ABSTRACT
Lotus rhizome starch was isolated and subjected to chemical modification using different levels of oxidation, cross-linking, and dual modifications. Fourier transform infrared (FTIR) and X-ray diffraction analyses confirmed the successful modification of native starch. The carbonyl, carboxyl contents, and degree of cross-linking of modified starch were in the range of 0.03–0.07%, 0.07–0.15%, and 4.31–58.28%, respectively, for oxidized, cross-linked, and dual-modified starches. Amylose content varied from 13.57% to 20.60% for native and modified starches. Swelling power (SP) and solubility were temperature dependent and increased with increase in temperature. Oxidation and dual modification increased solubility, while cross-linking decreased solubility. Paste clarity, degree of crystallinity, and whiteness of starches increased significantly with increase in level of modifications. Peak, breakdown, and setback viscosities of the modified starches decreased with increase in modification. Phase transitions using DSC presented lower enthalpy values for modified starches. Gelatinization temperature increased due to modification whereas scanning electron micrographs revealed that modification altered the starch morphology.
Introduction
Starch is an important polymer and is immensely important for food as well as other industries. It contributes to the textural properties of food products such as thickening, gelling, binding, stabilizing, and bulking agent.[Citation1] Native starch lacks some functional aspects, which limits its use at the industrial level. The diversity in the modern food industry and food products demands starch that can withstand various processing techniques, storage, and distribution conditions. The improved functional properties of modified starches motivate their exploitation as an important functional ingredient over native starch.[Citation2] Chemical modification has been considered a classical way to markedly alter the physicochemical and functional characteristics by introducing new functional groups into starch molecules;[Citation1] however, the degree of modification of starch to be used in food applications is limited by legislation.[Citation3]
Starch oxidation is a chemical modification in which hydroxyl groups of the starch molecules are initially oxidized to carbonyl groups and then to carboxyl groups as the primary final product.[Citation4] The number of carbonyl and carboxyl groups on the oxidized starch indicates the extent of starch oxidation. Oxidized starches possess low viscosity, high thermal stability, low reterogradation, paste clarity, film forming, and binding properties.[Citation5] Among the different oxidizing reagents used, sodium hypochlorite (NaOCl) is the most commonly used modifying agent. Cross-linking is another chemical method of starch modification used for the preparation of thermal- and shear-resistant starches with restricted swelling. However, the effect of cross-linking depends on the botanical source of starch, cross-linking reagents, and the reaction conditions.[Citation1] Recently, sodium trimetaphosphate (STMP), a slow-acting cross-linking agent, has been proposed as a non-toxic that can penetrate further into the interior of starch granules, resulting in evenly distributed cross-linking throughout the granule volume.[Citation6] The maximum concentration of the modifying agent, STMP, allowed by FDA for starches intended for food industries is 3% (w/w) with respect to the weight of the starch.[Citation7] The judicious combination of these starch modifications can bring desirable changes in the properties of starch. Therefore, in the present study, dual modification of starch was carried out first by cross-linking the starch using STMP, followed by oxidation. Different authors have studied the effect of dual modification on the properties of different starches [Citation1,Citation6,Citation8] and reported favourable results.
India demands around 27,500 tons of modified starch per annum, with a growth rate of 8–9% per annum.[Citation2] Corn is the major starch source for industrial application and is also a staple food. However, non-conventional starch sources like tubers and rhizomes are not utilized in industries as they are not well explored to date for their inherent properties. Starches from unconventional sources may be used as ingredients in the same manner as starches from cereals and tuber due to their similar characteristics. These characteristics can be improved by modification treatments and may be used to develop new processes and consequently new products. The authors had earlier studied the effect of oxidation, cross-linking, and dual modification on the properties of lotus rhizome starch at a single modification level[Citation9] and obtained desirable properties. Hence, the present research was extended to study the effect of oxidation, cross-linking, and dual modification at different modification levels on the physicochemical, thermal, pasting, structural, and morphological characteristics of lotus rhizome starches.
Materials and methods
Materials
Fresh lotus rhizome (Nelumbo nucifera) was procured from the certified centres of Punjab (India). Starch was isolated from lotus rhizomes using the method described by Sukhija et al.[Citation10] The chemicals used in the experimental work were purchased from S.D. Fine Chemicals Ltd. (India) and Sigma Aldrich (India) and were of AR grade.
Lotus rhizome starch modifications
Preparation of oxidized starch
Three levels of oxidation of starch using NaOCl were performed according to the method mentioned by Wang and Wang,[Citation11] with slight modifications.
Preparation of cross-linked starch
Three levels of cross-linking of starch with STMP were carried out using the method of Liu et al.[Citation8] Accordingly a total of nine different types/levels of modification were prepared for native lotus rhizome starch (NLS) as outlined next. Oxidation levels were chosen based on preliminary trails. Above 2.5% concentration of NaOCl, the viscosity values of oxidized starch were very low and could not form gel upon cooling. Cross-linking levels were selected at maximum 3% STMP according to the permissible limits of FDA.
Oxidation level-1 (OLS1): Containing NaOCl at 0.5% (w/w) active chlorine based on dry weight of starch.
Oxidation level-2 (OLS2): Containing NaOCl at 1% (w/w) active chlorine based on dry weight of starch.
Oxidation level-3 (OLS3): Oxidation was carried out using NaOCl at 1.5% (w/w) active chlorine on dry starch weight basis.
Cross-linking level-1 (CLS1): Cross-linking was carried out using STMP at 1% (w/w) on dry weight starch basis.
Cross-linking level-2 (CLS2): Cross-linking was carried out using STMP at 2% (w/w) based on dry weight of starch.
