ABSTRACT
It is expected to improve the utilization of potatoes and enhance the nutritional value of traditional staple food by adding a certain proportion of potato granules to wheat flour in developing noodles and bread. This research aimed to investigate the effects of potato granules on the rheological properties and microstructure of wheat flour dough samples. The rheological properties of dough samples with various proportions of potato granules (0, 20, 25, 30, 35, and 40 wt%) were determined using a rheometer. The typical steady flow curves of the dough samples exhibited shear-thinning behaviour. Frequency sweep tests revealed that the addition of the potato granules increased the storage and loss moduli (G′ and G′′) but decreased tanδ (G′′/G′). The creep-recovery curves indicated that the deformation of the dough samples decreased significantly as the proportions of potato granules increased. Compared with the control sample, the blended potato granule samples showed a delay in the dough gelatinization temperature, as demonstrated by temperature sweep tests. The rheological behaviour of the dough samples was correlated with the results obtained through scanning electron microscopy. Noodles added with 20% potato granules was suggested as the most appropriate recipe considering the textural analysis.
Introduction
Potato (Solanum tuberosum L.) is the world’s fourth most important food crop after rice, wheat, and maize.[Citation1] Potatoes can grow with stable yields under conditions unfavourable for the growth of other crops, such as less land and harsher climates. Moreover, potato cultivation produces a larger amount of dry matter and protein per hectare than cereal crop cultivation.[Citation2] On the other hand, it has been reported that potato contains numerous essential nutritional components, such as carbohydrates, proteins, minerals, dietary fibre, and antioxidants.[Citation2–Citation4] Compared with other vegetables and cereal products, potatoes contain high-quality proteins because the lysine content in potato is high, which is often insufficient in such crops.[Citation5] Because of its high yield and great nutritive value, potato has been considered as a vital food-security crop and substitute for cereal crop.[Citation6] In both developed and developing countries, potato is an economically important staple crop. It is estimated that by the year 2020, over two billion people will depend on it for food, feed, or income.
China is currently the world’s largest potato producer, with 93 million tons produced in 2012.[Citation7] However, the potatoes are mainly consumed in China as fresh vegetables, and the processing rate is only about 10%, which is far lower than the world’s average (50%). Moreover, there are only a few kinds of potato products in China, such as potato starch, potato granules, French fries, chips, and so on. Although the potato starches are usually used as material for food processing, the productions of potato starches are recently limited because of large quantities of potato starch wastewater (PSW), which is rich in proteins, minerals, and free amino acids.[Citation8,Citation9] Moreover, the disposal of PSW is costly because of its high polluting effect.[Citation10] Compared to potato starches, the potato granules contain more comprehensive nutritional ingredients and better organoleptic characteristics.[Citation11–Citation13] Because of its higher nutritional values and less polluting effects, the production of potato granules has increased steadily over the past few years.
To improve the utilization of potato granules and increase the dietary nutrient intake of residents, many researchers have attempted to develop Chinese traditional staple food products like noodles and steamed bread with addition of potato granules.[Citation14] The potato granule–wheat flour composite doughs are the most widely used raw material for the processing of potato staple food. However, with the addition of potato granules, the processing quality of composite doughs were changed and to explore the effect of potato granules on dough processing characteristics is of great importance.
Rheological property is a comprehensive evaluation of the viscoelasticity and mixing tolerance of dough; this property is also an important indicator to examine the processing properties of raw material powder.[Citation15] The rheological properties of dough not only determine the processing properties but also affect the quality of final products.[Citation16,Citation17] Many reports have focused on the morphological, thermal, and rheological properties of potato starch,[Citation18–Citation21] but those of potato granules have yet to be investigated. To achieve better designs of the formulation and processing conditions of food systems, in which potato granules and wheat flour are the major ingredients, the effects of potato granules on the rheological properties and microstructure of wheat flour dough samples have been investigated in this research.
Materials and methods
Materials
High gluten wheat was provided by the Institute of Crop Science of Chinese Academy of Agricultural Sciences. The wheat flour was produced by using a laboratory mill (The Brabender Quadrumat Senior), containing 11.44% moisture, 15.59% protein, 71.85% starch, and 0.66% ash. Shepody potatoes were provided by the Dingbian Science and Technology Bureau of Shaanxi Province, China. Potato granules were made from Shepody potatoes. There were 6.54% moisture, 7.73% protein, 69.09% starch, and 3.00% ash in the potato granules we made. All the contents above were based on dry matters of samples. Protein, moisture, and ash contents were determined via AOAC official methods.[Citation22] Starch content was determined using a total starch assay kit (Megazyme, K-TSTA 04/2009).
