ABSTRACT
A 66.3 kDa lipoxygenase (LOX) with optimum pH of 8.0 and temperature of 35 °C was purified from Sesamum indicum. Fe2+and Ca2+ could stimulate the enzyme activity by 160 and 141%, respectively, while Co2+ highly reduced it to 20%. The LOX activity was decreased at 1.5 mM of cetyltrimethylammonium bromide (CTAB) and β-mercapto ethanol, and 2.5 mM of phenylmethylsulfonyl fluoride (PMSF) and ethylenediaminetetraacetic acid (EDTA). The enzyme showed Km = 1.01 μM and Vmax = 0.054 μmol.min−1 toward linoleic acid as the substrate. The IC50 values for LOX inhibitors including potassium cyanide (KCN) (270 µM), β-carotene (48 µM), and ascorbic acid (132 µM) were determined.
Introduction
Lipoxygenases (LOXs) are non-heme, non-sulphur-iron dioxygenases that incorporate molecular oxygen into polyunsaturated fatty acids (PUFAs) containing one or more (1Z,4Z)-pentadiene moieties to form 9- or 13-hydroperoxy octadecadienoic acid. In simple terms, it catalyses the oxidation of PUFA to form a peroxide of the acid.[Citation1,Citation2] These hydroperoxide products are a highly reactive species. The existence of this enzyme in animals and plants has been recognized for more than five decades.[Citation3] LOX did not require a metal or other prosthetic group.[Citation4] LOX from soybean has been studied in detail, because this enzyme is abundant in various seeds and is easy to purify.[Citation5,Citation6] A number of different physiological roles have been postulated for plant LOXs. Recent studies suggest that they may be involved in plant physiology related to growth and development, such as pest resistance, senescence, pathogen defense, biotic and abiotic stress, and responses to wounding.[Citation3,Citation6,Citation7] LOXs also play an important role in the production of regulatory molecules, including jasmonic acid and traumatin in plants.[Citation2]The subcellular localization of LOX in different plant tissues in many studies has been examined. Most of the evidences suggest that LOX is predominantly a soluble, cytoplasmic enzyme.[Citation3] LOX not only has food-related applications in bread making and aroma production, but also has negative implications for colour, off-flavour, and antioxidant status of plant-based foods.[Citation8] Sesamum indicum seeds have been employed in food factories and sesame oil is a very useful substance in the food and pharmaceutical industries because of the high lipid (44–58 g/100 g dry-solids) and protein content (18–25 g/100 g dry-solids).[Citation9–Citation12]
There are natural antioxidants such as sesamol, sesamolin, and gammatocopherol in sesame oil that prevent cells from damages caused by free radicals. Lignans, such as sesamin, asarinin, and sesamolin, have been found in the sesame seeds and possess health-promoting effects.[Citation13] The main objective of this work was to isolate and characterize LOX from Sesamum indicum. The LOX of sesame has not been studied in terms of either its isolation or its biochemical characterization to date.
Materials and methods
Sesame seeds were prepared from Mashhad grocery store (Mashhad Iran). Linoleic acid, ethylenediamintetraacetic acid (EDTA), and phenylmethylsulfonyl fluoride (PMSF) was purchased from Sigma; Triton X-100, β-carotene, 1, 10-phenanthroline, cetyltrimethylammonium bromide (CTAB) were purchased from Merck (Darmstadt, Germany). Sephadex G-100, fast flow Q-Sepharose was bought from GE Healthcare Life Sciences (Noushdarou Co. Ltd. Tehran, Iran). Ultrafiltration membrane with 30 kDa cutoff was prepared from Millipore (Life Sciences/Biotech, Billerica, Massachusetts, USA).
Preparation of enzyme extract
A total of 100 g of sesame was blended by a milling process. The seed flour was mixed with 300 ml of 50 mM sodium phosphate buffer, pH 7.5. The crude homogenate was subjected to mechanical stirring at 4 ºC for 1 h sonicated using five pulses (30 S each) of a sonicator (Qsonica, LLC series XL-2000, USA), set at power setting 5 W. Later, the homogenate was filtered through four layers of cheesclothes. The resulting suspension was centrifuged at 7,000 × g for 20 min. The pellet was discarded and the supernatant further treated with solid ammonium sulphate up to 60% of saturation and then the inactive supernatant eliminated by centrifugation at 7,000 × g for 20 min. The resulting precipitate was resuspended in a minimal amount of sodium phosphate buffer solution (50 mM pH 7.5) dialyzed twice against the same buffer for 20 h at 4 ºC. The dialyzed extract was assayed for protein content by Bradford’s method. Bovine serum albumin (BSA) was used as the standard.
