, margarines, shortening, and production of special fats. The soft palm mid-fraction (PMF), an example of mid-fractions, is predominantly used for the formulation of cocoa butter equivalents and special confectionery fats.[Citation1]
The crystallization of lipids has important implications in the industrial processing of food products such as chocolates, margarine, spreads, confectionery, as well as bakery and dairy products,[Citation2] whose physical characteristics are significantly dependent on fat crystals. Crystallization studies usually focus on the crystallization of TAGs, which are the major components that are naturally present in fats and oils. However, minor components such as monoacylglycerol (MAG), diacylglycerol (DAG), and phospholipids are also important in determining the quality of the final product as these compounds modulate the entire crystallization process – from nucleation to post-crystallization events – which include polymorphism, driving force, and solid fat content (SFC).[Citation3]
DAGs are partial acylglycerols, which can exist as two isomers: 1,2-DAG and 1,3-DAG. Edible DAGs have attracted a longstanding interest because of their importance in the human diet.[Citation4] Nevertheless, a minor component can affect either the nucleation or the growth of crystals or both parameters simultaneously. Some compounds can stimulate nucleation while slowing down the growth of crystals, or vice versa, while others can stimulate or retard the two specified crystallization events.[Citation5]
A change in the crystallization behavior of lipid systems in fat-based products is a critical issue in the processing of foods, with the aims of preparing tailor-made products, reducing costs, improving quality, and increasing the applicability and stability of different industrial fats.[Citation2] Currently, most studies on fat crystallization focus on the crystallization of TAGs, which is the main functional component of fats. Some recent studies have reported the influence of DAGs on multiple oil and fat matrices, but little is known about the crystallization behavior of fats rich in DAGs and many of these studies have conflicting results.[Citation4] Some studies have claimed that the presence of DAGs led to a negative effect on the crystallization process,[Citation6,Citation7] while others have reported both negative and/or positive effects.[Citation3–Citation5,Citation8] The difference in the mechanistic role of DAGs during fat crystallization has been frequently attributed to three reasons. First, the chemical interaction is sometimes based on the similarities or differences in the chemical composition between DAGs and their fat source lipids base.[Citation3,Citation5] In addition, the position of the DAGs’ fatty acids (positions 1,3 or 1,2),[Citation4,Citation7,Citation9] could occasionally be an influencing factor, along with the concentration of the added or naturally present DAGs.[Citation7,Citation10]
Despite there being many studies on the effects of DAG as minor components, hardly anything is known about the crystallization behavior of pure added DAGs and there are not many studies on this effect in soft PMF. With this background, this study aimed to investigate the effect of adding dipalmitin (PP) on the crystallization of soft PMFs by evaluating the macroscopic (consistency, SFC, and melting point) and microscopic (polarized light microscopy, isothermal crystallization, melting profile, and polymorphic form) properties over time.
Materials and methods
Sample preparation
The soft PMF was supplied by Fuji Oil Company, and PP (99 g/100 g pure) was purchased from Nu-Chek Prep. Inc. (USA). The proportions of PP in soft PMF investigated were 0 g/100 g (PMF), 0.5 g/100g (PMF0.5), and 1.0 g/100 g (PMF1.0). PP (0.5 and 1.0 g/100 g w/w) was dispersed in the melted soft PMF and stirred using a magnetic stirrer at 70 ± 0.5°C until the PP crystals completely melt. The soft PMF in the study was characterized by gas chromatography for fatty acids and TAGs composition[Citation11,Citation12] and high-performance liquid chromatography (HPLC) equipped with a refraction index detector for minor lipids .[Citation13] The PMF in this study naturally contains 6 g/100 g of DAGs, and are composed majorly of the following fatty acids: 1.06 g/100g myristic acid (M), 47.32 g/100 g palmitic acid (P), 4.77 g/100 g stearic acid (St), 38.31 g/100 g oleic acid (O), and 7.41 g/100 g of linoleic acid (L). These fatty acids form the following TAGs: PPP (2.88 g/100 g), MOP (2.95 g/100g), PPSt (22.29 g/100g), POP (32.36 g/100 g), PLP (8.04 g/100 g), POSt (7.67 g/100 g), POO (14.96 g/100 g), POL (4.67 g/100 g), StOO (0.75 g/100 g), OOO (1.28 g/100 g), and OOL (2.15 g/100 g).