Cross-linking level-3 (CLS3): Cross-linking was carried out using STMP at 3% (w/w) based on dry weight of starch.
Dual modification-1 (COLS1): CLS1 followed by OLS1.
Dual modification-2 (COLS2): CLS2 followed by OLS1.
Dual modification-3 (COLS3): CLS3 followed by OLS1.
Carbonyl, carboxyl contents, and degree of cross-linking
The carbonyl content was determined according to the method of Smith.[Citation12] The carboxyl content was determined according to the method of Wang and Wang.[Citation11] The relative degree of cross-linking of starch was determined according to the procedure of Kaur et al.[Citation13]
Physicochemical properties
Water-binding capacity was determined using the method of Yamazaki[Citation14] and Medcalf and Gilles.[Citation15] Amylose content of the isolated starches was determined following the colorimetric method as described by Morrison and Laignelet.[Citation16] Swelling power (SP) and solubility of starches were determined by the method of Balasubramanian et al.[Citation17] with slight modifications. Paste clarity (% transmittance) was measured according to Perera and Hoover,[Citation18] with slight modifications. Colour was measured using Hunter Lab Colorimeter (D-25, Hunter Associates Laboratory, Ruston, USA).
Structural characteristics
X-ray diffractograms of starches were recorded by a copper anode X-ray tube using an analytical diffractometer (Panalytical, X’pert PRO, The Netherlands) according to Nuwamanya et al.[Citation19] using Image Tool Software (UTHSCSA, 2002). The Fourier transform infrared (FTIR) spectra of native and modified starches was recorded using an FTIR spectrophotometer (Agilent Cary 660, United States) in the range of wavenumbers from 4000 to 400 cm−1. The absorbed water was removed from samples by drying at 40°C for 48 h prior to analysis.
The morphological characteristics of starch granules were observed using a scanning electron microscope (SEM, JEOL, USA) under an acceleration voltage of 20 kV at different magnifications. The pasting properties (pasting temperature, peak viscosity, trough viscosity, final viscosity, breakdown, and setback values) of the starches were evaluated with Rapid Visco Analyzer (RVA-4, Newport Scientific, Warriewood, Australia). Thermal characteristics (onset temperature (To); peak temperature (Tp); conclusion temperature (Tc), and enthalpy of gelatinization, ΔHgel) of isolated starches were determined using a differential scanning calorimeter (DSC, model-821e, Mettler-Toledo, Switzerland).
Statistical analysis
The analyses were carried out in triplicates and subjected to one-way Analysis of variance (ANOVA) (±SD) by Duncan’s test using Statistica-7. The mean values have been represented at the p ≤ 0.05 level.
Results and discussion
Carbonyl, carboxyl contents, and degree of cross-linking
represents the carbonyl and carboxyl contents of modified starches. Oxidation of lotus rhizome starch promoted the formation of carbonyl and carboxyl groups, which increased with increase in the level of oxidation. Other studies also reported an increase in carbonyl and carboxyl contents of different starches, i.e. barley, tamarind seed, and potato, oxidized with NaOCl at different oxidant concentrations.[Citation3,Citation4] The cleavage of the glycosidic bond during the oxidation process caused the depolymerisation of starch molecules, which created conditions for the formation of carbonyl and carboxyl groups. The oxidation reaction in the present study occurred at alkaline pH conditions, which, according to Wurzburg,[Citation20] favoured the formation of carboxyl groups. Similarly, higher carbonyl and carboxyl groups were also obtained for dual-modified starches. However, with an increase in the level of cross-linking, the carbonyl and carboxyl contents of dual-modified starches decreased, may be due to an increase in the degree of cross-linking. Liu et al.[Citation8] reported similar results of higher carbonyl and carboxyl contents of dual-modified corn starch and concluded that in order to achieve the same oxidation level, cross-linked starch would use less oxidant than native starch. Moreover, cross-linking could prevent the starch molecules from rearranging during drying, hence providing a more exposed internal structure to the oxidant and facilitating oxidation. Dual-modified starches represented a higher degree of cross-linking than cross-linked starches at all levels of cross-linking as reported by Koo et al.[Citation21]
Table 1. Carbonyl and carboxyl contents and degree of cross-linking of native and modified lotus rhizome starches*.
Physicochemical properties
Amylose contents of native and modified lotus rhizome starches are listed in . A significant reduction in the amylose content of starch was observed as a result of oxidation, cross-linking, and cross-linking oxidation. The amylose content of oxidized starches decreased progressively with increase in oxidant concentration. These results are consistent with the findings of Chavez-Murillo et al.[Citation22] and Kaur and Bhullar[Citation23] for oxidized barley, corn, and tamarind seed starches. The reason behind the decrease in amylose content upon oxidation may be attributed to the susceptibility of amylose to oxidative degradation due to its linear structure and random arrangement.[Citation24] The decrease in the amylose content of cross-linked starch could be attributed to the intermolecular bonding between the amylose and amylopectin molecules or within the amylose molecules.[Citation1] Dual-modified starches presented higher amylose content than oxidized starches, may be due to the introduction of phosphate groups into starch molecules during cross-linking, which resulted in strengthening of the starch structure and minimized the effect of oxidative depolymerisation during oxidation.