Gel-filtration and ion-exchange chromatography
The sample was concentrated by an ultrafiltration membrane with cutoff of 30 kDa and the 0.5 ml sample was loaded on gel filtration on a Sephadex G-100 (1 × 100 cm) column equilibrated with 20 mM sodium phosphate buffer at pH 7.5 (equilibrium buffer). Chromatography was performed at a flow rate of 0.25 ml.min−1. Protein elution was monitored by absorbance measurement at 280 nm using an Optizen 3220 UV spectrophotometer (Daejeon, Korea). The active fraction was collected and dialyzed against 20 mM Tris-HCL buffer, pH 8.8. Anion-exchange column (Q-Sepharose, 2 × 5 cm) was used for fractionation. The column was equilibrated with Tris-HCL buffer (20 mM pH 8.8) and then the sample was loaded on the Q-Sepharose column. A linear gradient of NaCl (0.1–1.0 M) in 20 mM Tris-HCL pH 8.8 was used for elution at a flow rate of 1 ml.min−1. The fraction containing protein was monitored based on the absorbance and enzyme activity. The samples showing enzymatic activity were collected for further analyses.
Enzyme assay
The assay solution consisted of 50 μl linoleic acid (at 16 μM), 50 μl methylene blue (at 100 μM), and 300 μl Tris-HCL buffer solution (50 mM pH 8.0). The reaction was initiated by adding 100 μl enzyme solution to the reaction mixture. A control solution containing all the components minus enzyme preparation was used. LOX activity was measured according to Ikuo[Citation14] with a slight modification. Briefly, the decrease in absorbance at 660 nm over 5 min at 25 ºC was accomplished for the enzyme assay. One unit of enzyme activity was considered, the amount of enzyme that hydrolyses 1 μmol of linoleic acid to hydroperoxide over 1 min at 25 ºC.
SDS-PAGE electrophoresis
Sodium dodecyl sulfate polyacrylamide gel electrophoresis (SDS-PAGE) was performed on 5% (w/v) stacking gel and 10% (w/v) separating gel. The molecular weight of the purified LOX was estimated from the logarithm of standard molecular weight versus relative mobility (Rf). After running the gel, staining was carried out with Coomassie Brilliant Blue R-250 dissolved in 40% methanol and 10% glacial acetic acid.
Effect of pH on LOX activity and stability
The pH optimum of the activity of isolated sesame LOX was determined spectrometrically in the range of 3.0–10.0. The buffer systems were citrate phosphate buffer (50 mM, pH 3.5–5.5), sodium phosphate buffer (50 mM, pH 6.0–7.5), and Tris-HCL (50 mM, pH 8.0–10.0). The maximum activity of LOX was considered as 100% and the percentage of relative activity was plotted against different pH values. In the pH stability assay, the enzyme solution was incubated in each buffer for 30 min at 25 ºC, and LOX activity assayed for in the presence of linoleic acid. The buffer system for pH 3.5–10.0 was that used for the pH activity study.
Temperature activity and stability
To determine the optimum temperature for enzyme activity, LOX assay was carried out at 20 ºC–70 ºC in Tris-HCL buffer (50 mM, pH 8.0) with 5 ºC intervals. To evaluate thermal stability, the enzyme solution was incubated in a water bath from 20 ºC to 70 ºC for 30 min and then the mixture reaction was kept at room temperature for 10 min; eventually the residual LOX activity was measured. Experiments were carried out in triplicates.
To determine half-life (t1/2), an enzyme solution containing 160 U.mg−1 was incubated up to 150 min in optimal conditions at temperatures of 25 ºC, 30 ºC, 35 ºC, 40 ºC, and 45 ºC. For each 30-min interval, 20 μl of the sample was taken to examine the residual activity. The amount of activity at the onset of assay was considered as 100%. Finally, the results of residual activity were depicted against incubation time. The inactivation constant (Ki) as a first-order inactivation rate constant was calculated through the measurement of log percentage of the residual activity versus various temperatures. The half-life was calculated using the following relationship:[Citation11]
Inactivation energy (Eai) for LOX-catalysed reaction using linoleic acid was estimated via the Arrhenius plot. For this reason,—Log ki against T−1(inversion of absolute temperature in K) was plotted by which the Eai was calculated using the following equation:[Citation11]
where R is the gas constant (K cal−1 mol−1 = 1.985).
