ABSTRACT
Natural antioxidants have received considerable research interest nowadays, especially their usage in oil products. Blueberry anthocyanin extracts were added to crude camellia seed oil and lipophilized with free fatty acids in the oil through enzymatic process. The results showed that lipophilized anthocyanin derivates were synthesized, the oxidative stability of the oil under high temperature was improved, and the content of free fatty acids of the crude camellia seed oil was decreased. In conclusion, enzymatic lipophilization of blueberry extracts with free fatty acids in crude camellia seed oil could improve the quality of oil, especially the performance under high temperature.
Introduction
Camellia seed oil is a kind of nutritious edible oil, being rich in unsaturated fatty acids, especially oleic acid.[Citation1] But just because of the high content of unsaturated fatty acids, the oil is easy to be oxidized. The oxidation of oil causes the generation of off-flavours and the loss of potential benefits and conduces to the development of potentially toxic compounds that may harm consumers’ health. Therefore, preventing the oxidation of oil before being consumed is the foremost challenge to maintain their benefits. Researchers have proposed different methods and strategies to delay lipid oxidation, and the use of antioxidants can be one of the most efficient means.[Citation2]
The traditional synthetic antioxidants, such as tert-butylhydroquinone (TBHQ), butylated hydroxytoluene (BHT), and butylated hydroxyanisole (BHA), may generate great antioxidant efficacy at accelerated storage temperatures, but they perform poorly during high-temperature cooking process.[Citation3] Furthermore, due to their potential detrimental effects on health such as carcinogenic and toxic effects, their utility has been strictly limited and regulated.[Citation4,Citation5] Under these circumstances, more attention has been paid to natural bioactive extracts from plants and fruits.[Citation6–Citation8] To date, many reports on the effects of natural antioxidant extracts in edible oil have been published. Asefi[Citation9] used pennyroyal (Mentha piperita) extracts as natural antioxidants for stabilizing sunflower oil. Preparation of phenolic extracts from rosemary, banana peel, and bamboo-leaf and their effects on the oxidative stability of edible oil have also been reported.[Citation10–Citation12]
Anthocyanins are proved to be powerful antioxidants and beneficial for health,[Citation13–Citation15] but the hydrophilic nature limits their usage in lipophilic systems. Earlier studies suggested that the enzymic acylation of anthocyanins with phenolic acids could increase their hydrophobicity and lipid solubility.[Citation16,Citation17] Recent studies showed that enzymatic lipophilization was an effective method to expand the application of natural phenolic compounds in lipophilic system, such as the recently reported enzymatic lipophilization of rowanberry phenolic extracts and epigallocatechin gallate, and the antioxidative activity of the lipophilic derivatives in edible oil was also evaluated.[Citation18–Citation21]
Crude camellia seed oil always contains free fatty acids, and those free fatty acids were always removed through alkali deacidification during traditional refining steps to ensure the quality of the oil.[Citation22] But these deacidification steps always do harm to the bioactive compounds such as tocopherol and cause a loss of oil.[Citation23,Citation24] In this study, blueberry anthocyanin extract was lipophilized with the free fatty acids in crude camellia oil, in order to, on the one hand, synthesize lipophilized anthocyanin derivatives and obtain oxidative stable camellia seed oil without synthetic antioxidants and, on the other hand, reduce free fatty acid content and avoid the disadvantages of traditional deacidification steps.
Materials and methods
Materials
Crude camellia seed oil (squeezed oil without refining) and camellia seed oil product for commercial sale were gifts from Anhui Huayin Camellia Oil Co., Ltd. (Anhui, China). Blueberry anthocyanin extract was obtained from Xi’an Changyue Phytochemistry Co., Ltd. (Xi’an, China). This extract consisted of 94.3% anthocyanin, which mainly contained Malvidin-3-galactoside (28.3%), Cyanidin-3-galactoside (24.0%), Delphinidin-3-galactoside (14.1%), Malvidin-3-glucoside (12.5%), and Cyanidin-3-arabinoside (7.2%). Novozym 435 (Candida antarctica lipase B, 10000 PLU g−1) was obtained from Novozymes (Bagsvaerd, Denmark). HPLC-grade solvents used for LC-MS were obtained from Tedia Company, Inc. (Fairfield, USA). 4A molecular sieves and other general chemicals used in this study were obtained from Xilong Scientific Co., Ltd. (Guangdong, China).