SFC and melting point
SFC was determined according to the American Oil Chemistry Society (AOCS) Cd 16b‐93 method[Citation11] with a tempering period for nonstabilized fats, and the readings were recorded serially at 10, 20, 25, 30, 35, 40, 45, and 50°C. The Mq20 NMR Analyzer Bruker (NMR) and high-precision TCON 2000 dry bath (Duratech, Carmel, IN, USA) equipment with a temperature range of 0–70°C were utilized. The melting point was measured by a polynomial equation at the temperature at which the SFC is the same as 4 g/100 g.[Citation14] The results were expressed as a mean of three measurements.
Isothermal crystallization
The samples were melted at 100°C for 15 min and were maintained at 70°C for 1 h in the dry bath to completely erase crystal memories. The increase in SFC during crystallization time was automatically monitored every minute for 180 min at 17.5°C using a Bruker pc120 Minispec NMR spectrometer (Silberstreifen, Rheinstetten, Germany). Isothermal SFC data were fitted to the original Avrami equation (Eq. 1) to assess the kinetics parameters.[Citation15]
where SFC (t) represents the SFC (%) as a function of time, SFCmax (%) represents the asymptotic value of the SFC, k represents the Avrami constant (min−n), which considers the rates of both nucleation and crystal growth, and n represents the Avrami exponent, which is indicative of the crystal growth mechanism. The equation not linearized was used for obtaining the k and n values. To express the magnitude of k and n values, the half-times crystallization (t1/2) was calculated according to Eq. 2[Citation15]:
All results were expressed as a mean of three measurements.
Polarized light microscopy
Samples were melted at 70°C in an oven, and using a capillary tube, a drop of each sample was placed on a glass slide preheated at a controlled temperature (70°C) and covered using a cover glass. Samples were kept in the oven at the analysis temperatures (17.5 and 25°C) for 1 and 7 days. Crystal morphology was evaluated using a polarized light microscope (Olympus, model BX 51, USA) coupled to a digital video camera (Media Cybernetics). The images were captured using Image Pro-Plus software version 7.0 for Windows (Media Cybernetics) using polarized light and amplified up to 40 times. For each glass slide, three visual fields were focused, of which only one was chosen to represent the observed crystals.[Citation3] The evaluation parameter selected for quantitative image analysis was the mean diameter of crystals (Dm), and the percentage of the crystallized area (CA) was measured using Image Pro-Plus software version 7.0 for Windows. The two temperatures were evaluated to obtain any possible relation between isothermal crystallization at 17.5°C and the other analysis at room temperature (25°C).
Consistency
Consistency was determined using a texture analyzer TA-XT Plus (Stable Micro Systems, Surrey, UK). The samples were heated to 70°C for completely melting the fat crystals and were stabilized according to the AOCS method Cd 16b-93.[Citation11] The test was performed at 25°C. The probes used were Plexiglas® with a 45° cone having no truncated tips. The penetration depth was 10 mm, the probe velocity was 2 mm/s, and the time was 5 s. The compression force was expressed as gram force (gF). The compression force was converted to yield value (YV), according to Haighton,[Citation16] by using the following equation (Eq. 3):
where YV represents the yield value in Pa, and K is a constant that depends on the cone angle. W represents the compression force in gF, and p represents the penetration depth in units of 0.1 mm. For a cone of 45°, YV was 4.700. The results were expressed as a mean of four experiments.
Thermal behavior
Thermal behavior was analyzed by differential scanning calorimetry (DSC) using a DSC system (Model Q2000; TA Instruments, New Castle, DE, USA), according to the AOCS Cj 1-94 method[Citation11] with some modifications. The conditions for crystallization were as follows: in the first step, the initial temperature was maintained at 80°C for 5 min; in the second step, the temperature was decreased to −40°C at a rate of 2°C min−1; and in the third step, the temperature was maintained at −40°C for 5 min. The conditions for melting were as follows: 25°C for 5 min, melting to 60°C at a rate of 5°C min−1, and maintaining the temperature at 60°C for 5 min. Approximately 10 mg of the melted sample was weighed, and an empty sealed aluminum pan was used as the reference. For investigating the melting behavior, the samples were analyzed with time after 0, 1, 7, 14, and 21 days of stabilization at 25°C. The equipment was calibrated using indium.