Effect of chemical agents on LOX enzyme
To determine the influence of chelating agents on LOX activity, EDTA, and 1, 10-phenanthroline (at 0.001-2.5 mM) was selected. The enzyme activity was carried out at optimal pH 8.0, 50 mM Tris-HCL buffer. The effect of PMSF (at final concentrations of 0.001, 0.01, 0.1, 0.5, 1.0, 1.5, and 2.5 mM) as a serine modifier agent on the enzyme was examined. In addition, β-mercaptoethanol (at final concentrations of 0.01, 0.1, 0.25, 0.5, 1.0, 1.5, and 2.0 mM) on LOX activity was investigated. Each enzyme–reagent mixture was kept at ambient temperature for 10 min. Eventually, the amount of enzyme activity was estimated under standard conditions assay. A sample without the chemical reagents mentioned above was used as the control.
Effect of metal ions on enzyme activity
To evaluate the impact of metal ions on enzyme reaction, CaCl2, FeCl2, NaCl, MgCl2, ZnCl2, CoCl2, and MnCl2 were selected. The applied concentrations at which these ions were prepared in 50 mM Tris-HCL buffer at pH 8.0 were 0.1, 0.01, and 1.0 mM. Each mixture reaction was incubated at room temperature for 10 min and finally the activity was measured. A mixture assay without metal ions was considered as 100% activity.
Effects of inhibitors on LOX activity
The effects of some compounds on LOX activity were tested. Potassium cyanide (KCN), ascorbic acid, and β-carotene were selected to examine their potential inhibitors on LOX. Various concentrations of KCN (0.1, 0.5, 5.0, and 10.0 mM), ascorbic acid (0.01, 0.1, and 0.5 mM), and β-carotene (0.001, 0.01, and 0.1 mM) were prepared. One hundred microliter of the enzyme (160 U.mg−1) and 50 µl of inhibitor solution in 250 µl of Tris-HCL buffer (50 mM, pH 8.0) were mixed together. The reaction was incubated at room temperature for 10 min and then 50 µl of methylene blue and 50 µl of linoleic acid were added. The amount of LOX activity was evaluated under standard conditions. In control, the same volume of Tris-HCL buffer (50 mM, pH 8.0) was used instead of the inhibitor. The percentage of LOX inhibition was calculated by the following equation:
where ΔA is the differential absorbance of 660 nm. By plotting the percent of LOX inhibition versus inhibition concentration, the IC50 values were determined.
Kinetic measurement
LOX kinetic parameters (Km and Vmax) were calculated from the change in the absorbance at 660 nm.[Citation14] The data were analysed using the Michaelis–Menten equation and the Lineweaver–Burk plot. Using linoleic acid as the substrate in the range of 4.0–32.0 μM, the km value was determined under standard conditions.
Results
The sesame LOX enzyme was purified after extraction from sesame seeds by a two-chromatographic step: first, gel filtration of the extract using sodium phosphate buffer (20 mM pH 7.5) showed a sharp peak with the highest activity in the 68th fraction (). In the next step, ion-exchange was used and fractions eluted off the column by a linear gradient of 0.1–1.0 M NaCl in 20 mM Tris-HCL pH 8.8 (). The most active fraction was obtained at 0.3 M NaCl. The active fractions were collected and dialyzed against 50 mM Tris-HCL pH 8.0.
Figure 1. Chromatography of LOX on gel filtration and Q-Sepharose. (a) The chromatogram of Sephadex G-100 gel filtration. The sample was eluted at a flow rate of 0.25 ml.min−1. The fraction of evaluation based on OD 280 and enzyme activity. (b) Separation of sesame LOX by ion-exchange chromatography on Q-Sepharose column at a flow rate of 1 ml.min−1 using 20 mM Tris-HCL buffer, pH 8.8, as the eluting buffer with a linear gradient of 0.1–1.0 M NaCl. 2 ml fractions were collected. Blue line indicates lipoxygenase activity; red line represents absorbance at 280 nm.