Table 2. Physicochemical properties of native (NLS) and modified lotus rhizome starches*.
represents the water-binding capacities of native and modified starches. Oxidized, cross-linked, and dual-modified starches had shown higher values of water-binding capacity than their native counterparts. Lawal[Citation25] quoted that modification facilitates the addition of new functional groups causing the weakening of hydrogen bonds present in starch, which in turn results in the penetration of water into granules. These results lend credence to the observations of different researchers for oxidized/cross-linked cocoyam and cassava starches.[Citation26] In the present study, cross-linked oxidized lotus rhizome starches presented higher water-binding capacity than cross-linked starches due to the addition of hydrophilic carboxyl groups resulting from the oxidation of cross-linked starch.
shows the L* values of colorimetric assay, representing the whiteness of native and modified starches. Oxidation, cross-linking, and dual modification presented higher L* value of lotus rhizome starch and it increased with increase in the level of modification. Sanchez-Rivera et al.[Citation5] and Vanier et al.[Citation27] stated that the oxidation of starch increases the whiteness of starch by oxidizing pigments and protein of native starch prior to glucose units. Also, the L* values of cross-linked starches were higher than those of native starches but were lower than the oxidized and dual-modified starches at their respective modification levels. Hazarika and Sit[Citation1] have also reported remarkable improvement in the lightness of starch following dual modification. The possible reason for the improved whiteness of starch due to cross-linking was reported as a result of the use of NaOH during modification, which imparted a bleaching effect.
The paste clarity at (%T650) of native and modified lotus rhizome starches is listed in . The paste clarity of modified starches decreased with increase in storage period. During refrigerated storage, molecular realignment of solubilized starch chains occurred, which resulted in a decrease in paste clarity. An increase in paste clarity of oxidized starches compared with native starch may be attributed to the chemical substitution of hydroxyl groups with hydrophilic carboxyl groups, which hindered molecular reassociation. Cross-linking of starch led to a substantial decrease in the paste clarity of modified starch. These results are consistent with the reports of Kaur et al.[Citation13] and Koo et al.[Citation21] for cross-linked starch. Furthermore, the reduced SP of cross-linked starch () limited their dispersion and reduced paste clarity.[Citation13] With increase in the degree of cross-linking, the paste clarity of cross-linked starches decreased significantly due to the increase in cross-linked density.
Figure 1. Swelling power of native (NLS) and modified lotus rhizome starches at different temperatures.
Swelling power: 1. Native lotus rhizome starch (NLS); 2. Oxidation level-1 (OLS1); 3. Oxidation level-2 (OLS2); 4. Oxidation level-3 (OLS3); 5. Cross-linking level-1 (CLS1); 6. Cross-linking level-2 (CLS2); 7. Cross-linking level-3 (CLS3); 8. Dual modification-1 (COLS1); 9. Dual modification-2 (COLS2); 10. Dual modification-3 (COLS3): at different temperatures.
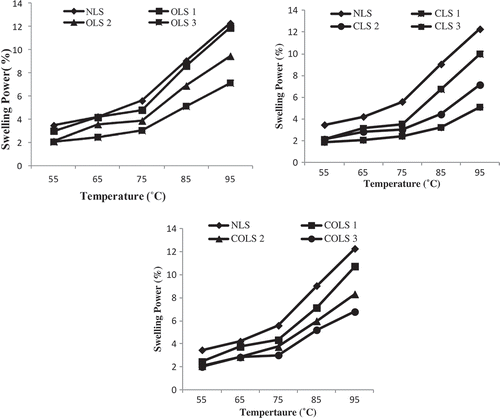
SP and solubility of lotus rhizome starches
and represent the effect of temperature, oxidation, cross-linking, and cross-linking oxidation at different levels, on the SP and solubility of lotus rhizome starches. With an increase in temperature from 55° to 95°C, there was a progressive increase in SP and solubility of native as well as modified starches. The reason behind may be attributed to the unfolding of the granular structure of starch due to the commotion of hydrogen bonds present between the starch chains. Wang and Wang[Citation11] explained that at high temperature, starch samples gelatinize sufficiently and also hydrolyse amylopectin chains, causing an increase in SP.
Figure 2. Solubility of native and modified lotus rhizome starches at different temperatures.
Solubility: 1. Native lotus rhizome starch (NLS); 2. Oxidation level-1 (OLS1); 3. Oxidation level-2 (OLS2); 4. Oxidation level-3 (OLS3); 5. Cross-linking level-1 (CLS1); 6. Cross-linking level-2 (CLS2); 7. Cross-linking level-3 (CLS3); 8. Dual modification-1 (COLS1); 9. Dual modification-2 (COLS2); 10. Dual modification-3 (COLS3): at different temperatures.
