ABSTRACT
Effects of tara gum (TG) at different TG concentrations (0.2–0.6% w/w) on the rheological and pasting properties of rice starch (RS) pastes (5% w/w) were studied. Confocal laser scanning microscopy (CLSM) observation was also conducted to investigate the possible interaction between RS and TG. All RS–TG mixture pastes exhibited a pseudoplastic shear-thinning characteristic (n = 0.17–0.26). RS alone displayed a highly thixotropic behaviour as compared to RS–TG mixtures, showing that the thixotropic loop area markedly decreased in the presence of TG. Consistency index (K), apparent viscosity (ηa,100), storage moduli (G′), and loss moduli (G″) values increased with increasing TG concentration. Viscous characteristics in the RS–TG mixture system were more pronounced compared to the control (0% TG) as evidenced by their higher tan δ values. Rapid visco-analysis showed that the addition of TG resulted in a significant increase in the peak, breakdown, final, and setback viscosities, and their viscosities also increased with an increase in TG concentration. These results suggested that the synergistic effect of TG addition on rheological and pasting properties of RS pastes appeared to be due to phase separation process that occurred as a result of incompatibility between TG and amylose component in starch, as confirmed by CLSM micrographs.
Introduction
Starch is one of the most important and widely available food polysaccharides. It greatly contributes to the rheological properties of many starch-based products as a functional and flexible food stabilizer for both thickening and gelling.[Citation1] The rheological characteristics of starch are known to be greatly influenced by starch type, amylose/amylopectin ratio, starch concentration, and the addition of salts, sugars, and non-starch polysaccharides (gums).[Citation2] Gums, consisting of high-molecular-weight hydrophilic polysaccharides, are also used as a functional ingredient in the food industry to control the rheological and textural properties of foods. Therefore, the addition of gum to starch plays an important role in improving the rheological and textural properties of starch-based products. Since gum influences the rheological properties of starch, it is crucial to understand the molecular interactions between them. Many studies have been conducted to determine the rheological properties of various starches mixed with galactomannans [guar gum (GG) and locust bean gum (LBG)] except for tara gum (TG). GG and LBG have been extensively studied because they are widely used in the food industry as a favourable thickening agent in food systems.
TG is galactomannans extracted by grinding the endosperm portions of the seed of the legume plant Cassalpinia spinosa.[Citation3] TG is a neutral polysaccharide composed of linear main chains of (1–4)- β -D-mannopyranose units attached by (1–6) linkage with α -D-galactopyranose units. The ratio of mannose to galactose in TG is 3:1 while it is 2:1 and 4:1 in GG and LBG, respectively, and TG possesses a more compact and flexible conformation compared to other galactomannans (GG and LBG).[Citation4] The characteristics of GG and LBG have been extensively studied, whereas less specific research was reported for TG because it was often regarded as a mixture of the two gums in galactomannan polysaccharides.[Citation5] It is also known that the galactomannans are commonly used in the food industry as food thickeners due to their high water binding capacity and synergistic interactions with starches and non-starch polysaccharides (gums), thus increasing viscosity.
Although extensive literature is available on the rheological and pasting properties of starches mixed with galactomannans, especially GG and LBG, there is still limited information on starch–TG mixtures. Therefore, in this study, TG was used to improve rheological and pasting properties of rice starch (RS) which is used as an ingredient in staple foods in Asia. In particular, no attempt has been made to study the microstructure of RS–TG mixtures using confocal laser scanning microscopy (CLSM). Such studies will improve the understanding of the physical properties of RS paste in the presence of TG. Thus, the main objective of this research was to investigate the effect of TG at different concentrations on the rheological, pasting, and structural properties of RS paste.
Materials and methods
Materials and preparation of RS–TG mixture dispersions
Commercial RS and TG were obtained from Thai Flour Industry Co. Ltd. (Bangkok, Thailand) and Silvateam Peru S.A.C. (Callao, Peru), respectively. For rheological measurements, RS–TG mixtures (total concentration 5% w/w) were prepared by mixing RS with distilled water and TG to obtain 0%, 0.2%, 0.4%, and 0.6% (weight basis) gum concentrations. The dispersions were moderately stirred for 60 min at room temperature and heated at 95°C for 30 min with mild agitation using a magnetic stirrer. After the heating period, the hot RS–TG mixture was immediately transferred to the rheometer plate.