Pretreatment of original crude camellia seed oil
The original crude camellia seed oil was squeezed oil without any further treatment. We preceded some pretreatment in order to remove the gums and waxes before the lipophilization step. This process was performed according to the methods of Lee et al.[Citation25] and Nergiz et al.[Citation26] with modifications. At the beginning, 5% (w/w) water was added to the original crude camellia seed oil, kept at 70°C with continuous slow agitation for 40 min, and then the gums were separated by centrifugation. The oil was then stored at 4°C for 13 h and centrifuged at 2000 g for 10 min. After this process, the oil was the designated crude camellia seed oil and used in the following experiment.
Preparation of lipophilized oil
Blueberry anthocyanin solution was prepared by dissolving 0.5 g blueberry anthocyanin extract in 1.0 g polyxoyethylene stearate. Then, 36 mg of the blueberry anthocyanin solution, 10 mg of Novozym 435, and 2 g of 4A molecular sieves to 10 g of crude camellia seed oil were added in a sealed glass vessel and then placed in a 50°C orbital shaker at 200 rpm for 36 h. After that, 1 mL of deionized water was added to remove the redundant blueberry anthocyanin, stirred mildly, and then centrifuged at 2000 g for 5 min, and the water layer was discarded. The lipophilized oil was finally obtained. At the same time, two control groups were prepared: crude camellia seed oil with only addition of blueberry anthocyanin was prepared as control group 1, and crude camellia seed oil with only addition of Novozym 435 and molecular sieves was also prepared as control group 2. Because of the poor solubility of anthocyanin in oil, there was some deposit in the bottom of control group 1, and all the following experiments were done after centrifugation and separation of the deposit.
Measurement of acid value
The measurement of AV of crude camellia seed oil, control groups, and the lipophilized oil was carried out by using Chinese national standard (GB/T 5530–2005). In brief, 3 g oil sample was put in an oil sample bottle, and then 50 mL of hot neutralized alcohol and 2 mL of phenolphthalein indicator solution were added. The mixture was titrated with potassium hydroxide solution (0.05 mol L−1) and shaken vigorously until the appearance of the first permanent pink colour.
LC-MS analysis
The LC-MS was performed according to Lohachoompol et al.[Citation27] with modifications. Anthocyanins and their derivates in crude camellia seed oil, control groups, and lipophilized oil samples were analysed using a Waters Premier XE HPLC pump equipped with a Waters 996 photodiode array detector (PDA) (Waters Corp., Milford, MA, USA). The samples were analysed at 25°C on a Waters X Bridge C18 column (5 μm; 250 × 4.6 mm; Waters Corp., Milford, MA, USA) using a mobile phase consisting of solvent A (5% formic acid in water) and solvent B (acetonitrile) at the flow rate of 0.8 mL/min with the gradient as follows: 5% B (0 min), 10% B (15 min), 13% B (30 min), 20% B (34 min), 25% B (40 min), and 100% B (43 min). The injection volume was 10 μL, and detection was carried out at 530 nm. ESI mass spectra were scanned from m/z 100 to 1500. Spectra were operated in the positive ion mode. N2 was used as drying gas, with the temperature of 350°C and flow rate of 12 L/min. The nebulizer gas pressure was set at 0.31 MPa, and the capillary voltage was maintained at 4500 V. The MS spectra were analysed using Agilent Chemstation Rev.A.09.01 software (Agilent, Palo Alto, CA).
The free fatty acids in crude camellia seed oil, control groups, and the lipophilized oil were also analysed, and the analysis was done as described by Persson et al.[Citation28] with modifications. The samples were analysed at 25°C on a Waters C18 column (2.7 μm, 150 × 2.1 mm, Waters Corp., Milford, MA, USA) using a mobile phase consisting of solvent A (80% acetonitrile, 20% 0.5 mmol/L ammonium acetate) and solvent B (99% acetonitrile, 1% 0.5 mmol/L ammonium acetate) at the flow rate of 0.4 mL/min with the gradient as follows: 55% B (remain from 0 to 8 min), 95% B (8.5 min), 95% B (remain from 8.5 to 10 min), 55% B (10.5 min), 55% B (remain from 10.5 to 12 min). The injection volume was 10 μL. The mass spectrometer settings were: N2 as drying gas with the temperature of 350°C and flow rate of 12 L/min, the capillary voltage 4500 V, and the nebulizer gas pressure 0.31 MPa. Quantification of free fatty acids and anthocyanins was achieved by external calibration curve using the respective standards.