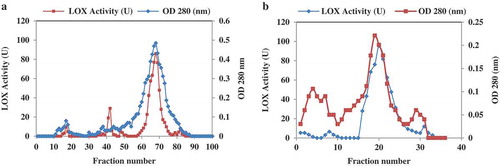
According to the results depicted in , fractions including 61–78 showed more activity than the others. Fraction 68 exhibited the most LOX activity. Those fractions possessing LOX activity were collected and subjected to Q-Sepharose column as the second round of chromatography. As shown in , fraction numbers of 16–25 displayed higher activity; meanwhile, the maximum activity was observed in fraction 20, which eluted at 0.3 M NaCl. Active fractions were dialyzed against 50 mM of Tris-HCL pH 8.0. The summary of purification results is given in . Through the protocol used herein, the enzyme was purified approximately 264-fold to a final specific activity of 159.4U.mg−1. In order to evaluate the purity of the sample and also the molecular weight of the enzyme, SDS-PAGE was carried out. As given in , isolated enzymes revealed a molecular weight of 66.3 kDa.
Table 1. Summary of lipoxygenase purification data from Sesamum indicum.
Figure 2. SDS-PAGE of LOX. Lane 1, standard molecular weight proteins (kDa) and lane 2, LOX purified.
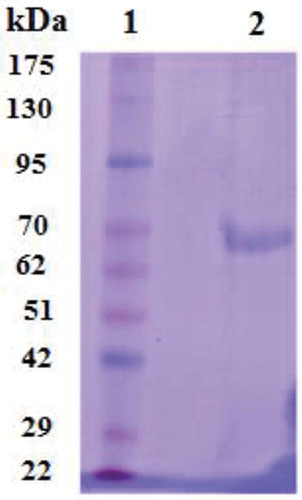
Effect of pH on LOX activity and stability
LOX activity showed up to 80% in the pH range of 7.5–8.5 with a maximum value at pH 8.0. The enzyme had no activity at pH 5.0 or below as well as at pH values more than 9.5. Based on , the LOX activity was observed in the range of alkaline pH values. Longer incubation of LOX under the above-mentioned conditions revealed the enzyme was the most active at pH 8.0.
Influence of temperature on activity and stability
The optimum temperature for LOX enzyme activity was 35 °C (). The enzyme had only 7.2% activity at 70 °C. More than 50% of the original activity was retained at the temperature of 30–50 °C. By increasing the incubation time, the thermal effect on the enzyme structure led to a decrease in the enzyme activity. The result of thermal stability of the LOX enzyme at 20–70 °C is shown in . The enzyme activity decreased progressively when the temperature increased more than 35 °C. The enzyme had 94% of its activity at 35 °C compared with a control incubated for 10 min at the same condition. The LOX stability gradually decreased at 35°C and above. After heating for 10 min, the residual LOX activities were about 12.8% at 65°C and 7.9% at 70 °C.
Half-life and Eai of enzyme reaction
Our results suggested that the LOX isolated had a half-life of 140 min at 35°C. However, the enzyme showed half-life values of 154 min and 90 min at temperatures of 25 °C and 45°C, respectively (). As shown in , the enzyme showed a Ki value after incubation at each temperature. By plotting Ki values versus 1/T, Eai of enzyme reaction was calculated. Results showed that the LOX-catalysed reaction had an Eai value of 10.1 kcal.mol−1 ().
Kinetic measurement
Michaelis–Menten and Lineweaver–Burk plots are illustrated in . Using linoleic as the substrate, the obtained kinetic parameters for the LOX reaction were measured. The isolated enzyme shows a Km value of 1.01 μmol and a Vmax value of 0.054 μmol min−1.
Figure 6. Some properties of the enzyme. (a) Lineweaver–Burk plot of the purified lipoxegenase from sesame seed. (b) Relative LOX activity in the presence of chelators such as EDTA and 1,10 phenanthroline. (c) The effect of organic solvents on LOX activity and (d) the effect of different metal ions on LOX activity.