X-ray diffraction
X-ray diffraction (XRD) analyses of the samples were performed according to the AOCS method Cj 2-95 a[Citation11] by using a Philips PW 1710 diffractometer (PANalytical, Almelo, the Netherlands) with Bragg–Brentano (θ:2θ) geometry and CuKα radiation (λ = 1.51418 Å; tension, 40 kV; and current, 30 mA). The geometry used resulted in the diffraction of the X-ray beam by the sample, which passed through a graphite monochromator crystal located just before the detector. All measurements were obtained with short spacing at steps of 0.03°C in 2θ and an acquisition time of 2 s, with scans from 15° to 30°, and at 25°C.[Citation17] The samples were also prepared after 0, 1, 7, 14, and 21 days of stabilization at 25°C.
Statistical analyses
Statistical analyses of the melting point, SFC, crystal diameter, crystallized area, and isothermal parameters were performed by the analysis of variance. Significant differences were determined by Tukey’s test at p < 0.05 using Statistica 8.0 (Statsoft, USA).
Results and discussion
SFC and melting point
SFC is the percentage of lipid in the solid form at a given temperature, and these values are responsible for many characteristics in lipid-based food, including their appearance, ease of packaging, spreadability, oiling-out, and organoleptic properties.[Citation18] The SFC was generally suppressed by the presence of diglycerides.[Citation7] In , no significant differences were observed among the SFC profiles of the PMF, PMF0.5, and PMF1.0 samples, which exhibited a behavior similar to those observed in Saberi et al.[Citation10] This could be a consequence of the lower amounts of DAG used, because the reduction observed for Siew and Ng[Citation7] was observed at a concentration above 2 g/100 g of different DAGs in palm oil (PP, OO, and PO).
Figure 1. Solid fat content (g/100 g) of soft PMF, PMF0.5 with 0.5 g/100 g of PP, and PMF1.0 with 1 g/100g of PP, at various temperatures.
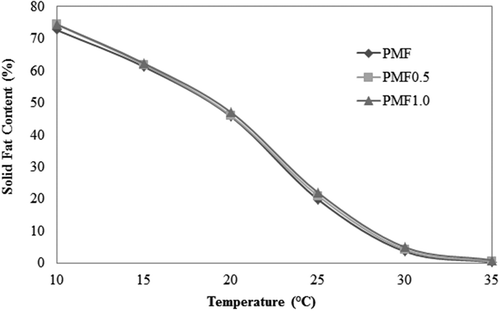
The melting point of a fat or oil is an important parameter as it determines its physical state at any given temperature and it is one of the specifications normally required in the vegetable oil industry.[Citation9] Fats with a melting point and high SFC values above body temperature (~34°C) can cause a waxy taste when eating.[Citation19] The addition of PP raises the melting points of the samples, which are equal to 24.07°C, 24.95°C, and 25.90°C for PMF, PMF0.5, and PMF1.0, respectively. This result suggests that the PMF with PP can be used for food application without sensorial problems and that PP facilitated the formation of more stable crystals with higher melting points, which could be due to transition to more stable polymorphic forms. The same result was obtained by Siew and Ng[Citation9] in palm oil when the added DAG was 1,3-PP (even though using 1,2-PP produced the opposite effect), thus indicating the potential efficacy of 1,3-PP in improving the melting point of PMF.
Isothermal crystallization
Crystallization kinetics is indicative of the mechanism of nucleation and crystal growth. It is well known that palm oil has a slow crystallization behavior, due to its TAG composition and to the presence of DAGs (5–8%).[Citation20,Citation21] However, as shown in and , the addition of PP accelerated the induction time and significantly increased the maximum solid content of PMF (p<0.05). The induction time of a sample is the time required to detect the formation of the first nucleus in a subcooled or supersaturated system. This period includes the actual time needed for nucleation, as well as that required for detecting crystallization using an experimental technique. Generally, the induction time is assumed to increase with rising crystallization temperature and falling melting point of the sample.[Citation15] We observed that PMF0.5 and PMF1.0 exhibited a two-step crystallization process at 17.5°C. Two-step crystallization is usually related to the nucleation of two different crystalline polymorphic forms, which generates curves that equate to an intermediate level, before increasing again to a second level. First, the SFC increases without an appreciable induction time and the elevated value remains constant for some time, after which it increases dramatically. Next, the SFC reaches the second plateau and the presence of a plateau is attributed to a polymorphic transition in the sample, changing from less to more stable polymorphs.[Citation22] Therefore, the addition of PP influences the primary nucleation and speeds up the formation of stable crystal nuclei in PMF.