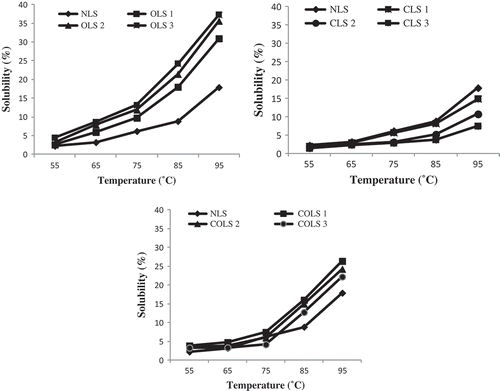
A significant reduction in SP of lotus rhizome starch was observed after oxidation and it decreased with increase in the level of oxidation. These results are consistent with the findings of different researchers for oxidized cocoyam, banana, bean, barley, tamarind seed, and potato starches.[Citation4,Citation23,Citation27] Oxidation results in weakening of the internal structure and disintegration within starch granules, which reduced the SP of oxidized starch. Halal et al.[Citation3] stated that oxidation at low levels occurs primarily in the amorphous region of starch granules, whereas, at higher oxidation level, partial hydrolysis of the amylopectin chain occurs. Cross-linking caused significant reduction in SP of starch. It is well known that cross-linking reinforces the bonding between starch chains and increases their resistance against swelling. With increase in the level of cross-linking reagent, SP of cross-linked starches decreased due to increase in the degree of cross-linking (). The increased density of cross-links hindered the penetration of water into the starch molecular network and resulted in the reduction of SP. Similar results for the reduction in SP of cross-linked starches have been earlier reported for oat, potato, cassava, jackfruit, and corn starches.[Citation6,Citation28,Citation29] Dual-modified lotus rhizome starches also presented reduction in SP compared with native starch, which may be due to the combined effect of cross-linking and oxidation. Other researchers have reported similar findings for SP of dual-modified rice starch[Citation30] and elephant foot yam starch.[Citation31]
represents the solubility of native and modified lotus rhizome starches (at different levels of modification) measured at different temperatures. Oxidized and dual-modified starches presented an increase in the solubility with increase in levels of modification. The increase in solubility of oxidized and dual-modified starches is attributed to the weakening of the internal structure of the starch granules and to amylose depolymerization.[Citation24] Zhou et al.[Citation4] stated that oxidation reaction mainly occurs in the amorphous regions of starch, which makes it more susceptible to leaching of molecular starch, due to the easier absorption of water and hence increases solubility. These results lend credence to the observations of other researchers for oxidized and dual-modified starches.[Citation3,Citation23,Citation31] Cross-linked starches showed an increase in solubility with increase in the temperature and level of cross-linking. However, the solubility of cross-linked starches was noticed to be lower than native starch, which suggested the density of cross-linked starches increased with increase in the concentration of cross-linking reagents, which resulted in less disintegration of starch granules during gelatinization and decreased solubility, which is consistent with the decrease in swelling behaviour.[Citation26]
X-ray diffraction
The X-ray diffraction curve of native and modified starches is shown in . Strong diffraction peaks appeared at 2θ of 15.07°, 23.09° and doublet at 17.10°, 18.01°, indicating typical type-A starch pattern. The diffractogram of starch was composed of two parts: typical polycrystalline starch, including peak diffraction, and dispersive diffraction.[Citation4] Oxidized, cross-linked, and dual-modified starches presented different peak intensities and degree of crystallinity compared with native starch. However, the positions of diffraction peaks of modified starches were still consistent with the position of peaks of NLS. Similar results for increase in peak intensity and degree of crystallinity of modified starches in comparison to their native counterparts were reported.[Citation21,Citation22]
Figure 3. X-ray diffractograms of native and modified lotus rhizome starches.
X-ray diffraction pattern: 1. Native lotus rhizome starch (NLS); 2. Oxidation level-1 (OLS1); 3. Oxidation level-2 (OLS2); 4. Oxidation level-3 (OLS3); 5. Cross-linking level-1 (CLS1); 6. Cross-linking level-2 (CLS2); 7. Cross-linking level-3 (CLS3); 8. Dual modification-1 (COLS1); 9. Dual modification-2 (COLS2); 10. Dual modification-3 (COLS3).
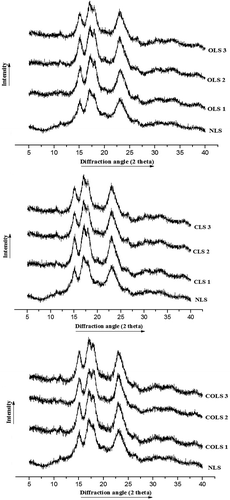
The degree of crystallinity of modified starches was observed to be higher than native starch and increased with increase in modification level (). A significant decrease in amorphous area results in an increase in crystallinity of the sample. Similar results have earlier been reported of crystallinity increase in oxidized barley, corn, and tamarind seed starches.[Citation23,Citation32] Zhou et al.[Citation4] reported an increase in the degree of crystallinity of oxidized starches only at lower active chlorine concentration. However, with increase in oxidation level, the degree of crystallinity of oxidized starches decreased because the molecular chains of crystallized starch were destroyed to some extent at higher levels of oxidation. Cross-linked and dual-modified starches also presented an increase in the degree of crystallinity than native starch but were lower than the oxidized starches. Hoover and Sosulki[Citation33] stated that the cross-linking mainly takes place in the amorphous region of starch granules, which do not change the crystalline pattern of starches.
Morphological characteristics
The scanning electron micrographs of native and modified lotus rhizome starches () depicted elongated, hemispherical granules with facets and oval shapes. Oxidation did not bring any changes in the size and shape of starch granules; however, with increase in oxidation level from 0.5% active chlorine concentration to 1.5% and 2.5% active chlorine, agglomeration of starch granules could be clearly seen (Fig. 4c and 4d). Zhou et al.[Citation4] have also reported cracks on the surface of potato starch granules at high oxidant concentration of 2% and 4% active chlorine and concluded that oxidation weakened the crystal structure of starch granules. The oxidized bean starch (1.5% active chlorine) presented imperfections in the external structure featuring a rougher surface than native starch, whereas no obvious changes were seen on the surface of starch granules oxidized with 0.5% and 1% active chlorine.[Citation28] Hence, it is clear from the present and previous studies that the extent to which the surface of oxidized starch granules gets affected depends on the concentration of oxidant used for modification.
Figure 4. Scanning electron micrographs (magnification 1000×) of native and modified lotus rhizome starches.