Preparation of heated oil samples
The five kinds of oils, crude camellia seed oil, lipophilized oil, commercial camellia seed oil, and two control groups were placed in a 180°C electric oven (Debao, model DFL-11, Guangzhou, China) for 4 h for heating study. Samples were taken every half hour. The samples were stored in a refrigerator after cooled to ambient temperature for further analysis.
Measurement of heated oil samples
To estimate the oxidative stability of the oil samples, the peroxide value (PV), the anisidine value (AnV), and the total oxidation value (TOTOX value) were measured. The PV was measured by using the Chinese national standard (GB/T 5538–2005). The AnV was measured by using the Chinese national standard (GB/T 24304–2009). The TOTOX value was calculated according to the formula: TOTOX = AnV+2PV.[Citation29]
Statistical analysis
All the measurements were carried out in triplicate, and mean values and standard derivations are shown in the figures and tables when appropriate. Figures were prepared by Origin software (version 8.0, OriginLab Corp., Northampton, MA, USA).
Results and discussion
LC-MS analysis
The anthocyanins in the crude camellia seed oil, the control groups, and the lipophilized oil were also analysed by LC-MS, and the retention times and characteristic fragments are shown in , and their contents in the oil samples are shown in . The results in showed that the crude camellia seed oil contained only tiny amount of anthoyanins and could barely be detected, and there was only a very small part of the anthocyanins dissolved and left after centrifugation in the control group 1. For the lipophilized oil, most part of the redundant blueberry anthocyanins added before the lipophilization process was removed, and the rest part of the anthocyanins and the lipophilization products were detected.
Table 1. Retention time (RT) and characteristic fragment ions of the anthocyanins and their derivatives in the oil samples.
Table 2. Content of anthocyanins in crude camellia seed oil, control groups, and the lipophilized oil.
The mass spectra of the lipophilization products are shown in . Identification and confirmation of the products were achieved by analysis of the full scan and the relative MSn data for each of the peaks. For the reaction product at RT 29.435 min (), the full scan data showed an ion at m/z 713.8, representing the molecular ion of Cyanidin-3-galactoside oleate. The MS2 data showed an ion at m/z 448.4, −265.4 Da from the molecular ion (m/z 713.8 M−1) and were consistent with loss of a oleic moiety. The MS3 data showed an ion at m/z 286.2, corresponding to a loss of 162.2 Da to the ion at m/z 448.4 and being consistent with loss of a galactoside moiety. On the basis of the full scan and fragmentation ions, the compound was confirmed to be Cyanidin-3-galactoside oleate. For the reaction product at RT 31.156 min, whose mass spectra are shown in , since the analysis was similar to that of Cyanidin-3-galactoside oleate, this compound was finally confirmed to be Cyanidin-3-galactoside palmitate. The confirmation of the two lipophilization products suggested that the lipophilization process was successfully proceeded and Cyanidin-3-galactoside esters were synthesized. The structure of Cyanidin-3-galactoside and its derivatives are shown in .
Figure 1. Mass spectrum (full scan, MS2, MS3) of (A) Cyanidin-3-galactoside oleate and (B) Cyanidin-3-galactoside palmitate.
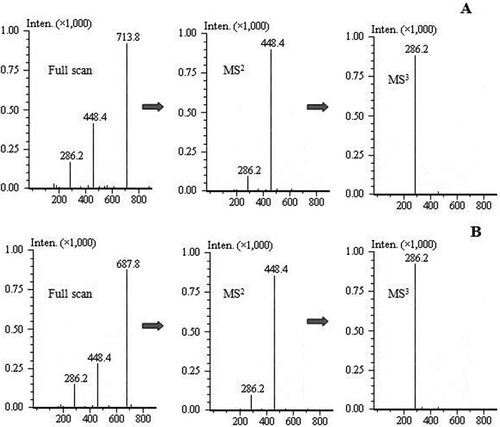
The free fatty acids in the crude camellia seed oil, the control groups, and the lipophilized oil were analysed by LC-MS, and the results are shown in . The crude camellia seed oil was rich in free fatty acids, in which the oleic acid (C18:1) was the most abundant kind. Palmitic acid (C16:0), stearic acid (C18:0), and linoletic acid (C18:2) were also the main components of its free fatty acids. Besides, in the two control groups, both the type and content of the free fatty acids were almost the same as that of the crude camellia seed oil. This showed that the enzyme itself didn’t affect the free fatty acids and that lipophilized anthocyanin derivatives won’t be produced without the enzyme. The free fatty acids in lipophilized oil, as is shown in the table, differed obviously on the content. The lowered palmitic acid (C16:0) and oleic acid (C18:1) indicated that these two acids played an important role in the lipophilization process and might participate more in the reaction, and this was proved by the content of anthocyanin derivates in . On the other hand, the acid values (AVs) of the crude camellia seed oil, the control group, and the lipophilized oil were 3.69, 3.67, and 2.54 mgKOH g−1, respectively (equivalent of 0.00657, 0.00654, and 0.00453 mmol g−1 oleic acid, respectively). The decrease of AV in lipophilized oil indicated the decrease of free fatty acids content, suggesting that the lipophilization process could be an effective way to reduce the free fatty acids. In addition, the AV of lipophilized oil met the Chinese standard for commercial camellia seed oil (GB/T 11765–2003).
