ABSTRACT
In this study, a cationic soluble peroxidase (POD) was homogeneously purified and biochemically characterized. The enzyme purification involved the combination of (NH4)2SO4 precipitation, CM-Sephadex cation exchange, and gel filtration chromatography. The purity of the enzyme was examined by sodium dodecyl sulphate-polyacrylamide gel electrophoresis, and one single prominent band was obtained with a molecular weight of approximately 45 kDa. The thermal and pH stability showed that the enzyme was stable at pH 5.0 and at 40°C, respectively. The activity remained stable at pH 4.0 at least for 10 days. Km and Vmax values were calculated from Lineweaver–Burk graph for each substrate. POD exhibited Km values of 0.0154 and 0.065 mM for guaiacol and H2O2, respectively. The enzyme was highly inhibited by sodium azide. This study could enrich the literature regarding the properties of POD from haricot bean that could be applicable to biomedical analysis.
Introduction
Peroxidase (POD, E.C. 1.11.1.7) is a haeme-containing enzyme that catalyses the oxidation of a wide-range electron donating substrate with hydrogen peroxide (H2O2).[Citation1] It is widely distributed in plant kingdom and plays role in oxidation and polymerization of phenols and aromatic rings. POD has great importance in cellular functions such as lignification, cell elongation, growth, wound healing, and biosynthesis of cell walls.[Citation2] It has been also shown that POD expression locally increases in some plant tissues under pathological conditions.[Citation3] PODs could be anionic or cationic, which have been related to pathogens and embryogenesis, respectively.[Citation4] POD has attracted attentions because of its common use in various applications including biosensors, ELISA kits, and remedification of odour smells. Also, lactoperoxidase, which is a POD enzyme secreted from mammary, salivary, and other mucosal glands, was extensively researched[Citation5] because of its function as a natural antibacterial agent.[Citation6]
Extraction and purification of an enzyme is one of the important steps to obtain the product without losing its biochemical properties during the bioprocessing. The requirement of a successful purification is to achieve maximum yield with minimum cost. Therefore, rapid and effective purification steps are of interest to reduce the cost and increase the possibility of daily use. Although POD is ubiquitously found in plant, the commercially available POD is produced from the roots of horseradish.[Citation7] However, plant PODs are known to have a wide range of isoenzymes that could differ in terms of thermal stability, optimum pH, and substrate specificity.[Citation8] Significant improvements were archived through purification and characterization of POD from different species to find new ones, which might be stable at extreme conditions such as low pH or high temperature. POD was purified from different species including wheat[Citation9], Brassica oleracea,[Citation1] and sweet gourd.[Citation2] Although haricot bean POD was partly purified,[Citation10] the complete purification and characterization have not been performed yet. We focused the variety of Phaseolus vulgaris, since it is largely produced in the province of Erzincan, which contributes an important part of haricot production in Turkey.
The current work deals with the purification of POD from easily available haricot bean with an inexpensive extraction procedure. In this study, we aimed to purify and characterize haricot bean POD to discover new potential source of POD that could be useful in food industry and biochemical applications. We also studied kinetic and inhibition properties of haricot bean POD.
Materials and methods
Plant materials
Haricot beans (Phaseolus vulgaris L.) were thoroughly washed with distilled water. Beans were frozen in liquid nitrogen and grounded to a fine powder with pestle and mortar chilled in liquid nitrogen. The bean powder was stored −20°C until the enzyme extraction was carried out.
Ammonium sulphate precipitation and dialysis
For the first step of the purification, powdered sample (20 g) was suspended in phosphate buffer (pH 7.5, 0.1 M) and the homogenate was incubated at 20°C for 30 min. After removing the debris via filtration through Whatman filter paper (No. 1), the sample was centrifuged at 8000×g for 30 min at 4°C using refrigerated table top centrifuge.[Citation11] The pellet was discarded and supernatant was collected and referred to as “sample extract.” Finely ground weighed ammonium sulphate was added to the sample extract with constant stirring in ice. Fractionation of proteins involved 0–10% to 70–80% saturation with ammonium sulphate, and after each saturation, sample extract was centrifuged for 45 min at 12,000 × g. Supernatant was kept, and the resulting pellet was dissolved in 2 mL phosphate buffer (pH 7.0, 0.1 M). The fraction with maximum POD activity was taken and dialysed in a dialysis tube against 100 mM phosphate buffer (pH 7.0, 1 mM NaCl), for 24 h.
