ABSTRACT
Meat extract is a by-product obtained from the cooking broth of meat processing, which is used as a flavoring agent in cooking and in pharmaceutical products. Information on the processing technology of meat extract and its corresponding thermodynamic properties have been seldom reported, making difficult the design of heat transfer processes with accuracy. Thus, the present work aimed to evaluate the boiling-point elevation (BPE) and specific heat (cp) of meat extract in the range of concentrations and temperatures found during its processing. Boiling point temperature was determined experimentally as a function of pressure and soluble solid concentration of meat extract. Different correlations based on Dühring’s rule, Antoine equation, and the model proposed by Crapiste and Lozano were obtained to represent the boiling point temperature and/or BPE in some processing conditions, resulting in good fitting accuracy. The representation of the experimental values of cp was evaluated by four empirical equations. The best fitting was acquired using a quadratic model simultaneously dependent on concentration and temperature of the meat extract. The close agreement between the experimental and predicted values reinforces the applicability of the resulting models for designing evaporation systems.
Introduction
Among the meat-producing countries, Brazil is one of the most important, with 8 million tons produced annually,[Citation1] and being the major exporter of concentrated meat extract. The meat extract is a by-product obtained from the cooking broth of meat processing, with yield production of 0.03 kg/kg of meat.[Citation2] This product is rich in iron, proteins, and amino acids, besides being the object of great demand by the pharmaceutical and food industries around the world.[Citation3]
Technically, the production of meat extract involves two or more unit operations. An example of meat extract process can be observed schematically in . Initially, the cooking meat by-product (meat juice) with 1.5 °Brix is subjected to a sterilization step, at 121°C during 3 min, in a plate heat exchanger fitted with a holding tube (). The concentration is carried out in a three-effect evaporation system, in which the meat juice is concentrated to 20 °Brix in the first effect, subsequently to 40 °Brix in the second effect, and finally up to 60 °Brix. The three evaporators can be used in direct flow or in countercurrent flow arrangement (). As soon as the evaporation process was finished, the meat extract is concentrated to 80 °Brix in a discontinuous process using a vacuum boiler.
Figure 1. Schematic representation of the meat broth concentration process: (a) sterilization step; (b) three-effect evaporation system.
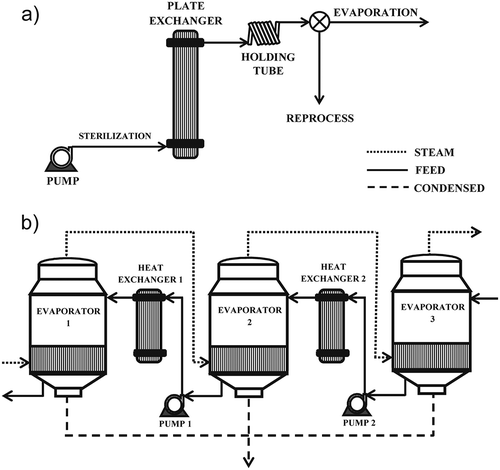
The exposure of foodstuffs, such as meat extract, to high temperatures for long time periods potentially causes changes in the color and flavor of the material, which, in some cases, can be acceptable or undesirable.[Citation4,Citation5] According to Bai et al.,[Citation6] the relatively low evaporation temperatures that prevail in vacuum evaporation imply that reasonable temperature differences can be maintained between the saturated steam used as the heating medium and the boiling liquid, while using relatively low steam pressures. These operating conditions limit the undesirable changes in the color and flavor of the product.[Citation7,Citation8] For aqueous liquids, the relationship between pressure and evaporation temperature may be obtained from thermodynamic tables or through theoretical and empirical relationships that correlate vapor pressure and temperature.[Citation5]
Although some physical and rheological properties of meat extract have been reported,[Citation9] the boiling-point elevation (BPE) and specific heat (cp) are properties needed in processes involving evaporation and heating. BPE describes the rise in boiling point of an aqueous solution as the soluble solid concentration increases, meaning that a solution with high concentration has a higher boiling point than one with low concentration. Some data on BPE, such as for coffee extract[Citation10] and blackberry juice,[Citation11] have been reported as a function of the water content and pressure.