Table 1. The Avrami parameters of isothermal crystallization from soft palm mid-fraction (PMF) and their blends with dipalmitin (PP).
Figure 2. Isothermal crystallization at 17.5°C of pure soft PMF, PMF0.5 with 0.5 g/100 g of PP, and PMF1.0 with 1 g/100 g of PP.
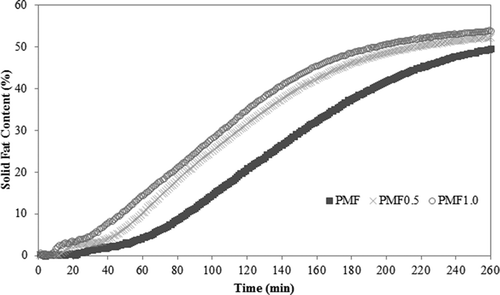
Temperature oscillations during crystal formation directly affect the crystallization kinetics. In , the analysis of the Avrami parameters showed that the crystallization rate (k) was significantly increased by the addition of PP. This result corroborates the theory that when the acyl group of a minor lipid is similar to those present in fats with high melting points, the crystallization process is accelerated because these DAGs could act as seed crystals and co-crystallize with TAGs.[Citation4] In this study, PMF exhibited the value of n = 2.78, which indicates a sporadic nucleation rate and the first crystal grows in a more two-dimensional manner. PMF0.5 shows the value of n = 2.02, suggesting that the first crystal grows bi-dimensionally. Meanwhile, PMF1.0 had a value of n = 1.82, which indicates instantaneous nucleation and one-dimensional crystal growth. The shape and crystalline growth data obtained using the Avrami exponent can be confirmed by the microstructure analysis and they agree with the results of Campos.[Citation15]
To ensure the quality of the data shown by the Avrami parameters, we calculated the t1/2 of the samples, which is expressed in minutes and more accurately reflects the magnitude of the k and n values. The half-time represents the time at which half of the sample crystallizes. This parameter is very important when one intends to apply the fat because it shows not only the beginning of the crystallization or SFCmax at the end but also how the sample actually works in practical terms. We observed significant differences between the t1/2 value of PMF and those of PMF0.5 and PMF1.0, inferring a change in the crystal growth (). The crystallization half-times were significantly lower for PMF0.5 and PMF1.0. Therefore, the results for the crystallization kinetics can be interpreted as the effect of PP’s compatibility with other components of PMF such as PPP, PPSt, MOP, POP, and PLP. Such interaction leads to a faster crystallization of TAGs, which is responsible for the crystallization induction of palm-based fats.[Citation3]
Microstructure and Consistency
Lipid composition and the conditions of crystallization affect crystal formation, potentially influencing the creation of different polymorphic forms and crystalline morphologies. The crystals aggregate as large structures to form a crystalline lattice, which characterizes the microstructure of fats.[Citation8] shows the polarized light microscopy images of the lipid systems, while lists the results of Dm and the total CA, both of which are parameters useful for studying the crystallization of lipid systems.
Table 2. Mean crystal diameter (Dm) and total crystallized area (CA) of soft palm mid-fraction (PMF) with dipalmitin (PP).
Figure 3. Polarized light micrograph of the crystal structure obtained under static isothermal crystallization at 17.5°C, for 3 h (PMF [A],PMF0.5[B], PMF1.0[C]), and at 25° C for 1 day (PMF [D], PMF0.5[F], PMF 1.0[H]) and 7 days (PMF [E], PMF0.5 [G], PMF1.0 [I]), magnification of 40x (scale bar = 50 μm).