Preparation of CM-Sephadex A-50 ion-exchange chromatography material
For further purification, the sample was applied onto the carboxymethyl (CM)-cellulose column pre-equilibrated with Tris–HCl (0.1 M, pH 9.0). The column was washed with 250 mL of the phosphate buffer, and the retained material was eluted using a continuous linear gradient of NaCl (1.0 M) with the same buffer at a flow rate of 15 mL.h−1. The fractions (3 mL.tube−1) were collected and immediately analysed for POD activity. The protein content of the fractions was determined by recording the absorbance at 280 nm. Following the purification procedure described above, aliquots showing the highest POD activity were combined and electrophoresis was performed. Our primarily result showed that POD activity is lost, if the sample is stored at 4°C until use (data not shown). Therefore, after ion-exchange chromatography, the fractions showing the highest POD activity were pooled and the sample was immediately applied ion gel filtration chromatography.
Gel filtration chromatography
Gel filtration chromatography was performed using a calibrated Sephadex G-250 column equilibrated with 6 mM phosphate buffer at 4°C.[Citation11] POD was eluted with a linear 0–100 mM NaCl gradient at a flow rate of 15 mL/h. The fractions of 3 mL were collected and followed by measuring absorbance at 280 nm for qualitative protein determination and 470 nm for POD activity determination.
Protein electrophoresis
In order to check the success of the purification and molecular weight of POD, sample with the highest POD activity obtained from gel filtration chromatography was loaded to sodium dodecyl sulphate (SDS) gel electrophoresis using 12.5% and 4% stacking polyacrylamide slab gels according to Laemmli[Citation12] as described previously in detail.[Citation13] The gel was run at constant current of 80 V per gel for 3 h followed by 110 V until the tracking dye reached the bottom of the plate. Protein bands were visualized using Coomassie Blue staining.[Citation14]
Peroxidase activity
Measurement of POD activity is based on absorbance reading of tetra guaiacol formation in the presence of guaiacol and hydrogen peroxide within 2 min. Briefly, 1.0 mL of 45 mM guaiacol and 1.0 mL of 22.5 mM hydrogen peroxide in 0.1 M phosphate buffer pH 7 at 4 ºC was mixed and the reaction was started by adding 50 μL of POD solution. Increases in the absorbance were recorded spectrophotometrically at 470 nm. One unit of enzyme activity was defined as the amount of enzyme that catalyses the production of 1.0 mmol of tetraguaiacol per minute.[Citation15]
Km and Vmax determination
For determination of Michaelis constant (Km) and maximum velocity (Vmax) of POD, enzymatic activity was measured using different concentrations of guaiacol and hydrogen peroxide.[Citation16] Km and Vmax values of POD were calculated by plotting 1/Vo versus 1/S by the method of Lineweaver and Burk as shown below:
where [S] is the concentration of the substrate and Vo is the initial reaction rate (EU.mL−1.min−1), Vmax is the maximum rate, and Km is the Michaelis–Menten constant (mM).[Citation17]
Stability and optimal temperature and pH determination
In order to achieve optimum pH conditions, the following buffer systems were used: pH of 3.5–4.5 with acetate; pH 5.0–7.0 phosphates; and pH 7.5–9.0 with Tris buffer. POD activity as a function of temperature was also assessed at various temperatures at 40°C and 50°C at optimum pH. Before induction of the enzyme, compounds in test tube (substrate and buffer) were heated in a water bath to reach the desired temperature. POD activity under the conditions mentioned above was measured in experimental level of day 0, 2, 4, 6, 8, 10, and 12 using guaiacol as substrate.
Vitro inhibition assay
The in vitro inhibition of haricot bean POD by different inhibitors was also determined. The purified POD was mixed with various inhibitors at different concentrations () for 3 min at room temperature. The remaining specific activities were measured using guaiacol as substrate.