Potato granule preparation
The Shepody potatoes were thoroughly washed and cut in half. The shapes of the shepody potatoes we used are elliptical, and the semi-major axis of the potato profile is 7–8 cm, and the semi-minor axis is 4–5 cm. Clean potatoes were placed in a steamer for 30 min with a constant temperature of 105°C. After steaming, the potatoes were peeled and mashed. The mashed potatoes were then spread on trays in an oven and dried at a constant temperature of 65°C. These dried potatoes were further ground into smaller granules by using a superfine pulverizer (Q-500B, Bingdu, China) and sieved through a 150 μm mesh sieve.
Dough preparation
Dough samples were prepared using blended powder containing wheat flour and potato granules. Each sample contains different proportions of potato granules (20, 25, 30, 35, 40 wt%), based on the dough weight. The control sample was prepared using wheat flour without the addition of potato granules. The amount of the added water (61.80% dry flour basis) was determined using a Farinograph (Farinograph-AT, Brabender, Duisburg, Germany) to obtain a peak centred on the 500 Brabender unit line; in all of the dough samples, a constant amount of water was maintained.[Citation23] The blended flour and deionized water were added in the bowl and mixed at a constant temperature of 30°C, and the mixing rate was 63 rpm/min. The samples used in rheological experiments and microstructural observation were obtained from the inner part of the doughs.
Noodle preparation
Noodles were processed with blended flour containing wheat flour and potato granules. Each sample contains different proportions of potato granules (0, 20, 25, 30 wt%), based on the dough weight. When the additive amount of potato granules reached 35%, the dough failed to form. Thus, we evaluated the potato noodle quality with 0–30% potato granules addition. Using a multifunctional mixer (KM005, Kenwood, England), 250 g of raw material flour was dissolved in a certain amount of purified water in advance. The amount of water was determined by the different water contents in raw material flour to ensure that the water content in all dough samples achieved 35.8%.
The dough was packed in polyethylene bags and kept in a container at a constant temperature of 25°C. After resting for 60 min, the dough was pressed through a noodle-making machine (JMTD-168/140, Dongfu, China) with a roller gap of 4 mm. The dough sheet was folded and turned 90° before the next sheeting. The folding, turning, and sheeting procedures were repeated 10 times before resting the dough sheets for another 30 min. Subsequently, the dough sheets were rolled until the thickness reached 1 mm. The expanded dough sheets were cut into 7 mm width strands to make fresh noodles. Fresh noodle samples were subsequently dried in an artificial climate box (LT-ACC300, LEAD-TECH, China).
Rheological measurements
The rheological properties of the dough samples with various proportions of potato granules (0, 20, 25, 30, 35, 40%) were determined using a rheometer (AR2000ex, TA Instruments, American). Steady shear viscosity measurements, frequency sweep tests, and creep recovery tests were performed at 25°C by using a stainless steel plate with a diameter of 20 mm and a gap of 1 mm between plates. The outer edge of the sample was coated with silicone oil to minimize water loss during measurements.[Citation24] An equilibration time of 2 min was applied to all samples before the measurement was conducted; in this manner, residual stress could be relaxed. Temperature was controlled using a water bath system connected to a peltier system in the bottom plate, which can accurately control temperature during rapid heating and cooling. Strain sweep tests were carried out at 1 Hz to determine the linear viscoelastic region (LVR) of the dough samples. The viscoelastic properties, particularly storage modulus, loss modulus, and loss tangent, of the samples were determined in the linear viscoelastic region.
Steady shear viscosity measurement
The steady shear tests were programmed to increase the shear rate from 0.01 s−1 to 10 s−1 with 10 points per decade and the gap was set at 1 mm to measure the apparent viscosity of the dough samples. The viscosity values were obtained as a function of shear rate. The flow behaviour of the mixed dough systems was analysed using a power law equation.[Citation25,Citation26]
where σ(Pa) is the shear stress, K (Pa sn) is the consistency index, γ(s−1) is the shear rate, and n is the flow index.