Scanning electron micrographs (magnification 1000×): 1. Native lotus rhizome starch (NLS); 2. Oxidation level-1 (OLS1); 3. Oxidation level-2 (OLS2); 4. Oxidation level-3 (OLS3); 5. Cross-linking level-1 (CLS1); 6. Cross-linking level-2 (CLS2); 7. Cross-linking level-3 (CLS3); 8. Dual modification-1 (COLS1); 9. Dual modification-2 (COLS2); 10. Dual modification-3 (COLS3)
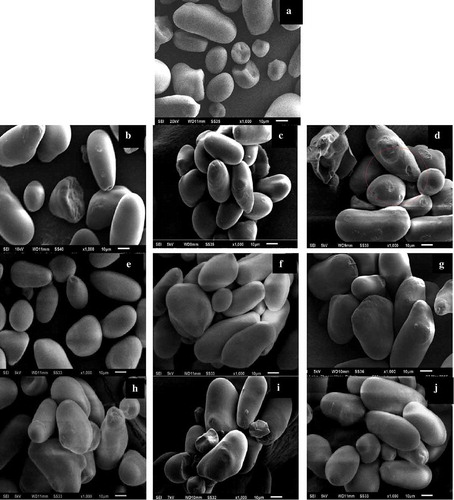
Cross-linking of lotus rhizome starch using STMP at different concentrations (1%, 2%, and 3%) resulted in smooth starch granules similar to native starch, suggesting that the modification did not cause any detectable morphological changes (–). These results are consistent with those reported in different studies for the cross-linking of jackfruit and elephant foot yam starches.[Citation29,Citation31] On the contrary, some researchers have reported a slightly rough surface and black zones on the surface of cross-linked starch granules.[Citation21] However, agglomeration of starch granules was observed clearly in the starch granules cross-linked at 2% and 3% levels of cross-liking. Dual-modified starches presented agglomeration of starch granules at different levels of modification, but no prominent changes in granule morphology were visualized. The reason behind this may be attributed to the strengthening of starch molecules in dual-modified starch resulting from the formation of cross-links in starch granules during cross-linking. Cross-linking followed by oxidation at a low concentration of oxidizing agent (i.e. 0.5% active chorine) positively affected the functional and pasting properties of dual-modified starches without altering the granule morphology.
FTIR spectroscopy
FTIR spectra presented in can be used to explore the structural organization of native and modified starches. The fundamental region of NLS represents a wide band at wavenumber 3397 cm−1, confirming the presence of O-H stretching vibration; the peak at 2930 cm−1 can be ascribed to the asymmetric C-H stretching. The peak at 1644 cm−1 was ascribed to adsorbed water, i.e. H-O-H bending vibrations, the peak at 1248 cm−1 was due to O-H bending vibration, the bands in 1369–1420 cm−1 could be assigned to C-H bending vibrations, and the peaks at 1156 cm−1 and 1080 cm−1 might be attributed to the C-O stretch of C-O-H in starch molecules. The peak around 1017 cm−1 was attributed to the vibration of C-O-H deformation. Oxidation of starch resulted in the conversion of hydroxyl groups into carbonyl and carboxyl groups and the changes in these groups were confirmed using FTIR spectroscopy. However, the FTIR spectra of oxidized starches at 0.5%, 1.5%, and 2.5% active chlorine concentrations did not present any difference compared to native starch, which may be the result of the formation of smaller carbonyl and carboxyl groups during oxidation. Similar results for no change in FTIR spectra of oxidized starches at different modification levels have been reported for barley starch.[Citation3] On the other hand, an additional peak at 1735.6 cm−1 was observed for oxidized starch by Zhang et al.[Citation34] due to the high oxidation degree (>40.5%) achieved in their study. It was mentioned that according to the chemical structure of STMP and the stretching vibrations of phosphate ester compounds, an absorption band of P-O-C stretching vibrations in fingerprint region is observed for cross-linked starches.[Citation6,Citation8] For cross-linked and dual-modified starches in the present study, a diminished peak at 1644 cm−1 was observed due to broken hydrogen bonds, which confirmed successful cross-linking. However, no difference in the FTIR spectra of cross-linked and dual-modified starches at different modification levels was observed.
Figure 5. FTIR spectra of native and modified lotus rhizome starches.
FTIR spectra: 1. Native lotus rhizome starch (NLS); 2. Oxidation level-1 (OLS1); 3. Oxidation level-2 (OLS2); 4. Oxidation level-3 (OLS3); 5. Cross-linking level-1 (CLS1); 6. Cross-linking level-2 (CLS2); 7. Cross-linking level-3 (CLS3); 8. Dual modification-1 (COLS1); 9. Dual modification-2 (COLS2); 10. Dual modification-3 (COLS3)
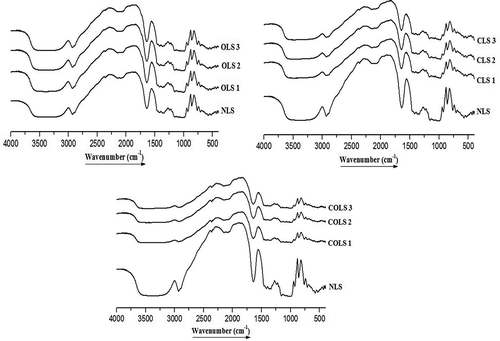
Pasting properties
represents the pasting properties of native, oxidized, cross-linked, and dual-modified lotus rhizome starches. Oxidized starches showed significantly lower pasting temperature compared to native starch, which decreased with increase in modification level. Similar results for decrease in pasting temperature upon oxidation have been earlier reported for potato, corn, rice, and barley starch.[Citation3,Citation32,Citation35] Decrease in the pasting temperature of oxidized starch may be attributed to the easier swelling of oxidized starch granules because the strength of association among the starch molecules weakens by repulsion with carboxyl groups due to the introduction of water in starch granules.[Citation35]
Table 3. Pasting properties of native (NLS) and modified lotus rhizome starches*.