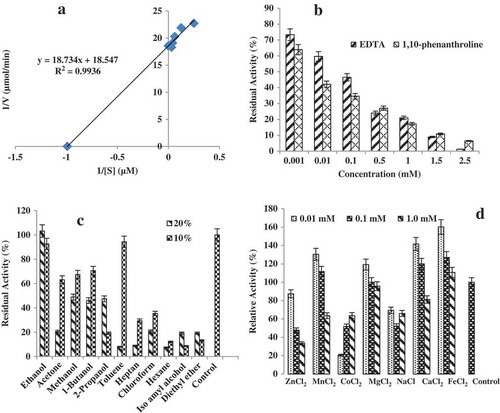
Effect of chemical agents on the LOX enzyme
Our results showed that both EDTA and 1,10-phenanthroline at 2.5 mM could decrease the enzyme activity by 98% and 93.5%, respectively (). Interestingly, our findings showed that PMSF at the tested concentrations had no significant effect on losing the enzyme activity. By increasing the concentration of β-mercaptoethanol from 0.01 mM to 1.5 mM, the LOX activity also decreased from 94% to19%, respectively (figure not shown).
Effect of organic solvents, detergent, and metal ions on enzyme activity
The influence of organic solvents on LOX activity was studied. Among the different solvents used only in the presence of ethanol (10% and 20%) and toluene (10%), the enzyme activities were remarkably maintained, whereas the enzyme activity decreased significantly at 10% and 20% of hexane, iso amyl alcohol, and diethyl ether (). The most organic solvents were able to decrease the activity of the LOX. Herein, no predicable correlation was observed between the type of solvent and the LOX residual activity.
At the highest concentration of SDS (2.0 mM), the enzyme exhibited 12% residual activity (). This effect may be because of the binding of the detergent to the enzyme and the breaking of the weak-stabilizing bands of the tertiary structure such as ionic hydrogen and hydrophobic. Meanwhile, at 0.25 mM of SDS, only 8.7% of the initial LOX activity decreased.
Table 2. The effect of various detergents on LOX activity.
Divalent ions such as Fe2+, Ca2+, and Mn2+ acted as activators for the enzyme, while metal ions such as Co2+, Zn2+, and Na+ inhibited the activity of LOX (Fig. 6d). The activation of LOX enzyme in the presence of metal ions was in the order of Fe2+>Ca2+> Mn2+>Mg2+>Zn2+>Na2+>Co2+.
Inhibition experiments
β-carotene inhibited LOX activity in a dose-dependent manner with IC50 of 48 μM. Ascorbic acid and KCN inhibited the enzyme relative activity with IC50 values of 132 μM and 270 μM, respectively.
Discussion
SDS-PAGE of the purified LOX enzyme showed a molecular weight of 66.3 kDa, which was in the range previously reported for LOXs isolated from Brassica napuscv, westar (possessing three isoforms of 62, 72, and 106 kDa)[Citation15] and the molecular weight of LOXs obtained from sweet corn germ (90.5 kDa), peanuts (73 kDa), pea seeds (74 kDa), cowpeas (68 kDa), and avocado (74 kDa).[Citation16] The isolated LOX enzyme showed maximum activity at pH 8. The pH profile of the LOX reaction implies stability of the tertiary structure of the enzyme over the applied pH values. An LOX from broad bean (Vicia faba) showed the maximal activity in the pH range of 9.0–10.0;[Citation17] however, the LOX obtained from tomato fruit and Luffa aegyptiaca (sponge gourd) exhibited optimal pH values at 6.5 and 7.0, respectively.[Citation3,Citation18] The LOX obtained from green algae (Oscillatoria sp.) showed a pH optimum at 8.8.[Citation19]
Maximum activity of the purified enzyme was observed at 35 °C. Formerly reports also showed the LOX derived from various sources were active at temperatures ranging from 30 °C to 50 °C. For example, LOX purified from banana leaf (Giant cavendishii, AAA)[Citation20] and cut lettuce (Lactuca sativa)[Citation21] showed an optimal thermal activity at 40 °C, and 30 °C, respectively. It seems that the LOX extracted from such plant sources has a moderate thermal activity ranging from 30 °C to 40 °C.