Frequency sweep test
A frequency sweep test was conducted in the range of 0.1 Hz (0.6283 rad/s) to 10 Hz (62.83 rad/s) with 10 points per decade, and the controlled variable was set at 1% strain (within the linear viscoelastic region). Storage modulus (G′), loss modulus (G′′), and loss tangent (tanδ = G′′/G′) were obtained by changing the frequency. Power law models represented in Eqs. (2) and (3) were applied to describe the frequency dependence of G′ and G′′.[Citation27]
In Eqs. (2) and (3), K′, K′′, n′, and n′′ are the corresponding fitting parameters, and ω is the angular frequency (Hz). K′ and K′′ reflect the elastic and viscous properties, respectively. n′ and n′′ are referred to as the frequency exponents, which can provide useful information regarding the viscoelastic nature of materials.[Citation25]
Creep-recovery test
Constant stress (50 Pa) was applied on the dough samples for 5 min. Then the stress was removed, and strain recovery was allowed for another 5 min. The strain values were obtained as a function of time. The four-parameter Burger’s model has been used to predict the creep recovery behaviour of starch-based systems.[Citation28] This model is expressed in the following equations:
where ε is the strain (%), t is the time (s) after loading, σ0 is the stress (Pa), EM is the elastic modulus (Pa), and ηM is the viscous coefficient (Pa s) of Maxwell spring and dashpot. EK and ηK are the elastic modulus (Pa) and the viscous coefficient (Pa s) of Kelvin-Voigt spring and dashpot. Retardation time (τ) represents the time required for the deformation of 63.2% or (1-e−1) in the Kelvin unit. In Burger’s model [Eq.(4)], the creep rate (ε′) can be calculated using the following equation[Citation29]:
In the stable creep stage (t = ∞), the creep rate can be calculated when it asymptotically reaches a constant value, as described in the following equation:
Temperature sweep test
In the temperature sweep test, the dough samples were loaded onto the measuring apparatus with a strain of 2% and a frequency of 1 Hz in the linear viscoelastic region. The plate was programmed to increase the temperature from 30°C to 110°C at a heating rate of 5°C per min. G′, G′′, and tanδ were obtained as a function of temperature.
Scanning electron microscopy
The microstructure of the dough samples with different percentage of potato granules was observed using an S-570 scanning electron microscope (SEM) (Tokyo, Japan) with a 30-KV acceleration voltage. Samples were taken from the inner part of dough and then knead into oval shape before placed in 3% glutaraldehyde overnight (12 h). The samples were washed with 0.1 mol/L phosphate buffer thrice and dehydrated in a graded acetone series (25%, 50%, 75%, 80%, and 100%) for 20 min; afterward, the samples were critical point dried in CO2. Dehydrated dough samples were broken apart and added to double-sided adhesive tape mounted on an aluminium stub with the internal surface facing upward. After sprayed with gold, SEM was used to observe and photograph the samples at 1000× magnification.
Measurement of instrumental textural properties
Textural properties of cooked noodles including tensile characteristics and textural profile were determined using a Texture Analyser (TA-XT2i, Stable Micro System, England). Dried potato noodles (10 g) were added to 500 ml boiling water and cooked to the optimum cooking time. Noodle samples were taken every 10 s during boiling process and were squeezed by the slides. The optimum cooking time is the time when the white core in the noodles disappeared. Cooked noodles were cooled down using the running tap water for 30 s before testing. Tensile characteristics were analysed by the following conditions: probe: A/SPR; testing speed: 1 mm/s; return speed: 10 mm/s; data acquisition frequency: 25 p/s; trigger value: 5 g. The maximum tensile force before noodle broken was recorded. Textural profile analysis was carried out with the following conditions: probe: ALKB-F; testing speed: 0.8 mm/s; return speed: 10 mm/s; data acquisition frequency: 200 p/s; compression degree: 70%; time interval: 10 s; trigger value: 10 g. All results were calculated from the measurements of six samples.
Results and discussion
Steady shear properties
The typical steady flow curves of the dough samples in the presence or absence of potato granules are presented in . The apparent viscosity of the dough samples decreased as the shear rate increased, and the steady shear curves were almost parallel to one another. This result indicated that the samples exhibited shear-thinning behaviour, albeit at different levels. The shear thinning behaviour is attributed to the weakening of molecular network in dough systems due to applied shear strain.[Citation30] Similarly, shear thinning behaviour was also observed in other dough systems like wheat dough and gluten-free dough added with inulin.[Citation28,Citation31] shows the power-law-fitting parameters of steady flows. The flow behaviour index (n) is the reflection of the pseudoplasticity of the dough samples.[Citation30] As the proportion of potato granules increased, the flow behaviour index increased. This indicated that the potato granules reduced the pseudoplasticity of the dough samples. Similar phenomenon was also observed in other mixed dough samples, such as alfalfa-wheat dough.[Citation23] As the shear rate increased, the steady flow curves flattened out gradually after a steep fall occurred, and the viscosities approached a fixed value. Compared with the control sample, the apparent viscosity of the dough samples were increased when the potato granule proportions increased, and the consistency index (K) of the dough samples presents the same trend as shown in . Those results indicated that the viscosity of the dough samples increased with the addition of potato granules. It has been reported that the gelatinized starch in potato granules is highly viscous when this starch absorbs water. Furthermore, a high degree of macromolecular disorganization enhanced the solubility of gelatinized starches, which formed viscous pseudoplastics.[Citation30,Citation32]
Table 1. Effects of shear rate on the consistency index (K), flow behaviour index (n), and determination coefficient (R2) of the dough samples with potato granules at different proportions.