Table 3. Free fatty acid composition of the crude camellia seed oil, the control groups, and the lipophilized oil.
As we can see in , in 10 g of the lipophilized oil, palmitic acid decreased 0.91 mg (0.00355 mmol), and oleic acid decreased 3.71 mg (0.0131 mmol). As is shown in , 9.19 mg (0.0129 mmol) of cyanidin-3-galactoside oleate and 2.40 mg (0.0036 mmol) of cyanidin-3-galactoside palmitate were synthesized. We added 12 mg blueberry anthocyanins before the lipophilization process, and after the lipophilization process, about 62% of the added blueberry anthocyanins were turned into derivates, as we can calculate according to the results. The results also showed that almost all the reduced free fatty acids were esterified to cyanidin-3-galactoside oleate and cyanidin-3-galactoside palmitate, and tiny amount of other derivates may also exist.
Comparison of oxidative stability indexes of the heated oil samples
The oxidative stability indexes, including PV, AnV, and TOTOX value, were measured and compared through the four kinds of heated oil samples (the crude camellia seed oil, the lipophilized oil, the commercial camellia seed oil, and the control group 1) to find the difference. The multiple parameters were applied to make a comprehensive evaluation of the high-temperature performance of the oil samples. Control group 2 was not included in the heating study because its components were almost the same as the crude camellia seed oil.
PV determines the primary oxidation products in oil, mainly hydroperoxides, and reflects the oxidation status in the early phase. The PV of the crude camellia seed oil, the lipophilized oil, the commercial camellia seed oil, and the control group 1 were measured and compared (). As we can see, PV of the oil samples kept increasing during the 4 h heating procedure, and PV of the crude camellia seed oil increased most sharply, which indicated the drastic oxidation degradation in the oil. Comparison between control group 1 and other samples showed that anthocyanin directly added in the oil could protect the oil from oxidation, but the protection is not effective enough because of the poor solubility of anthocyanin. PV of the lipophilized oil, as we can see in , increased moderately and kept at a comparatively low level, and this suggested that the lipophilized oil is more stable under high temperature and that the lipophilized blueberry anthocyanin derivates play an important role in postponing the oxidation of oil. However, the primary oxidation products were unstable and easy to decompose under high temperature, so AnV and TOTOX value were also measured to make the result sufficient enough to evaluate oil oxidation.
AnV reflects the amount of secondary decomposition products of the primary oxidation products, and the secondary decomposition products are mainly carbonyl and aldehydic compounds such as ketones and aldehydes. The AnV of the crude camellia seed oil, the lipophilized oil, the commercial camellia seed oil, and the control group 1 were shown in . The AnV of the oil samples increased continuously throughout the 4 h heating process, and obviously, the lipophilized oil performed better than the control group 1 and the commercial camellia seed oil, which implied that the lipophilized athocyanin derivates were conducive to the antioxidative stability of the oil sample.
The PV and AnV are combined to calculate the TOTOX value by the formula: TOTOX value = 2PV + AnV, which is always used to evaluate the oxidative rancidity of oils. The TOTOX value of the oil samples was shown in . The TOTOX value kept increasing during the heating process, and the increase rate of the samples was as follows: for the crude camellia seed oil, its TOTOX value increased from 9.35 to 72.74, at a rate of 169.5% h−1; for the lipophilized oil, the TOTOX value increased from 6.94 to 31.66, at a rate of 89.0% h−1; and for the commercial camellia seed oil, the TOTOX value increased from 8.26 to 50.51, at a rate of 127.9% h−1; for control group 1, the increase rate was 147.8%. Besides, in the industrial evaluation classification of oils, oils with TOTOX value below 32 were suitable for human consumption, and those higher than 32 may not be acceptable.[Citation30] The results showed that the crude camellia seed oil and the control group 1 should be rejected after 1.5 h at high temperature, and commercial camellia seed oil could be acceptable after heating for 2.5 h, whereas the lipophilized oil was still edible after heating for 4 h. Both the increase rate and the time to reach 32 of the TOTOX value in different oil samples indicated that the lipophilized oil is more resistant to oxidative rancidity and may be more suitable for high-temperature cooking.