![Figure 3. Polarized light micrograph of the crystal structure obtained under static isothermal crystallization at 17.5°C, for 3 h (PMF [A],PMF0.5[B], PMF1.0[C]), and at 25° C for 1 day (PMF [D], PMF0.5[F], PMF 1.0[H]) and 7 days (PMF [E], PMF0.5 [G], PMF1.0 [I]), magnification of 40x (scale bar = 50 μm).](/cms/asset/2202dc6a-f73a-4d9c-9adb-ba8e356c21a0/ljfp_a_1318290_f0003_b.gif)
In –, the microstructure is associated with the Avrami parameters in the following manner: the CA value significantly increased with the addition of PP because of the higher k, which was confirmed by the values shown in . However, it was not possible to verify a change in the type of crystal formed, as described by the Avrami parameter at 17.5°C at the time of analysis.
In –, we noticed that the addition of PP drastically affected the crystalline morphology of PMF. Substantial changes were observed over time at 25°C. As shown in , we observed a low crystal density because of the presence of large crystals in PMF. After 7 days, the size of these crystals became even larger (). In contrast, we observed that PMF0.5 and PMF1.0 (–) had a high crystalline density owing to tiny needle-like crystals; however, these crystals also became larger over time. At the early stages of food processing, the relative speeds of nucleation and crystal growth determine the distribution, shape, and size of the crystals, parameters that are directly associated with the end-product characteristics such as consistency and hardness. However, various post-crystallization phenomena can occur during storage, potentially affecting the properties and stability of foods.[Citation2]
The palm oil upon storage naturally presents an increase in SFC and this may lead to post-hardening. Slow post-hardening causes primary crystal bonds, which results in a hard product.[Citation20] It was evident that the consistency of PMF, PMF0.5, and PMF1.0 increased over time. However, in , we observed that the addition of PP resulted in lower YVs. The YV significantly increased up to the 14th day for all the samples. After 21 days of storage, we only noticed a difference in the YV of PMF1.0.
Table 3. Consistency expressed as yield value (Pa), measured at 25°C, of blends of soft palm mid-fraction (PMF) and dipalmitin (PP).
Some studies have attributed the post-hardening of palm oil and its fraction due to the formation of granular crystal formation in palm oil when a slow k occurred tending to form β crystals and crystalline agglomerates.[Citation21] The PP in this case acts as a seed driving the crystallization process to small crystals without clusters formation as observed in the microscopies.
The macroscopic properties of fat crystal networks such as mechanical strength (consistency or hardening) depend on the SFC, morphology, and interaction of crystals. Therefore, these results can be explained by associating them with phenomena such as large crystal maturation (Ostwald ripening) and/or solid bond formation between the TAG and the solid/liquid phases (sintering). In this context, the addition of PP mitigates the negative effects of these phenomena.
Crystallization mechanisms: thermal and polymorphic behavior
shows the crystallization and melting behavior of PMF, PMF0.5, and PMF1.0. The crystallization profile is characterized by the initial formation of the fat crystal, represented by the rearrangement of the highly saturated TAG molecules, and the end of the crystallization process, as indicated by the aggregation and compression of these molecules.[Citation23] In , we observed that adding PP accelerated the first crystallization event and reduced the crystallization energy slightly. The first crystallization event corresponded to high melting point TAGs such PPP and PPSt, whereas the second event corresponded to the di-saturated TAGs in PMF such as POP, PLP, and POSt. Therefore, PP influences the crystallization of tri-saturated TAGs more strongly compared with that of di-saturated TAGs. A high melting DAG such as PP potentially acts as a seed, crystallizing before TAGs, and subsequently inducing nucleation and crystal growth,[Citation24] as observed in . Its high thermal stability (68.31°C) was close to that observed for 1,2-PP (50–67°C) by Wright and Marangoni.[Citation8]
Figure 4. Thermal behavior of the PMF samples, PMF, PMF0.5, and PMF1.0; (A) crystallization curves; (B) melting curves on zero time; (C) melting curves after 1 day; (D) melting curve of pure dipalmitin; (E) melting curves after 7 days; (F) melting curves after 14 days; (G) melting curves after 21 days. See for an explanation of abbreviations.