Table 1. Purification of peroxidase from haricot bean (Phaseolus vulgaris L.).
Table 2. The inhibition effects of different chemicals on peroxidase activity from haricot bean (Phaseolus vulgaris L.).
Protein assay
Total protein content was determined according to Braford method[Citation18] using bovine serum albumin (BSA) as standard.[Citation19] In this assay, the colour of the complex formed by the reaction of Coomassie Brilliant Blue G-250 agent with proteins in the presence of phosphoric acid is measured at 595 nm. Samples were prepared in triplicate and BSA was used as standard.[Citation20] For qualitative measurement of the proteins, absorptions of eluates from chromatography were recorded at 280 nm.[Citation21]
Result and discussion
Enzyme purification
In this study, POD from haricot bean was successfully purified through a procedure consisting of ammonium sulphate precipitation, gel filtration, and CM-Sephadex cation-exchange chromatographies. The specific activity, purification fold, and the yield (%) for each step are presented in .
Ammonium persulfate precipitation
In the first step of purification, crude extract of POD was precipitated with ammonium sulphate and different factions were obtained from 20% to 70%. After the addition of ammonium sulphate to the extract at each interval, sample was centrifuged and the pellet was solubilized in Tris–HCl buffer. POD activities of both precipitate and supernatant from all fractions were measured. Significantly higher POD activity was detected in the supernatant of first fraction and decreased by the following fractions (). Precipitation in the range of 50–60% saturation resulted in a substantial increase in POD activity in the pellet and removal of great amount of other proteins. Therefore, intervals of 50–60% ammonium sulphate saturation were chosen for further purification steps.
Figure 1. Purification of peroxidase (POD) purified from haricot bean (Phaseolus vulgaris L.). (A) Ammonium sulphate precipitation, (B) CM-Sephadex exchange chromatography, and (C) gel filtration chromatography.

Before loading the sample to ion-exchange chromatography, the sample was dialysed to remove the salt. In the literature, ammonium sulphate precipitation is a still widely used technique to partly purify the protein of interest. For example, ammonium sulphate saturation was used for purification of POD from horseradish cv Balady[Citation22], cabbage leaves,[Citation23] or Turkish black radish.[Citation24] There are also other studies where, instead of ammonium sulphate, acetone is used, which was claimed to remove colour material that tended to clog in the ion-exchange column chromatography.[Citation25]
In order to increase the efficacy of the purification steps and to remove large amounts of phenols that are present in the sample, previously partial purification of POD from Metroxylon sagu was also performed via the combination of temperature-induced phase partitioning ion-exchange and gel filtration chromatography. In this method, the extract POD is obtained via the addition of insoluble poly(vinylpyrrolidone) and Triton X-100 in 50 mM phosphate buffer (pH 7.5). However, it does not appear to be always effective, since increasing Triton X-100 concentration did not change the activity of POD activity from sweet potato tubers.[Citation26]
Cation-exchange chromatography
The second step of the purification was cation-exchange chromatography as shown in . For each fraction, POD activity and the amount of protein were spectrophotometrically measured at 470 nm and 280 nm, respectively. After the analysis, the chromatography resulted in only one peak with POD activity, consisting the fractions of 19, 20, and 21 which were pooled together and loaded to gel filtration chromatography. The result of the purification of POD from haricot bean is shown in and . This elution profile showed that the enzyme possess a net cationic charge that allowed it to be retained in negatively charged surface. Ion-exchange chromatography is especially useful to study different isoforms of POD with charged surface. For example, using ion-exchange chromatography, it has been shown that wheat bran,[Citation3] and Copaifera langsdorffii [Citation8] indicated only one peak for PODs, whereas broccoli stems[Citation27] contained three POD isoenzymes with three peaks in elution profile.
Gel filtration chromatography
The third step of purification involved gel chromatography. The elution profile is shown in . Only one peak was obtained through this step, which suggests that the enzyme is successfully obtained. The sample only exhibited 2.26 U/mg specific activity, which shows that haricot bean POD is not quite stable during the procedure of the purification. However, using the same techniques presented in this study, garlic POD and ber fruit PODs were obtained with 25.256 U/mg[Citation28] and 804.9 U/mg specific activities.