Considering the importance of data on the thermodynamic properties of meat extract and the lack of published information on these values, the present work aimed to evaluate the boiling point temperature, BPE, and cp of meat extract in the range of concentrations, pressure, and/or temperatures found during its processing.
Material and methods
Raw material and sample preparation
Concentrated meat extract with 80 °Brix was obtained from the meat processing industry JBS–Friboi (Barretos, São Paulo, Brazil). The raw material was determined by Polachini et al.,[Citation9] where it was found 15.9% of water, 64.4% of protein, 19.1% of ash, and 0.6% of fat. For experimental procedures, concentrated meat extract was mixed with distilled water and stirred for 10 min, resulting in 14 samples with soluble solid concentration ranging from 1 to 60 °Brix.
Experimental procedures and mathematical analysis
Boiling point elevation
BPE of the meat extract samples was measured using an apparatus similar to that presented by Moresi and Spinosi[Citation12] as shown in . The apparatus is composed of a glass flat bottom flask (F) with three openings. Each sample (approx. 180 mL) was introduced into the flask by means of tube A and heated by an electric heater provided with a magnetic stirrer model TE-0852 (Tecnal, São Paulo, Brazil). Once the boiling temperature was reached, the sample was recirculated between tubes B and C. The liquid–vapor mixture released from the liquid surface flowed up through tube B, thus heating the thermocouple installed in the well, which was connected to a temperature transmitter model TT302 (SMAR, Sertãozinho, Brazil). Liquid particles were trapped in compartment D and returned to flask F, allowing vapor to enter the reflux condenser R. Condensed vapor also returned to flask F through tube C, being controlled by the flow rate in valve V to keep the extract concentration constant.
A vacuum pump in the condenser allowed pressure to vary in the range of 5.8 × 103–9.4 × 104 Pa (abs.), being measured by differential pressure transmitters model LD-301 (SMAR, Sertãozinho, SP, Brazil) in two different positions in the vacuum tube. Static pressure data were collected using a data acquisition system with accuracy of 0.1 Pa. In each experiment, the cooling water flow was initiated in the reflux condenser, the vacuum pump was turned on with a valve regulated to provide a pressure of about 6 × 103 Pa (abs.), and the fluid was mixed and heated slowly. Temperature and pressure were then continuously recorded, and the final values of boiling point associated with the pressure were registered after readings had been constant for at least 5 min. The procedure was repeated almost up to atmospheric pressure and allowed the measurement of boiling points at different pressures with the same extract concentration. Sample concentration was checked periodically and when the desired concentration was not reached, the procedure was repeated. The apparatus was calibrated according to Telis-Romero et al.[Citation10] and Gabas et al.,[Citation11] using NaOH and LiCl as standard solutions in different concentrations. The measured values were compared with those presented by Perry and Chilton.[Citation13] No significant differences were observed between the theoretical and experimental values at 95% confidence interval.
The experimental data of boiling point for each extract concentration were correlated with the boiling point of pure water at the same pressure through Dühring’s rule,[Citation14] expressed by Eq. (1).
where TA and TA0 are the boiling point temperature (K) of the meat extract and the boiling point temperature (K) of pure water, at the same pressure, respectively; and m0 and m1 are the model parameters. The values of TA0 were calculated by the Antoine equation (Eq. 2) using the constants for water: A = 18.3036; B = 3816.44; and C = −46.13:[Citation13]
where P (mmHg) is the experimental pressure corresponding to TA. Using the experimental data of TA in Eq. (2), it was also possible to correlate the boiling point temperature of meat extract with absolute vapor pressure, obtaining values of the Antoine constants A, B, and C, for each extract concentration. Moreover, it was possible to represent the elevation of boiling point of meat extract by an empirical model proposed by Crapiste and Lozano,[Citation15] simultaneously dependent on pressure and soluble solid concentration (Eq. 3).
In Eq. (3), ΔTB = TA - TA0 is the boiling point elevation (K) of the meat extract, W represents the mass concentration of soluble solids (ºBrix), P is the absolute pressure (Pa), and α, β, γ, and δ are the model parameters.