Bisakowski[Citation22] reported an Eai value of 8.86 kcal.mole−1 for an LOX extracted from Fusarium proliferatem. Al-Obaidy and Siddiqi[Citation23] reported a value of 7.1 kcal.mol−1 for broad-bean LOX. In addition, an Eai of 4.53 kcal.mol−1 for LOX immature English peas was reported by Ou Chen.[Citation24] In general, this parameter of energy for chemical reactions ranges from 6 to 15 kcal mol−.[Citation25]
The isolated enzyme shows a Km value of 1.01 μmol and a Vmax value of 0.054 μmol min−1. The kinetic parameters reported for two LOXs from cut lettuce (Lactuca. sativa) are as follows: LOX1 possessed Km = 0.33 mmol, Vmax = 0.24 μmol.min−1, and LOX2 had Km = 0.98 mmol, Vmax = 0.24 μmol.min−.[Citation21] For other LOX too these parameters have been reported: tomato fruit (Km = 6.2 μmol, Vmax = 10.3 μmol Min−1mg−1),[Citation3] banana leaf (Giant cavendishii, AAA) (Km = 0.15 mM, Vmax = 2.4 μmol min−1mg−1),[Citation20] and LOX-derived olive (Km = 11.82 μmol, Vmax = 24.15 nmol.ml−1.min−1).[Citation6] Our parameters were in the range of those reported for LOXs of tomato fruit and olive.
Our results showed that both EDTA and 1,10-phenanthroline at 2.5 mM could decrease the enzyme activity by 98% and 93.5%, respectively. EDTA might form a complex with the iron in LOX and lead to a decrease in activity. In fact, EDTA and 1,10-phenanthroline cause inactivation of the enzyme by the formation of bonds with the active site of the enzyme, which blocks the binding between the enzyme and the substrate.[Citation16] EDTA as a metal chelating agent has been reported to have an inhibitor for LOX in some plants, e.g. broad beans[Citation23] and soybeans.[Citation26] However, this chelating agent had no affect on LOX activity in sunflower seeds[Citation27] and barley.[Citation28] Decreased activity might be a result of chelating a non-heme iron atom in soybean LOX.[Citation4,Citation26,Citation29–Citation32]
The effect of detergent on LOX activity showed that Triton X-100 led to a decrease in enzyme activity, but the level of effect was lower than SDS. Pinsky[Citation33] reported that Triton X-100 decreased the LOX activity in soybean, pea, spinach, and cauliflower.[Citation16] Among the studied metal ions, Fe2+ had the maximum effect on the LOX activity at 0.01 mM concentration, and, Co2+ strongly decreased the enzyme activity at the same concentration. Consequently, it seems that these ions have varying effects on the oxygenating activity of LOX or the binding of fatty acid substrate to the active site. At the concentration of 1.0 mM, Zn2+ inhibited enzyme activity of about 66.5% compared with the control and Mg2+ had no significant effect on LOX activity. Enzyme inhibition may be justified through the competitive reduction of the ferric ion, which is necessary for LOX activity. Soybean LOX activity has been inhibited by Mg2+ and Mn2+, whereas its activity was increased vigorously by Fe2.[Citation34]
β-carotene exhibited an IC50 of 48 μM on the purified enzyme, while Serpen et al.[Citation35] reported that β-carotene had an IC50 value of 2.5 μM against the LOX activity derived from soybean. Inhibition of LOX by β-carotene was predominately of the noncompetitive type.[Citation35] The presence of β-carotene keeps LOX in the reduction form of Fe2+ to prevent from the oxidation of linoleic acid. Ascorbic acid proved to be a competitive inhibitor of LOX. The ability of ascorbic acid to inhibit linoleic acid oxygenation by LOX was dependent on the pH of the reaction. Besides, ascorbic acid strongly suppressed the formation of oxodienes, the side products of linoleic acid oxidation, which are inactive of the dissociation of free radicals from the active site.[Citation1,Citation20] Cyanide ion can form very stable complexes with the heavy metal of the LOX enzyme, thereby leading to enzyme inhibition. Among the inhibitors tested, β-carotene proved to be a potent inhibitor of LOX followed by ascorbic acid and KCN. The degree of inhibition is typically dependent on several factors such as the type of inhibitor and its concentration, pH, temperature, nature and concentration of the buffer, ionic strength, enzyme source, and enzyme and substrate as well as reaction time.
Conclusion
LOX extracted from sesame seed had an optimal pH, which was similar to green algae (Oscillatoria sp.). In general, LOX enzymes have multilateral effects on the growth and evolution of plants. Principally, the LOX purified from sesame may be utilized for understanding the oxidoreductase enzyme mechanism. Sesame LOX could be used for the production of aldehyde volatiles as well as potential regulatory molecules such as traumatic acid, traumatin, and jasmonic acid. Moreover, the use of the isolated LOX as an indicator of proper blanching is recommended, although such application for LOX described herein needs to be investigated further in the near future.