Determination of rheological properties
The steady and dynamic shear rheological properties of the RS–TG mixtures were measured with a Haake RheoStress 1 rheometer (Haake GmbH, Karlsruhe, Germany) using a plate system (3.5 cm diameter) with a gap of 500 μm. Each sample was transferred to the rheometer plate at 25°C. Steady shear rheological tests were performed at shear rates from 0.4 to 500 s−1. To analyse time-dependent flow behaviour, the starch pastes were sheared first in ascending order (0.4–500 s−1) and then in descending order. In order to describe the steady shear rheological properties of the samples, the data of flow curves (shear stress versus shear rate) were fitted to the well-known power law model (Eq. 1):
where σ is the shear stress (Pa) ●, γ● is the shear rate (s−1), K is the consistency index (Pa●sn), and n is the flow behaviour index (dimensionless). The apparent viscosity (ηa,100) at 100 s−1 was calculated from the magnitudes of K and n. The steady shear rheological measurements were also conducted at temperatures ranging from 25 to 70°C to investigate the effect of temperature on ηa,100.
Dynamic shear properties were evaluated using small amplitude oscillatory rheological measurements. A frequency sweep test was performed in the range of 0.63–62.8 rad●s−1 at 2% strain to determine storage modulus (G′), loss modulus (G″), complex viscosity (η*), and loss tangent (tan δ =G″/G′) values. The 2% strain was in the linear viscoelastic region. All rheological experiments were performed in triplicate.
Pasting properties
Analysis of pasting properties was performed using a Rapid visco-analyzer (RVA-4, Newport Sci. Pty. Ltd, Warriewood, Australia). All RS–TG mixture dispersions (5% w/w) containing 0%, 0.2%, 0.4%, and 0.6% (weight basis) gum concentrations were prepared, as previously described. The dispersions (28 g) were then poured into aluminium canisters and stirred manually using a plastic paddle for 20–30 s prior to insertion into the RVA machine. The heating and cooling cycles were as follows: holding at 50°C for 1 min, heating to 95°C at a rate of 7.5°C/min, holding at 95°C for 5 min, cooling to 50°C at a rate of 7.5°C/min, and finally holding at 50°C for 5 min, while maintaining a rotation speed of 160 rpm. Then, the peak viscosity (maximum viscosity during pasting), breakdown viscosity (the difference between the peak viscosity and the minimum viscosity during pasting), setback viscosity (the difference between the maximum viscosity during cooling and the minimum viscosity during pasting), final viscosity (the viscosity at the end of the RVA run), and pasting temperature (temperature indicating an initial increase in viscosity) of the RS–TG mixture dispersions were determined.
Confocal laser scanning microscopy
The microstructure of samples was examined by CLSM (Nikon Eclipse Ti, Tokyo, Japan) to visualize the distribution pattern of RS granules and TG using the staining method proposed by Funami et al.[Citation6] with a slight modification. The hot pastes of RS alone and RS–TG mixture at 0.6% concentration were prepared as described in section ‘Materials and preparation of RS–TG mixture dispersions’. Rhodamine B (20 μl) and fluorescein isothiocyanate (FITC) (20 μl) were used as fluorescent dyes to identify TG and RS, respectively. In the multiple staining procedure of RS–TG mixture with two dyes, rhodamine B was immediately added after the heating of the mixture is completed. The hot mixture was allowed to cool to room temperature with continuous stirring for 2 h. Then, FITC was added, and the dyed sample was stirred for 2 h. After stirring, it was kept in the dark at room temperature for 18 h. An aliquot of the sample was placed on a slide glass and observed within 15 min. The emission wavelength was 500–525 nm for FITC and 600–635 nm for rhodamine B. Microstructure observation by CLSM of the multi-stained RS–TG mixture can lead to a better understanding of the microscopic rheological behaviour of polymer mixture systems.