Specific heat
The cp (kJ/kg K) of the meat extract samples was measured in triplicate in the temperature range of 5°C–95°C by a differential scanning calorimeter using a DSC 8000 apparatus (Perkin Elmer, Shelton, USA). Calibration of the DSC apparatus was made previously with indium (m.p. 156.6°C, ∆Hf = 28.45 kJ/kg) at a heating rate of 10°C/min. The purge gas was nitrogen (99.5% purity) flowing at ~20 mL/min. Aluminum pans of 24.01 ± 0.04 mg (ref. 0219-0062, Perkin Elmer, USA) were used for determining the baseline and also as a holder for the reference material, the sapphire standard disk of 3-mm diameter[Citation16] (ref. 0219-1268, Perkin Elmer, USA), and the meat extract samples. Samples were sealed and weighed before and after the experimental procedures. Empty pans, reference material, and samples were subjected to the following thermal programs: isothermal at 0°C for 4 min, heating rate at 10°C/min up to 95°C, and isothermal at 95°C for 4 min, according to the procedures of the American Society for Testing and Materials ASTM-E1269 method.[Citation17] The software PYRIS 11.0 (Perkin Elmer, Shelton, USA) was used to analyze and plot the thermal data. The cp was calculated using Eq. (4):
where DS is the vertical displacement between the thermal curves of the sample and the reference material at a given temperature (mW), DST is the vertical displacement between the thermal curves of the sapphire standard disks at a given temperature (mW), WS is the sample mass (mg), WST is the sapphire standard disk mass (mg), and is the specific heat of the sapphire standard disk at a given temperature available in Archer[Citation16] with an accuracy of 0.0001 J∙g−1∙K−1. Mathematical modeling of cp data was carried out using empirical models depending on the soluble solid mass concentration and temperature given by
In Eqs. (5)–(8), α1, α2, α3, and α4 are the empirical model parameters, W is the soluble solid concentration (°Brix), and T is the temperature (K).
Statistical analysis
Regression analyses of BPE and cp data were carried out using nonlinear regressions tool of the software OriginPro 8.0 (OriginLab Corporation, Northampton, USA). The coefficient of determination (R2) and the mean relative error (MRE) were used to evaluate the quality of fitting and accuracy of the estimation, respectively. An F test was applied to verify the significant differences among the predicting models for cp. The simpler model with good representativeness was chosen to express cp as a function of the significant studied parameters.
Results and discussion
Boiling point elevation
The experimental values for the boiling point temperature of meat extract (TA) as a function of pressure and soluble solid concentration are presented in . In the pressure range of 3695.4–98173.9 Pa and meat extract concentrations from 1 to 60 °Brix, the experimental values of TA varied from 27.9°C to 106°C. In addition, the boiling point temperature of pure water (TA0) was calculated in the same pressure range (3695.4–98173.9 Pa), resulting in values between 27.72°C and 99.12°C.
Figure 3. A: Experimental values for boiling point temperature of meat extract (TA) as a function of pressure (P) and soluble solids concentration (W); B: dependence of TA on the boiling point of pure water at the same pressure (TA0) and W; C: dependence of meat extract vapor pressure (ln P) on TA and on W using Eq. (2); D: dependence of the boiling point elevation (∆TB) of meat extract P and on W using Eq. (3); E: dependence of the specific heat (cP) of meat extract on temperature (T) and on W using the best model fitted (Eq. 5).