Funding
This research was supported by a grant from Ferdowsi University of Mashhad, Iran (No: 3/31656 on 24-03-1393), which is appreciated by the authors.
Additional information
Funding
References
- Maccarrone, M.; Veldink, G.A.; Vliegenthart, J.F.G.; Finazzi Agro, A. Inhibition of Soybean Lipoxygenase-1 by Chain-Breaking Antioxidants. Lipids 1995, 30, 51–54.
- Nguyen, M.D.; Nguyen, D.H.; Yoo, J.M.; Myung, P.K.; Kim, M.R.; Sok, D.E. Effect of Endocannabinoids on Soybean Lipoxygenase-1 Activity. Bioorganic Chemistry 2013, 49, 24–32.
- Bowsher, C.G.; Ferrie, B.J.M.; Ghosh, S.; Todd, J.; Thompson, J.E.; Rothstein, S.J. Purification and Partial Characterization of a Membrane-Associated Lipoxygenase in Tomato Fruit. Plant Physiology 1992, 100, 1802–1807.
- Pistoriuss, E.K.; Axelerod, B. Iron, an Essential Component of Lipoxygenase. Journal of Biological Chemistry 1974, 249, 3183–3186.
- Kermasha, S.; Metche, M. Characterization of Seed Lipoxygenase of Phasoelusvugariscv Haricot. Journal of Food Science 1986, 51, 1224–1227.
- Lorenzi, V.; Maury, J.; Casanova, J.; Berti, L. Purification, Product Characterization and Kinetic Properties of Lipoxygenase from Olive Fruit (Oleaeuropaea L.). Plant Physiology and Biochemistry 2006, 44, 450–454.
- Park, K.M.; Kim, Y.N.; Choi, S.J.; Chang, P.S. Development of the Simple and Sensitive Method for Lipoxygenase Assay in AOT/Isooctane Reversed Micelles. Food Chemistry 2013, 138, 733–738.
- Baysal, T.; Demirdoven, A. Lipoxygenase in Fruits and Vegetables: A Review. Enzyme and Microbial Technology 2007, 40, 491–495.
- Monteiro, E.M.H.; Chibli, L.A.; Yamamoto, C.H.; Pereira, M.C.S.; Vilela, F.M.P.; Rodarte, M.P.; De Oliveira Pinto, M.A.; Do Amaral, M.P.H.; Silverio, M.S.; De Matos Araujo, A.L.S.; De Araujo, A.L.A.; Del-Vechio-Vieira, G.; De Sousa, O.V. Antinociceptive and Anti-Inflammatory Activities of the Sesame Oil and Sesamin. Nutrients 2014, 6, 1931–1944.
- Borchani, C.; Besbes, S.; Blecker, C.; Attia, H. Chemical Characteristics and Oxidative Stability of Sesame Seeds, Sesame Paste, and Olive Oils. Journal of Agricultural Science and Technology 2010, 12, 585–596.
- Svendsen, A.;. Enzyme Functionality Design, Engineering, and Screening; Marcel Dekker, Inc:New York, USA, 2004.
- Amoo, S.O.; Okorogbona, A.O.M.; Du Plooy, C.P.; Venter, S.L. Sesamum indicum, Medicinal Spices and Vegetables from Africa; London, UK: Academic Press, 2017; 549–579.
- Henriques Monteiro, É.M.; Chibli, L.A.; Yamamoto, C.H.; Santana Pereira, M.C.; Pinto Vilela, F.M.; Rodarte, M.P.; … De Sousa, O.V. Antinociceptive and Anti-Inflammatory Activities of the Sesame Oil and Sesamin. Nutrients 2014, 6, 1931–1944.
- Ikuo, S.; Hajika, M.; Nishiba, Y.; Furuta, S.; Igaita, K. Simple and Rapid Method for the Selective Detection of Individual Lipoxygenase Isozymes in Soybean Seeds. Journal of Agricultural and Food Chemistry 1995, 43, 742–747.
- Kermasha, S.; Khalyfa, A.; Alli, 1.; Lee, B. Purification and Characterization of Lipoxygenase Isozymes from Canola (Brussicunupus Cv, Westar) Seed. Journal of Food Biochemistry 1991, 15, 219–238.
- Theerakulkait, C.; Barrett, D.M. Lipoxygenase in Sweet Corn Germ: Isolation and Physicochemical Properties. Journal of Food Science 1995, 60, 1029–1033.