Frequency sweep test
Small-amplitude oscillatory dynamic test is an effective tool to examine the viscoelastic properties of dough.[Citation23] shows G′, G′′, and tanδ of the dough samples with different proportions of potato granules plotted against frequency at 25°C. G′ and G′′ of the samples were dependent on the oscillation frequency, which indicated a typical viscoelastic nature of the food matrix.[Citation29] G′ and G′ increased as frequency increased; therefore, the overall chain mobility within the network was relatively high.[Citation33] The mechanical spectra of the dough samples evaluated in this study displayed higher G′ than G′′ throughout the whole frequency range. It indicated that these dough samples exhibited dominant elastic behaviour compared to viscous behaviour whether or not the potato granules are present.[Citation27] As a dimensionless parameter, tanδ provides a direct measure of whether a specific material behaves as solid like or liquid like. The results showed that tanδ of the samples decreased as the proportion of the potato granules increased. It means that the elastic nature prevailed over the viscous nature; dough samples added with potato granules process higher solid-like behaviour instead of liquid-like behaviour. This phenomenon is probably caused by the gelatinized starch in the potato granules. During the processing of potato granules, gelatinization occurred on potato starch granules under the condition of high temperature steam system. The disrupted crystalline structure of starch granules could enhance the water absorption ability of dough samples.[Citation15] These gelatinized starch can produce a paste when rehydrated and behaves similar to a gum.[Citation30] Like gums, the gelatinized potato starch in dough samples imparts greater water holding characteristics to dough that ultimately results into firmer consistency.[Citation34]
Figure 2. Typical frequency dependence of G′ (a), G′′ (b), and tanδ (c) of dough samples with potato granules at different concentrations (■Control; ●20%; ▲25%; ▼30%; ◄35%; ►40%; — predicted line).
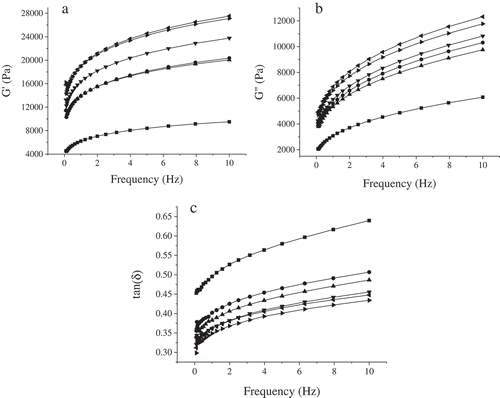
The power law parameters of samples affected by frequency are shown in . The determination coefficients of G′ and G′′ are >0.98, and so the power law model can basically represent the experimental data of G′ and G′′. The dough samples with the addition of potato granules yielded higher K′ and K′′ than the control sample. This finding indicated that G′ and G′′ increased as the proportion of the potato granules increased. K′ of the samples was higher than K′′, and this finding is consistent with the results shown in . The n′ and n′′ values of all dough samples were very close, indicating that these samples in the presence or absence of potato granules have similar frequency sensitivity.
Table 2. Effect of frequency on the consistency index (K′ and K′′), flow behaviour index (n′ and n′′), and determination coefficient (R2) of the dough samples with potato granules at different proportions.
Creep recovery test
The creep-recovery curves of the dough samples are shown in . The creep behaviours of the samples with different proportions of potato granules were quite different. The strain of the dough samples increased when the samples were subjected to a constant stress (50 Pa); by contrast, the strain decreased in the creep stage without stress. The addition of the potato granules decreased the maximum strain of the dough samples compared with the control samples. This means the deformation of samples added with potato granules is smaller than the wheat dough. represents the fitting parameters from Burger’s model of the dough samples. EM, ηM, and EK increased as the amount of the added potato granules increased. This is consistent with the results we obtained from the frequency sweep that both the elastic and viscous modulus increased with the addition of potato granules. also showed that τ decreased conversely as the amount of the added potato granules increased. This result indicated that the dough samples with the added potato granules can be deformed to a certain extent with a shorter time. As shown in , the creep rate in the stable stage (τ = ∞) and the recovery rate of the dough samples decreased as the amount of the potato granules increased. The smaller deformation and recovery rate of samples treated with potato granules might be caused by the solid-like nature of potato granules blend doughs. Thus, the presence of potato granules decreased the susceptibility of the dough samples to stress and also reduced the recovery rate. The results obtained via the creep recovery test are consistent with the previously described findings.