Conclusion
In this study, lipophilization of blueberry anthocyanins with free fatty acids in crude camellia seed oil was conducted, and the influences of the lipophilized anthocyanin derivates in the oil were investigated by measuring oxidative stability indexes of the heated oil samples. After the lipophilization process, the content of free fatty acids was lowered to the quality requirements of commercial camellia seed oil, the synthesis of Cyanidin-3-galactoside oleate and Cyanidin-3-galactoside palmitate was confirmed by LC-MS, and the oxidative stability under high temperature was improved. The results showed that the lipophilized oil performed better than the crude camellia seed oil and the commercial camellia seed oil, suggesting that the lipophilized anthocyanin derivates could help improve the quality of oil and that the lipophilization process may be a good method to reduce the free fatty acid content.
Additional information
Funding
References
- Li, H.; Zhou, G. Y.; Zhang, H. Y.; Liu, J. A. Research Progress on the Health Function of Tea Oil. Journal of Medicinal Plants Research 2011, 5, 485–489.
- Shahidi, F.; Zhong, Y. Lipid Oxidation and Improving the Oxidative Stability. Chemical Society Reviews 2010, 39, 4067–4079.
- Taghvaei, M.; Jafari, S. M. Application and Stability of Natural Antioxidants in Edible Oils in order to Substitute Synthetic Additives. Journal of Food Science and Technology 2015, 52, 1272–1282.
- Branen, A. L.;. Toxicology and Biochemistry of Butylated Hydroxyanisole and Butylated Hydroxytoluene. Journal of the American Oil Chemists’ Society 1975, 52, 59–63.
- Botterweck, A. A. M.; Verhagen, H.; Goldbohm, R. A.; Kleinjans, J.; Van Den Brandt, P. A. Intake of Butylated Hydroxyanisole and Butylated Hydroxytoluene and Stomach Cancer Risk: Results from Analyses in the Netherlands Cohort Study. Food and Chemical Toxicology 2000, 38, 599–605.
- Quideau, S.; Deffieux, D.; Douat-Casassus, C.; Pouységu, L. Plant Polyphenols: Chemical Properties, Biological Activities, and Synthesis. Angewandte Chemie International Edition 2011, 50, 586–621.
- Aladedunye, F.; Matthäus, B. Phenolic Extracts from Sorbus aucuparia (L.) and Malus baccata (L.) Berries: Antioxidant Activity and Performance in Rapeseed Oil during Frying and Storage. Food Chemistry 2014, 159, 273–281.
- Aladedunye, F. A.;. Natural Antioxidants as Stabilizers of Frying Oils. European Journal of Lipid Science and Technology 2014, 116, 688–706.
- Asefi, N.;. Stabilization of Sunflower Oil by Pennyroyal (Mentha Piperita) Extracts during Accelerated Storage. International Journal of Food Properties 2017, 20, 30–40.
- Sayyad, R.; Jafari, S.; Ghomi, M. Thermoxidative Stability of Soybean Oil by Natural Extracted Antioxidants from Rosemary (Rosmarinus officinalis L.). International Journal of Food Properties 2017, 20, 436–446.
- Kurhade, A. H.; Waghmare, J. S. Effect of Banana Peel Oleoresin on Oxidative Stability of Sunflower and Soybean Oil. Journal of Food Processing and Preservation 2015, 39, 1788–1797.
- Ma, X.; Yan, R.; Yu, S.; Lu, Y.; Li, Z.; Lu, H. Enzymatic Acylation of Isoorientin and Isovitexin from Bamboo-Leaf Extractswith Fatty Acids and Antiradical Activity of the Acylated Derivatives. Journal of Agricultural and Food Chemistry 2012, 60, 10844–10849.
- Huang, D.; Ou, B.; Prior, R. L. The Chemistry behind Antioxidant Capacity Assays. Journal of Agricultural and Food Chemistry 2005, 53, 1841–1856.