Statistical analysis
The results of each sample are presented as the mean and standard deviation of triplicate analyses. Analysis of variance and Duncan’s test were used to establish the significance of differences among the mean values at the 0.05 significance level. Statistical analyses were performed using the Statistical Analysis System program (version 9.2) (SAS Institute, Cary, NC, USA).
Results and discussion
Flow properties
The shear stress (σ) versus shear rate (γ●)data of the RS–TG mixtures with different TG concentrations (0.2–0.6%) were well fitted to the simple power law model (Eq. 1) with high determination coefficients (R2 = 0.96–0.99), as shown in . All samples demonstrated shear-thinning behaviour with flow behaviour index (n) values of as low as 0.17–0.26 (). The n values of the RS–TG mixtures were higher n values (0.20–0.26) than that of the control without TG addition (0.17). This shear-thinning behaviour may result from modifications in macromolecular organization as the shear rate changes.[Citation3] At low shear rates, the disruption of entanglements is balanced by the formation of new ones. At high shear rates, disruption predominates over the formation of new entanglements, resulting in less intermolecular resistance to flow and a lower apparent viscosity. The n values of the mixtures also increased as TG concentration increased, indicating that they had less shear-thinning behaviour at higher TG concentrations. This result is in good agreement with those found in RS–LBG mixtures which had less shear-thinning behaviour than RS-GG mixtures.[Citation7] The extent of shear-thinning of starch-galactomannan mixtures can be explained in terms of gum structure.[Citation8] GG has a structure (mannose:galactose ratio = 2:1) which prevent the formation of intramolecular hydrogen bonding, resulting in an extended formation. In contrast, TG and LBG, where their ratios of mannose to galactose are 3:1 and 4:1, tend to coil with the formation of intramolecular hydrogen bondings and hence interact less with the linear amylose molecules due to the decrease in the number of hydroxyl groups available to form intermolecular hydrogen bondings with amylose molecules. Therefore, a much lower degree of shear-thinning behaviour can be observed for the RS–TG mixtures when compared to the RS–GG mixtures. Reduced shear-thinning in the presence of TG may be attributed to the increased rate of chain rearrangement in RS caused by high TG concentration, which reflects the increase in the viscosity of the RS–TG mixtures, as described by Yoo and Yoo.[Citation2] In general, the consistency index (K) and apparent viscosity (ηa,100) values of RS–TG mixtures were much higher than those of the control, and their increase with increasing TG concentration indicates a higher synergism with TG. This outcome was in agreement with that observed in previous studies of RS–galactomannan mixtures.[Citation7] Based on these results, it can be confirmed that RS–TG mixture pastes exhibit a shear-thinning behaviour, and their flow properties are greatly dependent on TG concentration.
Table 1.. Effect of tara gum concentration on steady shear properties of rice starch–tara gum mixtures.
Figure 1. Shear stress–shear rate plots of rice starch–tara gum mixtures with different tara gum concentrations at 25°C: (◯) 0%, (□) 0.2%, (△) 0.4%, (◇) 0.6%.