Table 3. Burger’s modelling parameters of the creep-recovery behaviour of the dough samples with potato granules at different proportions.
Temperature sweep test
shows G′, G′′, and tanδ as a function of temperature of the dough samples added with the potato granules at different proportions. As the temperature increased, G′ and G′′ of the dough samples decreased in the initial stage until a specific temperature was reached. This phenomenon showed a decreasing interaction in the dough systems. In this stage, the damaged starch released the absorbed water and reduced G′ and G′′, as affected by amylase at early heating temperatures.[Citation23] When the samples were heated to the gelatinization temperature, the temperature sweep oscillatory curves increased abruptly until the maximum viscoelastic values were reached. This increase was mainly attributed to starch gelatinization and protein denaturing.[Citation35] Gelatinization increased the elasticity and viscosity of the dough samples by changing the structure of the starch granules. During gelatinization, water initially penetrates the amorphous zone of the starch granules and initiates irreversible swelling; microcrystals melt and starch compounds composed of amylase leach.[Citation30] As the temperature further increased, G′ and G′′ decreased after the maximum viscoelastic values were obtained. This finding confirmed that starch granules break down when the maximum swelling is attained and the crystalline zones become disorganized and disrupted.
Figure 4. Typical temperature dependence of G′ (a), G′′ (b), and tanδ (c) of the dough samples with potato granules at different concentrations (■Control; ●20%; ▲25%; ▼30%; ◄35%; ►40%; — predicted line).
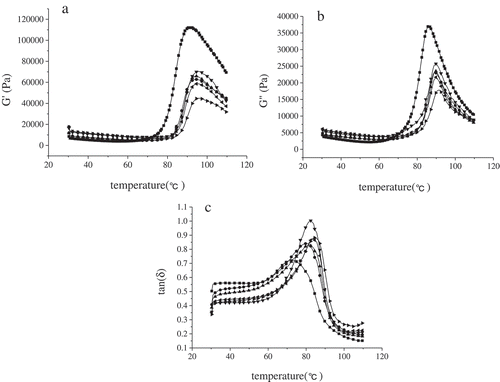
Tanδ against temperature is shown in ). Former study by Jekle defined a simple possibility to identify the gelatinization onset in oscillatory tests by the derivative of the loss factor.[Citation36] He revealed that the maximum of Tanδ in rheological measurements could be defined as the onset gelatinization temperature of samples. Thus, as the proportions of the potato granules increased (0%, 20%, 25%, 30%, 35%, and 40%), the gelatinization temperatures of the dough samples were 71.7, 79.7, 80.8, 82.4, 84, and 84.5°C, respectively. This phenomenon indicated that the presence of potato granules caused a delay in the dough gelatinization temperature. This happens mainly due to the disrupted crystalline structure of potato starch granules caused by gelatinization, and thus samples added with potato granules have stronger water absorption ability than the control sample.[Citation15] The existence of potato granules decreased the content of available water for wheat starch to swell and gelatinize because potato granules exhibit a strong water absorption capacity. Therefore, gelatinization was delayed.
Morphological properties
The microstructures of the dough samples with different concentrations of potato granules were observed using a SEM, as shown in . The wheat dough was characterized by the presence of small and large starch granules, which were densely distributed in a partially formed protein matrix.[Citation37] The wheat dough sample formed a more continuous gluten network and exhibited a distinguishable gluten film structure entrapping the starch granules. Compared with the samples added with potato granules, the control sample showed a more compact microstructure with fewer hollows and ditches. The starch granules in the control sample were tightly embedded in the membrane-like gluten matrix. However, a more roughly continuous gluten network with some cranks was observed on the surfaces of the dough samples with the addition of potato granules. When the additive amount of the potato granules reached 40% (), a hollow and porous structure was evident. The addition of the potato granules decreased the continuity of the protein coating on the dough surfaces and enlarged the hollows and voids. Although some portions of the gluten film could be observed, the starch granules were not completely wrapped and appeared less embedded in the protein matrix than the control sample.
Figure 5. Scanning electron micrographs of the dough samples with potato granules at different concentrations (A: 0%, B: 20%, C: 25%, D: 30%, E: 35%, F: 40%). SG: starch granule; GF: gluten film; HD: hollows and ditches.