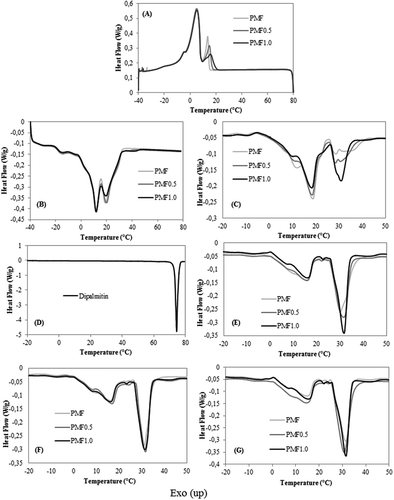
shows the melting phenomenon at time zero and we observed a few differences between the samples, resulting from the short stabilization time. The melting behavior of a non-stabilized PMF was used as a measurement parameter and the stabilizing effect of the PP addition on PMF was confirmed. In , we observed some changes in the melting events after a one-day storage at 25°C. All samples exhibited two distinct melting events, which resulted from polymorphic transitions. The addition of PP decreased the intensity of the first peak but increased the intensity of the second peak. This change was greater in PMF1.0, which indicates that PP accelerates the formation of stable polymorphs.
As shown in , the second melting events of PMF0.5 and PMF1.0 were much more intense than that of PMF after being stored for 7 days. In other words, PMF1.0 reached its maximum stability in 7 days as all the samples exhibited practically the same melting profile after prolonged storage for 14 and 21 days ( and ). The accelerated stability probably is a response to the high amount of PP that works as nuclei and enhanced the crystallization process.
In addition to the calorimetry results, –) shows the short spacing patterns of PMF, PMF0.5, and PMF1.0 as analyzed via XRD at 25°C. PMF crystallizes predominantly in the β′ polymorphic form regardless of the storage time, which is expected for palm oil and its fractions[Citation25] owing to the presence of POO and other asymmetrical types of TAGs that tend to produce the β´-form. The β′ form, having a small crystal size, is a desirable polymorphic form for margarine and shortening stock.[Citation18]
Figure 5. Diffractograms of pure soft PMF (A), PMF0.5 (B), and PMF1.0 (C) after 1, 7, 14, and 21 days at 25°C. See for an explanation of abbreviations.
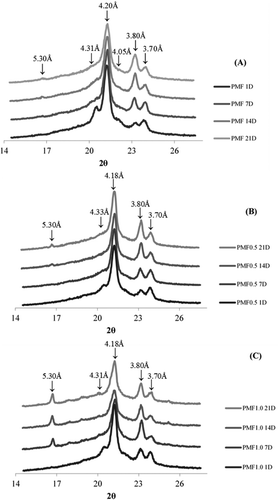
Notably, the addition of PP in this fraction facilitates the formation of the β´ stable polymorph and delays the onset of the β-form. Most studies have reported similar results regarding the delay in the polymorphic transition of the β’→β form. Hernquist et al.[Citation22] reported that DAGs stabilize metastable polymorphs, resulting in a delay of the polymorphic transition predominantly by the 1,2-DAG. Currently, this delay has been attributed to the preferable packing of 1,2-DAG in an orthorhombic formation, and the DAG concentration is inversely proportional to the onset time of the β-form. In the present study, PMF0.5 and PMF1.0 showed intense peaks in the regions of 4.20 and 3.80 Å after 21 days, indicating a stable β’-form. As seen in , PMF1.0 shows diffraction patterns that correspond to the β’-form, although the peak appears at 5.30 Å.
On Day1, PMF has diffraction peaks at 4.31 and 4.05 Å. However, the 4.05 Å peak was not observed in PMF0.5 and PMF1.0 while the 4.31 Å peak was less evident. These peaks disappeared from PMF with an increase in storage time, thus suggesting that the presence of PP accelerates the formation of the more stable β’ polymorph. These results corroborate the thermal behavior of the samples over time.
The kinetics of the polymorphic TAG transitions are influenced by the presence of minor lipids and these precursor crystalline materials can act as nuclei to influence the type of TAG crystal formed.[Citation26] In this context, we observed a higher polymorphic stability of the samples added with 0.5 and 1.0 (g/100 g) of PP. The addition of foreign materials, called templates or additives, during the crystallization of lipids is widely used to modify the heterogeneous nucleation behavior. Sato et al.[Citation26] reported that the conditions required for the successful modification of lipid crystal nucleation via the use of template/additive are as follows: similarity in molecular shape, thermal stability, and optimal supercooling.