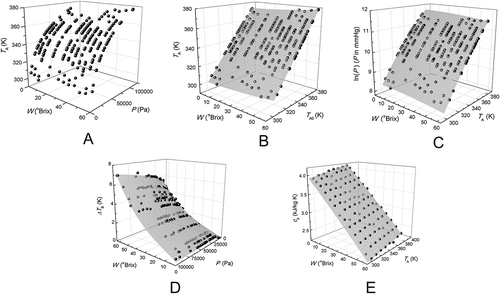
As expected, the boiling point of meat extract increased with increasing pressure and soluble solid concentration. Similar behavior was observed for TA0 values as a function of pressure. The higher values of TA when compared with TA0 at each level of pressure can be attributed to the mixture meat extract + water that present a little higher cp capacity than that of pure water. According to Darros-Barbosa et al.,[Citation18] foodstuff in aqueous solution have a stronger deviation from pure water boiling point temperature at higher concentrations, due to a relative increase of the cp capacity. This is probably related to the difference in the equilibrium isomeric composition of aqueous solutions of hydrated solutes; less water-compatible solutes would lead to a more disturbed hydration layer, and consequently to TA higher than ideality. Similar behavior was reported for products such as D-glucose and D-fructose solutions,[Citation19] blackberry juice,[Citation11] and aqueous solutions of mate.[Citation20]
To examine the relationship between the TA and TA0 values, Dühring’s rule (Eq. 1) was applied. The corresponding results for the model fitting and statistical analysis are presented in . There was a good agreement between the proposed model and the experimental data, as evidenced by the high values of R2 >0.999 and the low values of MRE <0.041%, obtained for all levels of meat extract concentration. The good accuracy of the linear model to predicting TA data from the theoretical data of TA0 at all concentration levels () indicates a strong reliability of the methodology used to measure this thermodynamic property. Furthermore, the values of the parameter m0 showed a tendency to decrease as the meat extract concentration increases, whereas the values of m1 increased as the meat extract concentration increases, in a similar trend to that reported by Gabas et al.[Citation11]
An alternative way of correlating the dependence of boiling point on pressure is based on extending the use of expressions suitable for describing the temperature dependence of pure water vapor pressure, as in the case of the Antoine equation (Eq. 2). The results of this model adjustment to the experimental data obtained for meat extract, at each soluble solid concentration studied, are also presented in . The statistical analysis indicated that this model can also be used to describe the experimental data, since the R2 values were higher than 0.999 and MRE (%) was lower than 0.108. The parameters A and C decreased when the concentration of meat extract increased, whereas parameter B increased with increasing concentration. This finding is in close agreement with the analogous results reported in the literature for similar products, such as coffee extract[Citation10] and solutions of mate.[Citation20] The goodness of the agreement between the experimental and theoretical values of ln(P) as a function of TA and concentration can be observed in , establishing an explicit dependence of the model constants as a function of soluble solids content of meat extract.[Citation12]
A third correlation is presented in terms of the rise in boiling point (∆TB, K) according to Eq. (3). shows a very good agreement of the model with the experimental data, revealed by a high determination coefficient (R2 = 0.991) as well as a low MRE of 14.19%. The accuracy of Eq. (3) for predicting the elevation of boiling point of meat extract can also be seen by the fitted surface shown in . The values of parameters α, β, γ, and δ shown in are in accordance with those published for other food products, such as coffee extract,[Citation10] apple juice and sugar solutions,[Citation15] and blackberry juice.[Citation11]
Table 2. Fitting parameters for the model correlating the boiling point elevation (∆TB) as a function of pressure and soluble solid concentration (Eq. 3).
In general, the three examined correlations, Dühring’s rule, the Antoine equation, and the Crapiste and Lozano equation, could be successfully used for describing the thermodynamic property TA as a function of pressure (P), meat extract concentration (W), and boiling point temperature of pure water (TA0). These correlations have great importance in the design and simulation of evaporation systems.
Specific heat
The experimental values of cp varied from 2.214 to 4.140 kJ/kg K in the temperature range of 5°C–95°C and meat extract concentration between 1.5 and 60 °Brix. These values are similar to those reported for apple,[Citation21] reconstituted milk,[Citation22] and acerola and blueberry pulps.[Citation23] When comparing the experimental values of cp for meat extract to those estimated by the classical method of Choi and Okos[Citation24] using the compositional data presented in item 2.1, an MRE of 6.57% was observed. Thus, linear and quadratic models were proposed to correlate the cp as a function of meat extract concentration (W) and temperature (T). Comparing the four models (Eqs. 5–8), the model that included the quadratic dependence on soluble solid concentration and linear dependence on temperature (Eq. 5) resulted in the best fitting with values of R2 = 0.997 and MRE = 0.657% () and significant difference at 95% when analyzing the variances among each model according to the F test, where the calculated F was much higher than the tabulated F for all comparisons.