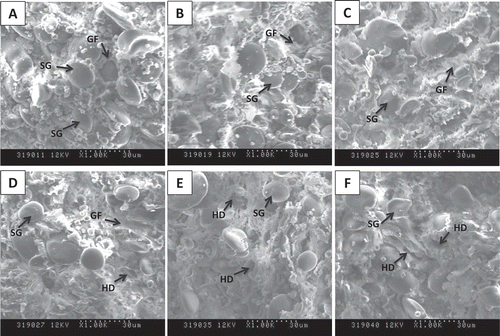
This phenomenon confirmed the absence of gluten in the potato granules, and the addition of the potato granules formed a relatively low degree of gluten network, which could not firmly envelope and adhere to the starch granules. Compared with the compact structure of the control sample, the structure of the samples added with the potato granules was disaggregated with thin fibril-like gluten. Furthermore, the lower quality of the gluten network could be attributed to the diminished elastic values of the samples with the potato granules. The SEM results are consistent with the rheological test findings.
Textural properties of noodles
The effect of potato granules proportion on noodle quality including tensile force, and TPA parameters were showed in . Results showed that the tensile force of noodles decreased with the increasing amount of potato granules, which means that noodles added with potato granules possess lower tolerance to tensile strength. TPA parameters showed that with the increasing proportion of potato granules, the hardness, cohesiveness, gumminess, and chewiness of doughs decreased significantly, while the adhesiveness increased. Additionally, noodles added with 20% potato granules were comparable with wheat noodles, because no significant difference was observed on tensile characteristic between these two kinds of noodles. However, when the proportion of potato granules reached 25%, the noodle quality decreased significantly, and when it reached 35%, the dough sheet failed to form. In general, the replacement of 20% of wheat flour by potato granules is the suggested recipe for potato noodles.
Table 4. Effect of potato granule proportions on tensile characteristic and TPA parameters of noodles.
Previous research showed that the textural properties of noodles and noodle doughs have a positive relationship with protein content and protein quality in flour.[Citation31] In this research, results obtained from tensile force and TPA parameters showed that the addition of potato granules showed a negative effect on the textural properties of noodles. This might be due to the lack of gluten in potato granules. Gluten proteins are the main proteins in flour, which can form a network and give dough the unique viscoelastic properties. Unlike wheat flour, potato granules do not have gluten. Moreover, the fibres, proteins, gelatinized potato starch, and other components in potato granules could dilute the gluten network in dough samples. Since potato granules would elicit a damaging effect on a gluten network structure, the network in noodles becomes even more fragile during cooking. Therefore, the addition of potato granules decreased the maximum tensile force of noodles, which also means that noodles added with potato granules are easier to break. The TPA parameters including hardness, cohesiveness, gumminess, and chewiness of noodles also decreased as a consequence of the broken network during cooking. Similarly, other studies on oat noodles also indicated that the addition of oat flour decreased the edible quality of noodles. This is also mainly caused by the lack of gluten in oat flour.[Citation38] The results from rheology and SEM indicated that dough samples added with potato granules produced diluting effect on gluten network, which can also support the results obtained from the textural properties of noodles.
Conclusion
This study demonstrated that the addition of different proportions of potato granules affected the rheological properties of dough systems. All of the dough samples exhibited shear-thinning behaviour and pseudoplastic properties. The addition of the potato granules increased the apparent viscosity of the dough samples. The frequency sweep tests revealed that the presence of the potato granules increased the storage modulus and loss modulus but decreased tanδ in the entire frequency range. The power law model was found to fit the flow curves of the elastic and viscous moduli, and the presence of the potato granules significantly affected the power law parameters. The creep-recovery curves indicated that the deformation and recovery rate of the dough samples decreased as the amount of the potato granules increased. Burger’s model predicted the experimental creep data reasonably well, and the corresponding parameters were also affected by the addition of the potato granules. Compared with the control sample, the potato-granule-blended samples showed a delay in the dough gelatinization temperature according to the temperature sweep tests. The microstructure of dough samples were observed through SEM, and the results showed that the addition of potato granules decreased the continuity of protein coating on dough surfaces. In addition, potato granules have a considerable effect on noodle quality. The addition of potato granules decreased the tensile force, hardness, cohesiveness, gumminess, and chewiness of noodles. Noodles added with 20% potato granules could preserve the overall quality of noodles, and this is suggested as the most appropriate recipe for potato noodles. This research could encourage the application of potato granules in dough systems. Further research is needed to improve the quality of the dough mixed with potato granules and wheat flour to produce high-quality potato-based nutritional staple foods.