PP affects the solidification properties of soft PMF owing to a similarity in molecular shape between PP and PMF’s TAGs, especially the presence of the palmitic acid moiety as suggested by Smith et al.[Citation24] It is well known that when several phases coexist in solution, the only stable phase is the one that has the lowest solubility. In this case, the decrease in PP’s solubility in the liquid phase is a result of the saturated long-chain palmitic acids. Thus, PP acts as a template for clusters and is a promoter of critical nuclei. The high thermal stability of PP is also a contributing factor. In addition, the temperatures used in this study induced the nucleation process and the effects of PP as a template could be maximized and visualized via the crystallization kinetics, crystal network structure, as well as the thermal and polymorphic behaviors of PMF.
Conclusion
This study showed that the addition of PP changes the arrangement form of soft PMF’s TAGs, causing drastic changes in the crystal lattice structure and the solidification properties of soft PMF. Such structural changes in turn accelerate the crystallization process owing to a faster stabilization of the polymorph of interest. The changes occurring during the crystallization process of the PMF/PP blend can be summarized as follows: (i) PP is highly soluble in liquid PMF but its solubility decreases with supercooling owing to the presence of long-chain palmitic acid, (ii) PP crystallizes prior to the PMF’s TAGs and acts as a template for PMF crystals, (iii) PP accelerates the rearrangement of the PMF’s TAGs from the melted state and assists in the formation of stable β’ crystals. Such changes in the crystallization process of soft PMF represent a highly viable option, in terms of economy and technology, for modulating the crystallization properties of special fats in the food industry.
LJFP_A_1318290_Supplemtary_Material.docx
Download MS Word (17.2 KB)Funding
The authors are grateful for the financial support received from Fundação de Amparo à Pesquisa do Estado de São Paulo (FAPESP—Proc. 2009/53006-0 and Proc. 2012/10871-6) and Conselho Nacional de Pesquisa (CNPq).
Supplemental data
Supplemental data for this article can be accessed on the publisher’s website.
Additional information
Funding
References
- Kellens, M,; Gibon, V.; Hendrix, M.; De Greyt W. Palm Oil Fractionation. European Journal of Lipid Science and Technology 2007, 109, 336–349.
- Ribeiro, A.P.B.; Masuchi, M.H.; Miyasaki, E.K.; Domingues, M.A.F.; Stroppa, V.L.Z.; Oliveira, G.M.; Kieckbusch, T.G. Crystallization Modifiers in Lipid Systems. Journal of Food Science and Technology 2015.
- Silva, R.C.; Soares, F.A.S.D.M.; Maruyama, J.M.; Dagostinho, N.R.; Silva, Y.A.; Calligaris, G.A.; Ribeiro, A.P.B.; Cardoso, L.P.; Gioielli, L.A. Effect of Diacylglycerol Addition on Crystallization Properties of Pure Triacylglycerols. Food Research International 2014, 55,436–444.
- Saitou, K.; Mitsui, Y.; Shimizu, M.; Kudo, N.; Katsuragi, Y.; Sato, K. Crystallization Behavior of Diacylglycerol-Rich Oils Produced from Rapeseed Oil. Journal of American Oil Chemistry Society 2012, 89, 1231–1239.
- Foubert, I.; Vanhoutte, B.; Dewettinck, K. Temperature and Concentration Dependent Effect of Partial Glycerides on Milk Fat Crystallization. European Journal of Lipid Science and Technology 2004, 106, 531–539.
- De Oliveira IF, Grimaldi R, Gonçalves LAG. Effect of Diacylglycerols on Crystallization of Palm Oil (Elaeis guineensis). European Journal of Lipid Science and Technology 2014, 116, 904–909.
- Siew, W.L.; Ng, W.L. Influence of Diglycerides on Crystallisation of Palm Oil. Journal of the Science of Food and Agriculture 1999, 79, 722–726.
- Wright, A.J.; Marangoni, A.G. Effect of DAG on Milk fat TAG Crystallization. Journal of American Oil Chemistry Society 2002, 79, 395–402.
- Siew, W.; Ng, W. Differential Scanning Thermograms of Palm Oil Triglycerides in the Presence of Diglycerides. Journal of Oil Palm Research 2000, 12, 1–7.