Table 3. Fitting parameters for the proposed models correlating the specific heat (cp) as a function of temperature and soluble solid concentration.
shows the experimental of cp using the selected fitting model (Eq. 5), in which it is expressed the linear and quadratic dependence of cP on the meat extract concentration, and the linear behavior in relation to temperature. In addition, it is possible to state that the concentration effect is more prominent than the influence of temperature.
Conclusion
BPE and cp capacity for meat extract were determined experimentally at different conditions of concentration and temperature encountered in the processing plants. For the boiling temperature, a group of three correlations (Dühring’s rule, Antoine equation, and Crapiste and Lozano equation) was evaluated and all of them gave excellent adjustment results, although the Crapiste and Lozano model is recommended for practical purposes, since it includes the simultaneous dependence on pressure and soluble solid concentration. Four empirical equations were tested to fit the cp data as a function of temperature and meat extract concentration, and the model including quadratic dependence on soluble solid concentration and linear dependence on temperature presented the best statistical parameters, supporting the fitting procedure and being able to describe the heat-specific variations in a convenient form. The utility of the reported data is recommended in order to optimize the evaporation system design and performance, avoiding the underestimation of heat transfer areas and the overestimation of steam consumption. This objective can also be reached by the correct determination of global heat-transfer coefficients through the use of reported data along with the thermal conductivity and rheological properties of meat extract as a function of temperature and solid concentration.
Nomenclature
ΔTB | = | Boiling point elevation (K) |
ΔHf | = | Fusion enthalpy (kJ/kg) |
P | = | Pressure (mmHg; Pa) |
cp | = | Specific heat of the sample (kJ/kg K) |
cpst | = | Specific heat of the sapphire disk (kJ/kg K) |
DS | = | Vertical displacement between the thermal curves of the sample and the reference material at a given temperature (mW) |
DST | = | Vertical displacement between the thermal curves of the sapphire standard disk and the reference material at a given temperature (mW) |
WS | = | Sample mass used in the DSC calculation (mg) |
WST | = | Sapphire disk mass used in the DSC calculation (mg) |
W | = | Solids concentration (ºBrix) |
T | = | Temperature (K) |
TA0 | = | Pure water boiling point at a given pressure (K) |
TA | = | Boiling point of meat extract at a given pressure (K) |
A, B, C | = | Parameters of the Antoine equation |
MRE | = | Mean relative error (%) |
R2 | = | Coefficient of determination (dimensionless) |
m0, m1 | = | Parameters in Dühring’s rule (K; dimensionless) |
Greek symbols
α,β,γ,δ | = | Parameters in Crapiste and Lozano’s model |
α1, α2, α3, α4 | = | Parameters used in specific heat modeling |
Funding
The authors are grateful to the Sao Paulo Research Foundation, FAPESP (Grants 2013/09344-4 and 2010/09614-3), for the financial support.
Additional information
Funding
References
- IBGE. Estatística da Produção Pecuária; Instituto Brasileiro de Geografia e Estatística: Rio de Janeiro, 2016; 1–47.
- Santos, S.E.; Esteban, J.P. Extracto de carne: la invención de un químico. In Enseñanza y Divulgación de la Química y la Física, Cañon, G.P.; Sánchez, M.M.; Eds.; Ibergaceta Publicaciones: Madrid, 2012; 1–487.
- Banks, C.J.; Wang, Z. Treatment of Meat Wastes. In Waste Treatment in the Food Processing Industry, Wang, L.K.; Hung, Y.T.; Lo, H.H.; Yapijakis, C.; Eds.; Taylor & Francis Group: Boca Raton, 2014; 67–100.
- Brennan, J.G.; Butters, J.R.; Cowell, N.D.; Lilly, A.E.V. Food Engineering Operations; third ed. Elsevier Applied Science: London, 1990.
- Brennan, J.G. Evaporation and Dehydration. In Food Processing Handbook; Brennan, J.G., Ed.; WILEY-VCH Verlag GmbH & Co. KGaA: Weinheim, 2006; 71–124.
- Bai, L.; Lin, G.; Mu, Z.; Wen, D. Theoretical Analysis of Steady-State Performance of a Loop Heat Pipe with a Novel Evaporator. Applied Thermal Engineering 2014, 64, 233–241.