Funding
The authors acknowledge financial support from the National Natural Science Foundation of China (Grant No: 31601507) and the Public Welfare Industry (Agriculture) Scientific Research Projects of China (Grant No: 201503001-2).
Additional information
Funding
References
- Ezekiel R.; Singh N.; Sharma S.; Kaur A. Beneficial Phytochemicals in Potato — a Review. Food Research International. 2013, 50, 487–496.
- Galdón B.R.; Mesa D.R.; Rodríguez E.M.R.; Romero C.D. Amino Acid Content in Traditional Potato Cultivars from the Canary Islands. Journal of Food Composition and Analysis. 2010, 23, 148–153.
- Singh J.; Kaur L.; Moughan P.J. Importance of Chemistry, Technology and Nutrition in Potato Processing. Food Chemistry. 2012, 133, 1091.
- Arun K.B.; Chandran J.; Dhanya R.; Krishna P.; Jayamurthy P.; Nisha P. A Comparative Evaluation of Antioxidant and Antidiabetic Potential of Peel from Young and Matured Potato. Food Bioscience. 2015, 9, 36–46.
- Bártová V.; Bárta J.; Brabcová A.; Zdráhal Z.; Horáčková V. Amino Acid Composition and Nutritional Value of Four Cultivated South American Potato Species. Journal of Food Composition and Analysis. 2015, 40, 78–85.
- Lutaladio N.; Castaldi L. Potato: The Hidden Treasure. Journal of Food Composition and Analysis. 2009, 22, 491–493.
- Li S.; Duan Y.; Guo T.; Zhang P.; He P.; Johnston A.; Shcherbakov A. Potassium Management in Potato Production in Northwest Region of China. Field Crops Research. 2015, 174, 48–54.
- Pu S.Y.; Qin L.L.; Che J.P.; Zhang B.R.; Xu M. Preparation and Application of a Novel Bioflocculant by Two Strains of Rhizopus sp. Using Potato Starch Wastewater as Nutrilite. Bioresource Technology. 2014, 162, 184–191.
- Waglay A.; Karboune S.; Alli I. Potato Protein Isolates: Recovery and Characterization of Their Properties. Food Chemistry. 2014, 142, 373–382.
- Vikelouda M.; Kiosseoglou V. The Use of Carboxymethylcellulose to Recover Potato Proteins and Control Their Functional Properties. Food Hydrocolloids. 2004, 18, 21–27.
- Kim E.J.; Kim H.S. Influence of Pectinase Treatment on the Physicochemical Properties of Potato Flours. Food Chemistry. 2015, 167, 425–432.
- Lamberti M.; Geiselmann A.; Conde-Petit B.; Escher F. Starch Transformation and Structure Development in Production and Reconstitution of Potato Flakes. LWT - Food Science and Technology. 2004, 37, 417–427.
- Rytel E. The Effect of Industrial Potato Processing on the Concentrations of Glycoalkaloids and Nitrates in Potato Granules. Food Control. 2012, 28, 380–384.
- Xu, F.; Hu, H.H.; Zhang C.J.; Huang F.; Zhang X.; Liu Q.N.; Dai X.F.; Zhang H. Effects of Different Types of Proteins on the Eating Quality of Potato Noodles. Modern Food Science and Technology (In Chinese). 2015, 31, 269–276.
- Dapčević Hadnađev T.; Pajić-Lijaković I.; Hadnađev M.; Mastilović J.; Torbica A.; Bugarski B. Influence of Starch Sodium Octenyl Succinate on Rheological Behaviour of Wheat Flour Dough Systems. Food Hydrocolloids. 2013, 33, 376–383.
- Ahmed J. Effect of Barley β-glucan Concentrate on Oscillatory and Creep Behavior of Composite Wheat Flour Dough. Journal of Food Engineering. 2015, 152, 85–94.
- Turbin-Orger A.; Shehzad A.; Chaunier L.; Chiron H.; Della Valle G. Elongational Properties and Proofing Behaviour of Wheat Flour Dough. Journal of Food Engineering. 2016, 168, 129–136.
- Cai X.; Hong Y.; Gu Z.; Zhang Y. The Effect of Electrostatic Interactions on Pasting Properties of Potato Starch/Xanthan Gum Combinations. Food Research International. 2011, 44, 3079–3086.