- Galli, R. L.; Bielinski, D. F.; Szprengiel, A.; Shukitt-Hale, B.; Joseph, J. A. Blueberry Supplemented Diet Reverses Age-Related Decline in Hippocampal HSP70 Neuroprotection. Neurobiology of Aging 2006, 27, 344–350.
- You, Q.; Wang, B.; Chen, F.; Huang, Z.; Wang, X.; Luo, P. G. Comparison of Anthocyanins and Phenolics in Organically and Conventionally Grown Blueberries in Selected Cultivars. Food Chemistry 2011, 125, 201–208.
- Glӓssgen, W. E.; Seitz, H. U. Acylation of Anthocyanins with Hydroxycinnamic Acids via 1-O-Acylglucosides by Protein Preparations from Cell Cultures of Daucus Carota L. Planta 1992, 186, 582–585.
- Stevenson, D. E.; Wibisono, R.; Jensen, D. J.; Stanley, R. A.; Cooney, J. M. Direct Acylation of Flavonoid Glycosides with Phenolic Acids Catalysed by Candida Antarctica Lipase B (Novozym 435®). Enzyme and Microbial Technology 2006, 39, 1236–1241.
- Liu, L.; Jin, C.; Zhang, Y. Lipophilic Phenolic Compounds (Lipo-Pcs): Emerging Antioxidants Applied in Lipid Systems. The Royal Society of Chemistry 2014, 4, 2879–2891.
- Aladedunye, F.; Niehaus, K.; Bednarz, H.; Thiyam-Hollander, U.; Fehling, E.; Matthäus, B. Enzymatic Lipophilization of Phenolic Extract from Rowanberry (Sorbus aucuparia) and Evaluation of Antioxidative Activity in Edible Oil. LWT - Food Science and Technology 2015, 60, 56–62.
- Zhong, Y.; Shahid, I. F. Lipophilized Epigallocatechin Gallate (EGCG) Derivatives as Novel Antioxidants. Journal of Agricultural and Food Chemistry 2011, 59, 6526–6533.
- Zhong, Y.; Ma, C.-M.; Shahidi, F. Antioxidant and Antiviral Activities of Lipophilic Epigallocatechin Gallate (EGCG) Derivatives. Journal of Functional Foods 2012, 4, 87–93.
- Vaisali, C.; Charanyaa, S.; Belur, P. D.; Regupathi, I. Refining of Edible Oils: A Critical Appraisal of Current and Potential Technologies. International Journal of Food Science and Technology 2015, 50, 13–23.
- García, A.; Ruiz-Méndez, M. V.; Romero, C.; Brenes, M. Effect of Refining on the Phenolic Composition of Crude Olive Oils. Journal of the American Oil Chemists’ Society 2006, 83, 159–164.
- Yoon, S. H.; Kim, M.; Gil, B. Deacidification Effects of Rice Hull-Based Adsorbents as Affected by Thermal and Acid Treatment. LWT - Food Science and Technology 2011, 44, 1572–1576.
- Lee, S. Y.; Jung, M. Y.; Yoon, S. H. Optimization of the Refining Process of Camellia Seed Oil for Edible Purposes. Food Science and Biotechnology 2014, 23, 65–73.
- Nergiz, C.; Celikkale, D. The Effect of Consecutive Steps of Refining on Squalene Content of Vegetable Oils. Journal of Food Science and Technology 2011, 48, 382–385.
- Lohachoompol, V.; Mulholland, M.; Srzednicki, G.; Craske, J. Determination of Anthocyanins in Various Cultivars of Highbush and Rabbiteye Blueberries. Food Chemistry 2008, 111, 249–254.
- Persson, X.-M. T.; Błachnio-Zabielska, A. U.; Jensen, M. D. Rapid Measurement of Plasma Free Fatty Acid Concentration and Isotopic Enrichment Using LC/MS. Journal of Lipid Research 2010, 51, 2761–2765.
- Abdulkarim, S. M.; Long, K. O.; Lai, M.; Muhammad, S. K. S.; Ghazali, H. M. Frying Quality and Stability of High-Oleic Moringa oleifera Seed Oil in Comparison with Other Vegetable Oils. Food Chemistry 2007, 105, 1382–1389.
- Mariod, A.; Matthäus, B.; Eichner, K.; Hussein, I. H. Frying Quality and Oxidative Stability of Two Unconventional Oils. Journal of the American Oil Chemists’ Society 2006, 83, 529–538.