Peak and final viscosities of oxidized lotus rhizome starch were found to be lower than that of native starch and decreased with increase in the levels of oxidation. Other researchers have also reported a similar trend of decrease in peak and final viscosities for potato, barley, and bean starches with increase in oxidant concentration.[Citation3,Citation4,Citation27] The decrease in viscosity of oxidized starch is a consequence of the partial cleavage of glycosidic linkages resulting in low molecular weight starches.[Citation25] In case of oxidized starch prepared at 2.5% (w/w) active chlorine, increase in viscosity during the cooling step was very low, which produced gel with softer consistency. Sanchez-Rivera et al.[Citation5] observed similar findings for oxidized banana starch at 2% active chlorine concentration and mentioned that at a higher concentration of active chlorine, the cleavage of starch chains was more prominent. This pattern was due to the network formation of short-chain alignments producing minor interactions, which led to softer gel consistency.
The thermal stability of starch pastes, i.e. the resistance of starch paste to heating and mechanical stirring, is represented by breakdown. The breakdown values of oxidized starches were found to be significantly lower than native starch and decreased with increase in modification level. Zhou et al.[Citation4] have also reported a similar trend of lower breakdown value of oxidized potato starch than that of native starch, and the value decreased with increase in oxidant concentration (0.1–4%). Halal et al.[Citation3] quoted that the starch with a higher degree of oxidation has a greater tendency to resist shear force during heating. The reduced reterogradation tendency of oxidized starches may be attributed to the introduction of voluminous carbonyl and carboxyl groups, which inhibited the reassociation of the chains.[Citation8]
Cross-linked lotus rhizome starch presented significantly higher pasting temperature than its native counterpart, which increased with increase in degree of cross-linking. Similar results have been earlier reported for corn[Citation6,Citation30] and cassava[Citation27] starches. The increase in the pasting temperature indicated successful cross-linking within starch granules. Peak viscosity of cross-linked starches decreased significantly with increase in the concentration of cross-linking agent and was found to be lower than native starch. However, the final viscosity of cross-linked starches was found significantly higher than that of native starch. These results are consistent with the findings of cross-linked cassava and corn starches.[Citation6,Citation26] Breakdown viscosity of cross-linked starch was lower than native as well as oxidized starch and decreased significantly with increased level of cross-linking, representing better thermal stability and shear resistance accorded by STMP cross-linking. On the other hand, setback viscosity was found to be higher (than native starch) at lower levels of cross-linking (1%), which could be attributed to the observed low peak viscosity value. However, with an increase in the degree of cross-linking, the setback viscosity also decreased.
Dual-modified starch presented lower peak, trough, breakdown, and final viscosities as compared to native, oxidized, and cross-linked starches at all levels of modification, elucidating successful oxidation followed by cross-linking. Xiao et al.[Citation30] also observed similar results for decrease in peak, final, and breakdown viscosities of dual-modified rice starch. In dual-modified starch, oxidation of cross-linked starch resulted in a decrease in viscosities in the whole range of viscosity profile. Pasting temperature and setback viscosity of dual-modified starch were lower than those of cross-linked starch.
Thermal properties
The thermal properties of native and modified lotus rhizome starches, i.e. To, Tp, Tc and ΔHgel, represent the amount of energy involved in the gelatinization process (). The To, Tp, and Tc of modified starches were significantly higher than those of native starch. Sandhu et al.[Citation24] stated that the thermodynamic characteristics of oxidized starches get altered due to the incorporation of carboxyl groups, causing weakening of the intermolecular bonds responsible for the crystalline form of amylopectin. Oxidized starches presented no significant difference in the onset and peak temperatures at different levels of modification. Sanchez-Rivera et al.[Citation5] stated that Tp is an average temperature, whereas the granules have a range of temperature where they can become disorganized; therefore Tp of the oxidized starches did not change with chlorine concentration. However, Tc and ΔHgel decreased significantly with increase in the oxidant level (0.5%–2.5% active chlorine) for oxidized starches. The decrease in ΔHgel with the increased level of oxidation represented weakening of the starch granules due to the partial degradation of starch at higher oxidant concentration. These results can be further confirmed by the lower viscosity values of oxidized starch at higher concentration (2.5% active chlorine) presented in . Similar results for decrease in ΔHgel for oxidized banana starches have been reported earlier.[Citation5]
Table 4. Thermal properties of native (NLS) and modified lotus rhizome starches*.