- Chung H.-J.; Li X.-Q.; Kalinga D.; Lim S.-T.; Yada R.; Liu Q. Physicochemical Properties of Dry Matter and Isolated Starch from Potatoes Grown in Different Locations in Canada. Food Research International. 2014, 57, 89–94.
- Parada J.; Aguilera J.M. Effect of Native Crystalline Structure of Isolated Potato Starch on Gelatinization Behavior and Consequently on Glycemic Response. Food Research International. 2012, 45, 238–243.
- Singh N.; Isono N.; Srichuwong S.; Noda T.; Nishinari K. Structural, Thermal and Viscoelastic Properties of Potato Starches. Food Hydrocolloids. 2008, 22, 979–988.
- AOAC (Association of Analytical Chemists). Official Methods of Analysis, 17th edn.; AOAC International: Gaithersburg, MD, USA, 2000.
- Hao C.C.; Wang L.J.; Li D.; Özkan N.; Wang D.C.; Chen X.D.; Mao Z.H. Influence of Alfalfa Powder Concentration and Granularity on Rheological Properties of Alfalfa-Wheat Dough. Journal of Food Engineering. 2008, 89, 137–141.
- Zhang X.; Tong Q.; Zhu W.; Ren F. Pasting, Rheological Properties and Gelatinization Kinetics of Tapioca Starch with Sucrose or Glucose. Journal of Food Engineering. 2013, 114, 255–261.
- Albano K.M.; Franco C.M.L.; Telis V.R.N. Rheological Behavior of Peruvian Carrot Starch Gels as Affected by Temperature and Concentration. Food Hydrocolloids. 2014, 40, 30–43.
- Witczak T.; Witczak M.; Ziobro R. Effect of Inulin and Pectin on Rheological and Thermal Properties of Potato Starch Paste and Gel. Journal of Food Engineering. 2014, 124, 72–79.
- Shi A.M.; Wang L.J.; Li D.; Adhikari B. Characterization of starch films containing starch nanoparticles. Part 2: viscoelasticity and Creep Properties. Carbohydrate Polymers. 2013, 96, 602–610.
- Juszczak L.; Witczak T.; Ziobro R.; Korus J.; Cieslik E.; Witczak M. Effect of Inulin on Rheological and Thermal Properties of Gluten-Free Dough. Carbohydrate Polymers. 2012, 90, 353–360.
- Meng Y.C.; Sun M.H.; Fang S.; Chen J.; Li Y.H. Effect of Sucrose Fatty Acid Esters on Pasting, Rheological Properties and Freeze–Thaw Stability of Rice Flour. Food Hydrocolloids. 2014, 40, 64–70.
- Fu Z.Q.; Che L.M.; Li D.; Wang L.J.; Adhikari B. Effect of Partially Gelatinized Corn Starch on the Rheological Properties of Wheat Dough. LWT - Food Science and Technology. 2016, 66, 324–331.
- Park; C.S.; Baik B.K. Relationship Between Protein Characteristics and Instant Noodle Making Quality of Wheat Flour. Cereal Chemistry. 2004, 81, 159–164.
- Gryszkin A.; Zięba T.; Kapelko M.; Buczek A. Effect of Thermal Modifications of Potato Starch on its Selected Properties. Food Hydrocolloids. 2014, 40, 122–127.
- Singh S.; Singh N. Relationship of Polymeric Proteins and Empirical Dough Rheology with Dynamic Rheology of Dough and Gluten from Different Wheat Varieties. Food Hydrocolloids. 2013, 33, 342–348.
- Yu L.J.; Ngadi M.O. Rheological Properties of Instant Fried Noodle Dough as Affected by Some Ingredients. Journal of the Science of Food and Agriculture. 2006, 86, 544–548.
- Salvador A.; Sanz T.; Fiszman S.M. Dynamic Rheological Characteristics of Wheat Flour–Water Doughs. Effect of adding NaCl, sucrose and yeast. Food Hydrocolloids. 2006, 20, 780–786.
- Jekle M.; Mühlberger K.; Becker T. Starch–gluten Interactions During Gelatinization and its Functionality in Dough Like Model Systems. Food Hydrocolloids. 2016, 54, 196–201.
- Liu R.; Xing Y.; Zhang Y.; Zhang B.; Jiang X.; Wei Y. Effect of Mixing Time on the Structural Characteristics of Noodle Dough Under Vacuum. Food Chemistry. 2015, 188, 328–336.
- Majzoobi M.; Layegh B.; Farahnaky A. Inclusion of Oat Flour in the Formulation of Regular Salted Dried Noodles and Its Effects on Dough and Noodle Properties. Journal of Food Processing and Preservation. 2014, 38, 48–58.