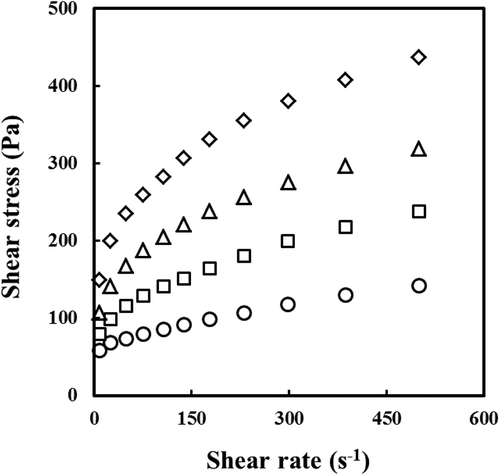
It is generally known that the effect of temperature on rheological properties needs to be documented because a wide range of temperatures are encountered during the processing and storage of fluid foods.[Citation9] The effect of temperature (25–70°C) on apparent viscosity (ηa,100) at a specific shear rate of the RS–TG mixture can be described by the Arrhenius relationship (Eq. 2), in which ηa,100 decreases to an exponential function with temperature. The Arrhenius temperature relationship has been confirmed experimentally in previous studies of starch pastes mixed with various gums.[Citation10–Citation12]
where ηa,100 is the apparent viscosity (Pa●s) at 100 s−1, A is a constant (Pa●s), T is the absolute temperature (K), R is the gas constant (8.3144 J●mol−1●K−1), and Ea is the activation energy (kJ mol−1). From a plot of ln ηa,100 (ordinate) ηersus (1/T) (abscissa), Ea equals (slope × R), and A is the exponential of the intercept. The magnitudes of Ea and A were determined from regression analysis of 1/T versus in ηa,100 (). The calculated values of Ea and the constant A were in the range of 3.07–5.11 kJ/mole and 0.21–0.37 Pa●s, respectively, with high determination coefficients (R2 = 0.95–0.99) (). The Ea values (4.79–5.11 kJ/mol) of the RS–TG mixtures were much higher than that (3.07 kJ/mol) of the control, and they increased with the increase in TG concentration. These results indicated that the decrease in ηa,100 with temperature was more pronounced in the RS–TG mixtures than the control, and the mixtures were also more sensitive to temperature change at higher TG concentrations. From these observations, it can be concluded that the Ea values of RS–TG mixtures with different TG concentrations in a temperature range of 25–70°C were significantly affected by the addition of TG and TG concentration.
Table 2. Activation energies (Ea) of rice starch–tara gum mixtures at different tara gum concentrations.
Time-dependent flow behaviour
In the range of shear rates used in this study, all samples exhibited time-dependent shear-thinning (thixotropic) flow behaviour. Hysteresis loops were found in the shear rate versus shear stress curves obtained by first increasing and then decreasing shear rates in the range of 0.4–500 s−1 (). If the sample is shear sensitive, the two flow curves do not coincide, thus causing a hysteresis loop. The hysteresis loop can be interpreted as structure breakdown by the shear field (thixotropic behaviour) in altering a structure or forming a new structure, which then maintained shear-thinning characteristics on following shear sweeps.[Citation10] It is known that the area enclosed by the hysteresis loop is an indication of the degree of breakdown due to shearing.[Citation13] Thixotropy was less pronounced in the presence of TG as seen by the area of the loop between the up and down flow curves (), indicating that TG addition apparently enhanced re-association after structure breakdown caused by high shear. The thixotropic loop area of the RS–TG mixture decreased with increasing TG concentration. In particular, the loop area of RS paste with 0.4% TG was markedly decreased compared with that of RS paste alone, and it seemed to disappear with 0.6% TG. The smaller loops at higher TG concentrations can be attributed to rather small changes in the structure of RS–TG mixture pastes when shear was applied, thus indicating a low degree of structural breakdown during shearing. This result indicated that TG addition at high concentrations enhanced the formation of the shear-induced network structure of the RS–TG mixtures and reduced the breakdown of the network by shear. Similar observations have also been reported for other starch–gum mixture pastes.[Citation11,Citation12]
Dynamic rheological properties
shows the changes in storage modulus (G′) and loss modulus (G″) as a function of frequency (ω) of RS–TG mixture pastes at 25°C. In general, G′ and G″ values increased with increasing ω and G′ was much higher than G″ with a ω dependency. The slope values of the dynamic moduli (G′ and G″) were positive, demonstrating viscoelastic and weak gel-like behaviour. A similar trend has also been observed in other starches mixed with galactomannans (e.g. GG and LBG)[Citation1,Citation14,Citation15] The dynamic moduli (G′, G″) and complex viscosity (η*) of the RS–TG mixture pastes were significantly higher than those of the control, and these values increased with increasing TG concentration from 0.2% to 0.6%, as shown in . These results indicated that the addition of TG had a synergistic effect on the viscoelastic properties of RS, leading to a significant increase in the dynamic moduli of RS–TG mixtures. The increased viscoelastic properties in the presence of TG can be attributed to the increase of viscoelastic properties of added TG. TG can be concentrated within the continuous phase in the starch–gum mixture system because of segregative interactions caused by the thermodynamic incompatibility of gum with the amylose component in starch, as described by the studies of Yoo et al.[Citation7] and Kim et al.[Citation16] on the viscoelastic properties of RS–GG and RS–LBG mixtures.