- Saberi, A.H.; Tan, C.P.; Lai, O.M. Phase Behavior of Palm Oil in Blends with Palm-based Diacylglycerol. Journal of American Oil Chemistry Society 2011, 88, 1857–1865.
- American Oil Chemists’ Society (AOCS). Official Methods and Recommended Practices of the American Oil Chemists’ Society, 5th edn.; AOCS, Champaign, 2009.
- Filho, N.R.A.; Mendes, O.L.; Lanças, F.M. Computer Prediction of Triacylglycerol Composition of Vegetable Oils by HRGC. Chromatographia 1995, 40, 557–562.
- Márquez-Ruiz, G.; Jorge, N.; Martín-Polvillo, M.; Dobarganes, M.C. Rapid, Quantitative Determination of Polar Compounds in Fats and Oils by Solid-Phase Extraction and Size-Exclusion Chromatography Using Monoestearin as Internal Standard. Journal of Chromatography 1996, 749, 55–60.
- Karabulut, I.; Turan, S.; Ergin, G. Effects of Chemical Interesterification on Solid Fat Content and Slip Melting Point of Fat/Oil Blends. European Food Research and Technology 2004, 218, 224–229.
- Campos, R. Experimental Methodology. In Fat Crystal Network; Marangoni, A.G.; Ed.; Marcel Dek: New York, 2005; p. 267–349.
- Haighton, A.J. The Measurement of the Hardness of Margarine and Fats with Cone Penetrometers. Journal of American Oil Chemistry Society 1959, 36, 345–348.
- Yap, P.; DeMan, J.; DeMan, L.; Polymorphism of Palm Oil and Palm Oil Products. Journal of American Oil Chemistry Society 1989, 66(5), 693–697.
- Yu, D.; Qi, X.; Ren, Y.; Wang, W.; Sun, L.; Xu, D.; Zhang, H.; Hu, L.; Jiang, L.; Elfalleh, W. Thermal and Crystal Characteristics of Enzymatically Interesterified Fats of Fatty Acid-Balanced Oil and Fully Hydrogenated Soybean Oil in Supercritical CO 2 System. International Journal of Food Properties 2016, 1–11.
- Torbica, A.; Jambrec, D.; Tomic, J.; Pajin, B.; Petrovic, J.; Kravic, S.; Lončarević, I. Solid fat content, Pre-crystallization Conditions, and Sensory Quality of Chocolate with Addition of Cocoa Butter Analogues. International Journal of Food Properties 2015, 19, 1029–1043.
- Aini, I.N.; Miskandar, M.S. Utilization of Palm Oil and Palm Products in Shortenings and Margarines. European Journal of Lipid Science and Technology 2007, 109, 422–432.
- Tanaka, L.; Miura, S.; Yoshioka, T. Formation of granular crystals in margarine with excess amount of palm oil. Journal of American Oil Chemistry Society 2007, 84, 421–426.
- Hernqvist, L.; Herslof, B.; Larsson, K.; Podlahaa, O. Polymorphism of Rapeseed Oil with a Low Content of Erucic Acid and Possibilities to Stabilise the β-crystal Form in Fats. Journal of the Science of Food and Agriculture 1981, 1197–1202.
- Tan, C.P.; Che Man, Y.B. Differential Scanning Calorimetric Analysis of Edible Oils: Comparison of Thermal Properties and Chemical Composition. Journal of American Oil Chemistry Society 2000, 77, 143–155.
- Smith, K.W.; Bhaggan, K.; Talbot, G.; Van Malssen, K.F. Crystallization of fats: Influence of minor components and additives. Journal of American Oil Chemistry Society 2011, 88, 1085–1101.
- Braipson-Danthine, S.; Gibon, V. Comparative Analysis of Triacylglycerol Composition, Melting Properties and Polymorphic Behavior of Palm Oil and Fractions. European Journal of Lipid Science and Technology 2007, 109, 359–372.
- Sato, K.; Bayés-García, L.; Calvet, T.; Cuevas-Diarte, M.À.; Ueno, S. External Factors Affecting Polymorphic Crystallization of Lipids. European Journal of Lipid Science and Technology 2013, 115, 1224–1238.