- Clemente, A.; Olias, R.; Olias, J.M. Purification and Characterization of Broad Bean Lipoxygenase Isoenzymes. Journal of Agricultural and Food Chemistry 2000, 48, 1070−1075.
- Elemo, G.N.; Elemo, B.O.; Onwunbolu, L.O.; Okafor, O.Y. Isolation and Characterization of Lipoxygenase from Spong Gourd (Luffaaegyptiaca). African Scientist 2010, 11, 157–164.
- Beneytout, J.L.; Andrianarison, R.H.; Rakotoarisoa, Z.; Tixier, M. Properties of a Lipoxygenase in Green Algae (Oscillatoria Sp.). Journal of Plant Physiology 1989, 91, 367–372.
- Kuo, J.M.; Hwang, A.; Yeh, D.B.; Pan, M.H.; Tsal, M.L.; Sun, B. Lipoxygenase from Banana Leaf: Purification and Characterization of an Enzyme that Catalyzes Linoleic Acid Oxygenation at the 9-Position. Journal of Agricultural and Food Chemistry 2006, 54, 3151–3156.
- Altunkaya, A.; Gökmen, V. Purification and Characterization of Polyphenol Oxidase, Peroxidase and Lipoxygenase from Freshly Cut Lettuce (L. sativa). Food Technology and Biotechnology 2011, 49, 249–256.
- Bisakowski, B.; Kermasha, S.; Lavorel, V.; Belin, J.M. Partial Characterization of a Lipoxygenase from Fusarium proliferatum. Food Biotechnology 1995, 9, 189–205.
- Al-Obaidy, H.M.; Siddiqi, A.M. Inhibition of Broad Bean Lipoxygenase. Journal Food Sciences 1981, 46, 597–600.
- Ou Chen, A.; Whitaker, J.R. Purification and Characterization of a Lipoxygenase from Immature English Peas. Journal of Agricultural and Food Chemistry 1986, 34, 203–211.
- White, A.; Handler, P.; Smith, E. Principle of Biochemistry; McGraw-Hill Book Co; New York, USA, 1973.
- Chan, H.W.S.;. Soy-Bean Lipoxygenase: An Iron-Containing Dioxygenase. Biochimica Et Biophysica Acta 1973, 327, 32–35.
- Leoni, O.; Iori, R.; Palmieri, S. Purification and Properties of Lipoxygenase in Germinating Sunflower Seeds. Journal Food Sciences 1985, 50, 88–92.
- Lulai, E.C.; Baker, C.W. Physicochemical Characterization of Barly Lipoxygenase. Cereal Chemistry 1976, 53, 777–786.
- Draheim, J.E.; Carroll, R.T.; McNemar, T.B.; Dunham, W.R.; Sands, R.H.; Funk, M.O.J. Lipoxygenase Isozymes: A Spectroscopic and Structural Characterization of Soybean Seed Enzymes. Archives of Biochemistry and Biophysics 1989, 269, 208–218.
- Navaratnam, S.; Feiters, M.C.; Al-Hakim, M.; Allen, J.C.; Veldink, G.A.; Vliegenthart, J.F.G. Iron Environment in Soybean Lipoxygenase-1. Biochimica Et Biophysica Acta 1988, 956, 70–76.
- Nelson, M.J.;. Catecholate Complexes of Ferric Soybean Lipoxygenase 1. Biochemistry 1988, 27, 4273–4278.
- Roza, M.; Francke, A. Soy Bean Lipoxygenase: An Iron-Containing Enzyme. Biochimica Et Biophysica Acta 1973, 327, 24–31.
- Pinsky, A.; Grossman, S.; Trop, M. Lipoxygenase Content and Antioxidant Activity of Some Fruits and Vegetables. Journal of Food Science 1971, 36, 571–572.
- Aberomand, M.; Kheirollah, A.; Nikzamir, A.; Malekasgar, A.M.; Alimohammadi, M. The Inhibitory Effect of KCN, NAN3 and Some Bivalent Ions on Lipoxygenase Activity of the Purified Human Placental, Iranian. Journal of Pharmaceutical Sciences 2013, 9, 39–45.
- Serpen, A.; Gokmen, V. Effects of Β-Caroten on Soybean Lipoxygenase Activity: Kinetic Studies. European Food Research and Technology 2007, 224, 743–748.