Table 3. Log G′, G″, η*and tan δ value at 6.28 rad·s−1 of rice starch–tara gum mixtures with different tara gum concentrations.
Figure 4. Plots of log G′, log G″ versus log ω of rice starch–tara gum mixtures with different tara gum concentrations at 25°C: (◯) 0%, (□) 0.2%, (△) 0.4%, (◇) 0.6%.
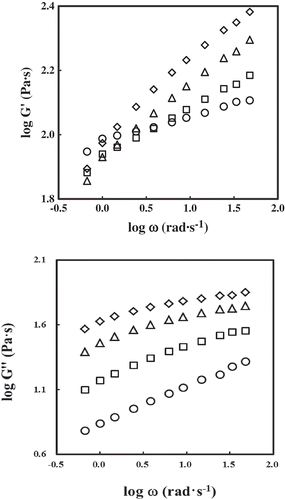
In general, the loss tangent ‘tan δ’ (ratio of G″/G′) can be used to identify differences in viscoelastic behaviour. The ‘tan δ’ values of all samples were in the range of 0.11–0.37 and increased with increasing TG concentration (). In particular, there were significant differences in the tan δ values between the control (0.11) and the RS–TG mixtures (0.21–0.37). The increase in tan δ indicated an increase in viscous characteristics in the system because of greater changes in G″ than in G′. Furthermore, similar results have been reported in other studies.[Citation7,Citation13,Citation16–Citation18] These observations suggest that the viscous properties of RS–TG mixture pastes could be greatly affected by the addition of TG, depending on its concentration. In general, the dependence of viscous properties on gum concentration is associated with the thermodynamic incompatibility between the amylose component and gums.[Citation7,Citation16] The viscoelastic rheological behaviour in RS–TG mixture can be determined by the characteristics of RS (permanent junction zones in the network) and the characteristics of the TG (temporary entanglements in the network).[Citation16,Citation17] According to Eidam et al.,[Citation17] in the three-dimensional network the polysaccharide molecules function as disruptive sites, resulting in the decreased overall elasticity due to the reduced number of permanent junction zones in the starch. This indicates that the decrease in junction zones of RS molecules with increasing TG concentration results in an increase of the viscous properties. In addition, the thermodynamic incompatibility phenomenon can be explained by the conformational characteristics of TG; its chains are more compact and flexible compared with those of GG and LBG, as reported by Wu et al.[Citation4] Therefore, the synergistic effect of TG on the rheological properties of RS paste can be attributed to the formation of a thermodynamically incompatible network structure between RS and TG because of the much higher tan δ values of RS–TG mixtures compared with that of the control with increasing TG concentration. The same mechanism was also reported with RS or corn starch containing GG and LBG.[Citation7,Citation17,Citation18] These viscoelastic results showed that the desirable properties of RS for industrial use can be enhanced or modified by the addition of TG.
Pasting properties
All pasting parameters of RS in the presence of TG at different concentrations determined by RVA are summarized in . Considering the effect of TG concentration on RS–TG mixtures, the addition of TG resulted in a significant increase in peak, breakdown, final, and setback viscosities, in a concentration-dependent manner (p < 0.05). The significant increase in peak viscosity values with increasing TG concentration can be explained by the biphasic system with the gum located in the continuous phase, in which its concentration is greatly increased as the volume of the phase accessible to the gum is reduced due to swelling of the starch granules during gelatinization.[Citation19] The breakdown of the RS–TG mixtures was greater than that of the control and significantly increased with an increase in TG concentration, which could be caused by increases in viscosity, leading to much greater shear forces on the swollen granules in the shear field compared with those encountered in starch dispersions.[Citation20] The setback values of all mixtures were also higher compared with that of the control and increased with an increase in TG concentration. In comparison with the control, the RS–TG mixtures were characterized by much lower pasting temperatures. According to Galkowska et al.[Citation21] and Funami et al.,[Citation22] the lower pasting temperatures in starch–gum mixture systems can be associated with an increase in the effective starch concentration in the continuous phase, thus enhancing the interaction between the granules.