Cross-linked lotus rhizome starches exhibited significantly higher transition temperature than native starch. Gao et al.[Citation6] concluded that the higher transition temperature of cross-linked starch confirms the introduction of the phosphate group into starch molecules, leading to strengthening of bonds between starch chains, simultaneously increasing the resistance of starch granules to swelling and increasing the gelatinization temperature. The ΔHgel of cross-linked lotus rhizome starches increased with increase in the level of cross-linking; however, the values were significantly lower than those of native starch. Other authors have also reported significantly lower values of ΔHgel for cross-linked corn and jackfruit starches compared to their native counterparts and the reason was attributed to an increase in the free volume of starch chains due to the presence of bulky and ionic phosphate groups.[Citation29] Also, the decrease in ΔHgel of cross-linked rice starches was attributed to the reduced portion of starch granules that could be gelatinized, as a result of cross-linking.[Citation36] However, cross-linking did not change the onset and peak temperature significantly, whereas the conclusion temperature of cross-linked starches increased with increase in the degree of cross-linking, which has been reported for cross-linked oat starch.[Citation28]
Dual modified starches presented significantly higher onset and peak temperature than native, oxidized and cross-linked starch. All the starch granules in cross-linked starches could not gelatinize due to strengthening of the starch structure resulting from the incorporation of phosphate cross-linkages, whereas oxidation caused the hydrolysis of amorphous zones, conformational reordering, and rearrangement of the starch structure, which in turn increased the peak temperature of dual-modified starches. However, ΔHgel of dual-modified starches was found significantly higher than oxidized starches but lower than the cross-linked starches at different modification levels. Decrease in enthalpy of gelatinization was probably due to an increase in the relative crystallinity of samples.[Citation27] These results are consistent with the findings of Liu et al.[Citation8]
Conclusion
The starch industry is in constant expansion, and modification processes increase its versatility. When starch is modified physically or chemically, it can be adapted for different purposes in food and/or non-food industries. Applications of starch modifications can increase the use of unconventional starches in the industry. Depending on cost and accessibility, the use of conventional starch can be replaced in whole or in part by unconventional starches in industrial processes when appropriate. Determining the required characteristics of starch for each process is necessary to select the best modification method according to the application requirements, market trends, availability, structural characteristics, and cost. The present study suggested that lotus rhizome starch can be efficiently used as a starch source for various industrial applications. Oxidized, cross-linked, and dual-modified starches presented marked differences in physicochemical, crystallinity, thermal, morphological, and pasting characteristics, hence confirming successful modification. For dual-modified starches, cross-linking of starch followed by oxidation resulted in better pasting properties, thermal stability, and degree of modification than native, oxidized as well as cross-linked starches even at low oxidant concentration. Starches modified by dual chemical methods are commonly used in the food industry and are included as adsorbents of heavy metals in the non-food industry. Dual modification techniques overcome the drawbacks that occur in conventional modification, and can produce all the advantages when modified by the single method, which would gain considerable economic benefits and are promising trends for starch modification.
Acknowledgements
The authors also wish to acknowledge the Sophisticated Analytical Instruments Laboratories (SAI Lab), Thapar University, Patiala, India, National Institute of Pharmaceutical Education and Research (NIPER), Mohali, India, and Central Instrumentation Laboratory, Punjab University, Chandigarh, India, for helping in sample analysis.
Funding
The first author is grateful to the Ministry of Human Resource Development, India, for providing financial assistance in the form of institutional fellowship.
Additional information
Funding
References
- Hazarika, B.J.; Sit, N. Effect of Dual Modification with Hydroxypropylation and Cross-Linking on Physicochemical Properties of Taro Starch. Carbohydrate Polymers 2006, 140, 269–278.
- Das, A.B.; Singh, G.; Singh, S.; Riar, C.S. Effect of Acetylation and Dual Modification on Physic-Chemical, Rheological and Morphological Characteristics of Sweet Potato (Ipomoea batatas) Starch. Carbohydrate Polymers 2010, 80, 725–732.
- Halal, S.L.M.E.; Colussi, R.; Pinto, V.Z.; Bartz, J.; Radunz, M.; Carreno, N.L.V.; Dias, A.R.G.; Zavareze, E.D.R. Structure, Morphology and Functionality of Acetylated and Oxidized Barley Starches. Food Chemistry 2015, 168, 247–256.
- Zhou, F.; Liu, Q.; Zhang, H.; Chen, Q.; Kong, B. Potato Starch Oxidation Induced by Sodium Hypochlorite and Its Effect on Functional Properties and Digestibility. International Journal of Biological Macromolecules 2016, 84, 410–417.
- Sanchez-Rivera, M.M.S.; Suarez, F.J.L.G.; Valle, M.V.D.; Meraz, F.G.; Perez, L.A.B. Partial Characterization of Banana Starches Oxidized by Different Levels of Sodium Hypochlorite. Carbohydrate Polymers 2005, 62, 50–56.
- Gao, F.; Li, D.; Bi, C.H.; Mao, Z.H.; Adhikari, B. Preparation and Characterization of Starch Crosslinked with Sodium Trimetaphosphate and Hydrolyzed Enzymes. Carbohydrate Polymers 2014, 103, 310–318.
- Gutierrez, T.J.; Tapia, M.S.; Perez, E.; Fama, L. Structural and Mechanical Properties of Edible Films Made from Native and Modified Cush-Cush Yam and Cassava Starch. Food Hydrocolloids 2015, 45, 211–217.
- Liu, J.; Wang, B.; Lin, L.; Zhang, J.; Liu, W.; Xie, J.; Ding, Y. Functional, Physicochemical Properties and Structure of Cross-Linked Oxidized Maize Starch. Food Hydrocolloids 2014, 36, 45–52.
- Sukhija, S.; Singh, S.; Riar, C.S. Physicochemical, Crystalline, Morphological, Pasting and Thermal Properties of Modified Lotus Rhizome (Nelumbo nucifera) Starch. Food Hydrocolloids 2016c, 60, 50–58.
- Sukhija, S.; Singh, S.; Riar, C.S. Isolation of Starches from Different Tubers and Study of Their Physicochemical, Thermal, Rheological and Morphological Characteristics. Starch/Stärke 2016a, 68, 160–168.
- Wang, Y.J.; Wang, L. Physicochemical Properties of Common and Waxy Corn Starches Oxidized by Different Levels of Sodium Hypochlorite. Carbohydrate Polymers 2003, 52, 207–217.
- Smith, R.J.; Production and Uses of Hypochlorite Oxidized Starches (Vol. II). Starch Chemistry and Technology; Whistler, R.L.; Paschall, E.F.; Eds.; Academic Press: New York USA, 1967; 620–625.
- Kaur, L.; Singh, J.; Singh, N. Effect of Cross-Linking on Some Properties of Potato (Solanum tuberosum L.) Starches. Journal of the Science of Food and Agriculture 2006, 86, 1945–1954.