- Sampaio, K.L.; Biasoto, A.C.T.; Marques, E.J.N.; Batista, E.A.C.; Silva, M.A.A.P. Dynamics of the Recovery of Aroma Volatile Compounds during the Concentration of Cashew Apple Juice (Anacardium occidentale L.). Food Research International 2013, 51, 335–343.
- Yanniotis, S.; Tsitziloni, K.; Dendrinos, G.; Mallouchos, A. Aroma Recovery by Combining Distillation with Absorption. Journal of Food Enginerring 2007, 78, 882–887.
- Polachini, T.C.; Betiol, L.F.L.; Bastos, M.G.; Telis, V.R.N.; Souza, A.C.; Telis-Romero, J. Density, Thermal Expansion Coefficient, and Rheological Behaviour of Meat Extract Under Different Temperatures and Solids Concentrations. The Canadian Journal of Chemical Enginerring 2016, 94, 988–994.
- Telis-Romero, J.; Cabral, R.A.F.; Kronka, G.Z.; Telis, V.R.N. Elevation on Boiling Point of Coffee Extract. Brazilian Journal of Chemical Engineering 2002, 19, 119–126.
- Gabas, A.L.; Sobral, P.J.A.; Cardona-Alzate, C.A.; Telis, V.R.N.; Telis-Romero, J. Influence of Fluid Concentration on the Elevation of Boiling Point of Blackberry Juice. International Journal of Food Properties 2008, 11, 865–875.
- Moresi, M.; Spinosi, M. Engineering Factors in the Production of Concentrated Fruit Juices 1. Fluid Physical Properties of Orange Juices. International Journal of Food Science and Technology 1980, 15, 265–276.
- Perry, R.; Chilton, C. Manual de engenharia química; fifth ed. Guanabara Dois: Rio de Janeiro, 1986.
- Foust, A.S.; Wenzel, L.A.; Clump, C.W.; Maus, L.; Andersen, L.B. Principles of Unit Operations. John Wiley & Sons: New York, 1960.
- Crapiste, G.H.; Lozano, J.E. Effect of Concentration and Pressure on the Boiling Point Rise of Apple Juice and Related Sugar Solutions. Journal of Food Science 1988, 53, 865–868.
- Archer, D.G. Thermodynamic Properties of Synthetic Sapphire (α-A1203). Standard Reference Material 720 and the Effect of Temperature Scale Differences on Thermodynamic Properties. Journal of Physical and Chemical Reference Data 1993, 22, 1441–1453.
- ASTM. American Society for Testing and Materials; ASTM International: West Conshohocken, 2005.
- Darros-Barbosa, R.; Balaban, M.O.; Teixeira, A.A. Temperature and Concentration Dependence of Density in Model Liquid Foods. International Journal of Food Properties 2003, 6, 219–238.
- Maximo, G.J.; Meirelles, A.J.A.; Batista, E.A.C. Boiling Point of Aqueous D-Glucose and D-Fructose Solutions: Experimental Determination and Modeling with Group-Contribution Method. Fluid Phase Equlibria 2010, 299, 32–41.
- Rodriguesa, F.T.; Cardozo-Filho, L.; Zanoelo, E.F. Boiling Point of Aqueous Solutions of Mate (Ilex paraguariensis): Modeling and Simulation of a Batch Evaporator. Biosystems Engineering 2010, 107, 242–250.
- Mykhailyk, V.; Lebovka, N. Specific Heat of Apple at Different Moisture Contents and Temperatures. Journal of Food Engineering 2014, 123, 32–35.
- Reddy, C.S.; Datta, S.K. Thermophysical Properties of Concentrated Reconstituted Milk During Processing. Journal of Food Engineering 1994, 21, 31–40.
- Mercali, G.D. Physical Properties of Acerola and Blueberry Pulps. Journal of Food Engineering 2011, 106, 283–289.
- -Choi, Y.; Okos, M.R. Effects of Temperature and Composition on the Thermal Properties of Foods. In Food Engineering and Process Aplications; Lemauguer, M.J.; Eds.; Elsevier Applied Science Publishers: London, 1986, 93–101.