Table 4. Pasting properties of rice starch–tara gum mixtures at different tara gum concentrations.
Confocal laser scanning microscopy
The network structure of RS paste in the presence or absence of TG was observed by CLSM (). In the CLSM micrographs, RS was labelled with FITC, and TG was labelled with rhodamine B, which corresponded to the green and red areas in the mixture, respectively. The CLSM micrographs clearly demonstrated a phase separation between RS and TG where TG molecules separate into local domains. This separation can be explained by the thermodynamic incompatibility between different polysaccharides, resulting from the preference for interactions between similar polysaccharides over interactions between different polysaccharides.[Citation18] Therefore, CLSM confirmed the formation of a thermodynamically incompatible network structure between RS and TG. This result was in good agreement with our results on the dynamic rheological and pasting properties of RS–TG mixtures.
Conclusion
The rheological parameters (ηa,100, K, and n) of RS–TG mixtures with different TG concentrations (0.2–0.6%) were strongly influenced by TG concentration. The ηa,100 values of RS–TG mixtures were more sensitive to temperature changes in comparison with that of RS alone, as demonstrated by the Arrhenius temperature relationship. The magnitudes of dynamic moduli (G′, G″) increased with increasing TG concentration, indicating a synergistic effect of TG addition on the viscoelastic properties of RS pastes. The presence of TG significantly influenced the pasting characteristics of the RS–TG mixtures significantly by increasing the RVA paste viscosity values (peak, breakdown, final, and setback viscosities) and decreasing the pasting temperature. RS–TG mixture pastes demonstrated distinct microstructures and rheological properties related to the thermodynamic incompatibility phenomenon. Our results on the rheological and pasting properties of the RS–TG mixture systems also indicated that CLSM can provide useful information to better understand the effects of gums on the behaviour of starch pastes in starch–gum mixture systems. In addition, the results of this study can contribute to knowledge of the rheological, pasting, and structural properties of the RS–TG mixture system for improving the formulations of RS-based food products in the food industry.
Additional information
Funding
References
- Choi, H. M.; Yoo, B. Rheology of Mixed Systems of Sweet Potato Starch and Galactomannans. Starch-Starke. 2008, 60, 263–269. DOI: 10.1002/star.200700703.
- Yoo, D.; Yoo, B. Rheology of Rice Starch-Sucrose Composites. Starch-Starke. 2005, 57, 254–261. DOI: 10.1002/star.200400356.
- Sittikijyothin, W.; Torres, D.; Goncalves, M. P. Modelling the Rheological Behaviour of Galactomannan Aqueous Solutions. Carbohydr. Polym. 2005, 59, 339–350. DOI: 10.1016/j.carbpol.2004.10.005.
- Wu, Y.; Li, W.; Cui, W.; Eskin, N. A. M.; Goff, H. D. A Molecular Modeling Approach to Understand Conformation-Functionality Relationships of Galactomannans with Different Mannose/Galactose Ratios. Food Hydrocolloids. 2012, 26, 359–364. DOI: 10.1016/j.foodhyd.2011.02.029.
- Wu, Y. B.; Ding, W.; Jia, L. R.; He, Q. The Rheological Properties of Tara Gum (Caesalpinia Spinosa). Food Chem. 2015, 168, 366–371. DOI: 10.1016/j.foodchem.2014.07.083.
- Funami, T.; Nakauma, M.; Noda, S.; Ishihara, S.; Al-Assaf, S.; Nishinari, K.; Phillips, G. O. Effects of Some Anionic Polysaccharides on the Gelatinization and Retrogradation Behaviors of Wheat Starch: Soybean-Soluble Polysaccharide and Gum Arabic. Food Hydrocolloids. 2008, 22, 1528–1540. DOI: 10.1016/j.foodhyd.2007.10.008.
- Yoo, D.; Kim, C.; Yoo, B. Steady and Dynamic Shear Rheology of Rice Starch-Galactomannan Mixtures. Starch-Starke. 2005, 57, 310–318. DOI: 10.1002/star.200400390.