- Yamazaki, W.T.; An Alkaline Water Retention Capacity Test for the Evaluation of Cooking Baking Potentialities of Soft Winter Wheat Flours. Cereal Chemistry 1993, 30, 242–246.
- Medcalf, D.G.; Gilles, K.A. Wheat Starches, Comparison of Physicochemical Properties. Cereal Chemistry 1965, 42, 558–568.
- Morrison, W.R.; Laignelet, B. An Improved Colorimetric Procedure for Determining Apparent and Total Amylose in Cereal and Other Starches. Journal of Cereal Science 1980, 1, 19–35.
- Balasubramanian, S.; Sharma, R.; Kaur, J.; Bhardwaj, N. Characterization of Modified Pearl Millet (Pennisetum typhoides) Starch. Journal of Food Science and Technology 2011, 51, 294–300.
- Perera, C.; Hoover, R. Influence of Hydroxypropylation on Reterogradation Properties of Native, Defatted and Heat-Moisture Treated Potato Starches. Food Chemistry 1999, 64, 361–375.
- Nuwamanya, E.; Baguma, Y.; Emmambux, N.; Rubaihayo, P. Crystalline and Pasting Properties of Cassava Starch are Influenced by Its Molecular Properties. African Journal of Food Science 2010, 4, 8–15.
- Wurzburg, O.B.;. Converted Starches. Modified Starches: Properties and Uses; Wurzburg, O.B.; Ed.; 1986, CRC Press: Boca Raton, FL.
- Koo, S.H.; Lee, K.Y.; Lee, H.G. Effect of Cross-Linking on the Physicochemical and Physiological Properties of Corn Starch. Food Hydrocolloids 2010, 24, 619–625.
- Chavez-Murillo, C.E.; Wang, Y.J.; Bello-Perez, L.A. Morphological, Physicochemical and Structural Characteristics of Oxidized Barley and Corn Starches. Starch/Stärke 2008, 60, 634–645.
- Kaur, M.; Bhullar, G.K. Partial Characterization of Tamarind (Tamarindus indica L.) Kernel Starch Oxidized at Different Levels of Sodium Hypochlorite. International Journal of Food Properties 2015. 10.1080/10942912.2015.1038722.
- Sandhu, K.S.; Kaur, M.; Singh, N.; Lim, S. A Comparison of Native and Oxidized Normal and Waxy Corn Starches: Physicochemical, Thermal, Morphological and Pasting Properties. LWT- Food Science and Technology 2008, 41, 1000–1010.
- Lawal, O.S.; Composition, Physicochemical Properties and Reterogradation Characteristics of Native, Oxidized, Acetylated and Acid-Thinned New Cocoyam (Xanthomosoma sagittifolium) Starch. Food Chemistry 2004, 87, 205–218.
- Jyothi, A.N.; Moorthy, S.N.; Rajasekharan, K.N. Effect of Cross-Linking with Epichlorohydrin on the Properties of Cassava (Manihot esculenta Crantz) Starch. Starch/Stärke, 2006, 58, 292–299.
- Vanier, N.L.; Zavareze, E.D.R.; Pinto, V.Z.; Klein, B.; Botelho, F.T.; Dias, A.R.G.; Elias, M.C. Physicochemical, Crystallinity, Pasting and Morphological Properties of Bean Starch Oxidized by Different Concentrations of Sodium Hypochlorite. Food Chemistry 2012, 131, 1255–1262.
- Mirmoghtadaie, L.; Kadivar, M.; Shahedi, M. Effects of Cross-Linking and Acetylation on Oat Starch Properties Food Chemistry 2009, 116, 709–713.
- Kittipongpatana, O.S.; Kittipongpatana, N. Preparation and Physicochemical Properties of Modified Jackfruit Starches. LWT- Food Science and Technology 2011, 44, 1766–1773.
- Xiao, H.X.; Lin, Q.L.; Liu, G.Q.; Yu, F.X. A Comparative Study of the Characteristics of Cross-Linked, Oxidized and Dual-Modified Rice Starches. Molecules 2012, 17, 10946–10957.
- Sukhija, S.; Singh, S.; Riar, C.S. Effect of Oxidation, Cross-Linking and Dual Modification on Physicochemical, Crystallinity, Morphological, Pasting and Thermal Characteristics of Elephant Foot Yam (Amorphophallus paeoniifolius) Starch. Food Hydrocolloids 2016b, 55, 56–64.
- Spier, F.; Zavaraze, E.D.R.; Silva, R.M.E.; Elias, M.C.; Dias, A.R.G. Effect of Alkali and Oxidative Treatments on the Physicochemical, Pasting, Thermal and Morphological Properties of Corn Starch. Journal of the Science of Food and Agriculture 2013, 93, 2331–2337.
- Hoover, R.; Sosulski, F. Effect of Cross-Linking on Functional Properties of Legume Starches. Starch/Starke 1986, 38, 149–155.
- Zhang, Y.R.; Wang, X.L.; Zhao, G.M.; Wang, Y.Z. Preparation and Properties of Oxidized Starch with High Degree of Oxidation. Carbohydrate Polymers 2012, 87, 2554–2562.
- Kuakpetoon, D.; Wang, Y.J. Characterization of Different Starches Oxidized by Hypochlorite. Starch/Stärke 2012, 53, 211–218.
- Yook, C.; Pek, U.H.; Park, K.H. Gelatinization and Reterogradation Characteristics of Hydroxypropylated and Cross-Linked Rices. Journal of Food Science 1993, 58, 405–407.