- Sudhakar, V.; Singhal, R. S.; Kulkarni, P. R. Starch-Galactomannan Interactions: Functionality and Rheological Aspects. Food Chem. 1996, 55, 259–264. DOI: 10.1016/0308-8146(95)00127-1.
- Rao, M. A.;. Flow and Functional Models for Rheological Properties of Fluid Foods. In Rheology of Fluid and Semisolid Foods; Rao, M. A., Ed.; Maryland: Aspen Pub., 1999, pp. 25–57.
- Achayuthakan, P.; Suphantharika, M. Pasting and Rheological Properties of Waxy Corn Starch as Affected by Guar Gum and Xanthan Gum. Carbohydr. Polym. 2008, 71, 9–17. DOI: 10.1016/j.carbpol.2007.05.006.
- Sikora, M.; Kowalski, S.; Tomasik, P. Binary Hydrocolloids from Starches and Xanthan Gum. Food Hydrocolloids. 2008, 22, 943–952. DOI: 10.1016/j.foodhyd.2007.05.007.
- Korus, J.; Juszczak, L.; Witczak, M.; Achremowicz, B. Influence of Selected Hydrocolloids on Triticale Starch Rheological Properties. Int. J. Food Sci. Technol. 2004, 39, 641–652. DOI: 10.1111/ifs.2004.39.issue-6.
- Weltman, R. N.;. Breakdown of Thixotropic Structure as a Function of Time. J. Appl. Phys. 1943, 14, 343–350. DOI: 10.1063/1.1714996.
- Nagano, T.; Tamaki, E.; Funami, T. Influence of Guar Gum on Granule Morphologies and Rheological Properties of Maize Starch. Carbohydr. Polym. 2008, 72, 95–101. DOI: 10.1016/j.carbpol.2007.07.028.
- Kim, W. W.; Yoo, B. Rheological and Thermal Effects of Galactomannan Addition to Acorn Starch Paste. LWT - Food Sci. Technol. 2011, 44, 759–764. DOI: 10.1016/j.lwt.2010.11.009.
- Kim, C.; Lee, S. P.; Yoo, B. Dynamic Rheology of Rice Starch-Galactomannan Mixtures in the Aging Process. Starch-Starke. 2006, 58, 35–43. DOI: 10.1002/star.200500408.
- Eidam, D.; Kulicke, W. M.; Kuhn, K.; Stute, R. Formation of Maize Starch Gels Selectively Regulated by the Addition of Hydrocolloids. Starch-Starke. 1995, 47, 378–384. DOI: 10.1002/star.19950471003.
- Alloncle, M.; Doublier, J. L. Viscoelastic Properties of Maize Starch/Hydrocolloid Pastes and Gels. Food Hydrocolloids. 1991, 5, 455–467. DOI: 10.1016/S0268-005X(09)80104-5.
- Chaisawang, M.; Suphantharika, M. Pasting and Rheological Properties of Native and Anionic Tapioca Starches as Modified by Guar Gum and Xanthan Gum. Food Hydrocolloids. 2006, 20, 641–649. DOI: 10.1016/j.foodhyd.2005.06.003.
- Li, X. J.; Xing, Y.; Sun, Q. J.; Chu, L. J.; Xiong, L. Effect of Food Gums on Properties of Pea Starch and Vermicelli Prepared from Pea Starch. Starch-Starke. 2015, 67, 399–406. DOI: 10.1002/star.201400100.
- Galkowska, D.; Pycia, K.; Juszczak, L.; Pajak, P. Influence of Cassia Gum on Rheological and Textural Properties of Native Potato and Corn Starch. Starch-Starke. 2014, 66, 1060–1070. DOI: 10.1002/star.201400078.
- Funami, T.; Kataoka, Y.; Omoto, T.; Goto, Y.; Asai, I.; Nishinari, K. Effects of Non-Ionic Polysaccharides on the Gelatinization and Retrogradation Behavior of Wheat Starch. Food Hydrocolloids. 2005, 19, 1–13. DOI: 10.1016/j.foodhyd.2004.04.024.