Molecular weight and purity
The purity and Mw of POD were determined by SDS-polyacrylamide gel electrophoresis (PAGE).[Citation29,Citation30] POD showed a distinct band between 50 and 35 kDa standard proteins markers and Mw of the purified POD was estimated as 45 kDa relative to the standard molecular weight (). Molecular weight of most plant PODs were reported between 30 and 60 kDa, which could be due to differences in amino acid sequence or the degree of glycosylation.[Citation31] For example, molecular weight of POD was 44 kDa for cauliflower,[Citation18] 38 kDa for artichoke,[Citation32] 50 kDa for windmill palm tree, and 39 kDa for chickpea cultivar.[Citation33] POD could be monomer such as found in Chlorella vulgaris or homotetramer such as found in oil from palm leaf.[Citation34]
Characterization studies
Optimum pH and pH stability
pH is crucial since it determines the ionization state of amino acid side chain on the enzyme and substrate. The effect of pH on the activity of POD is shown in . With the guaiacol as a substrate, the optimum catalytic pH of POD was found to be 5.0. We also looked at pH stability over the course of 2 weeks and found that POD was stable up to 14 days at pH 5.0 (). However, the enzyme was fairly unstable at pH 4.0, pH 6.0, and pH 7.0. There was almost no activity after day 8 at those pH values. However, there are reports showing that the optimum pH of POD varies; for example, it is pH 5.0 for bitter gourd[Citation35] and pH 7.0 for Raphanus sativus[Citation36] and between pH 5.3–6.3 for wheat germ.[Citation37]
Figure 3. The effect of different conditions on peroxidase (POD) purified from haricot bean (Phaseolus vulgaris L.). (A) Optimum pH of POD, (B) the enzyme stability at different pH with time, (C) the effect of temperature on POD activity, (D) thermal stability of POD, and (E) the effect of ionic strength on POD.
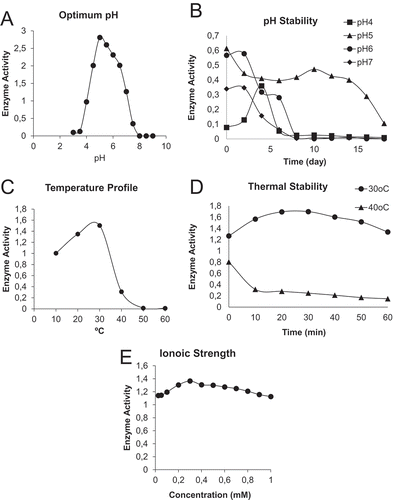
Optimum temperature profile and thermal stability
For determination of thermal and storage stability, POD activities were measured at different temperatures. As shown in , POD was stable at 10 and 20°C and showed highest activity at 30oC. After that point, the enzymatic activity was gradually lost at 40°C and no activity was detected at 50 and 60°C (). We also investigated the thermostability of crude enzyme extract, as a function of two different temperatures during 60 min (). The POD extracted showed maintained activity at 30oC even after 60 min, whereas the activity of POD at 40°C declined in the first 10 min and continued to gradually decline in 60 min (). There are some PODs, which are stable at even at 60°C such as spinach.[Citation38] Turnip POD was also found to be stable at 50°C and its activity was slightly decreased at 60°C.[Citation5] Interestingly, intracellular POD from genetically transformed roots of red beet maintained 95% of its activity at 50°C and, once it was purified; more than 70% of its activity is retained at 70°C.[Citation39]
Effect of ionic strength
The optimum ionic strength for POD activity was determined by measurement of the enzymatic activity with different NaCl concentrations. As seen in , the addition of salt did not significantly affect the activity of POD, although slightly higher activity was observed at 0.3 mM NaCl concentration. Previous studies indicated that high concentration of NaCl (2.5 M) resulted in 44% enhancement in POD activity from Jatropha curcas.[Citation40] Similarly, POD from Leucaena leucocephala leaves has been reported to have optimum ionic strength at 3.0 M NaCl concentrations.[Citation41]
Kinetic studies
Substrate specificity, Km and Vmax
In order to determine the Km and Vmax for POD, the Michaelis–Menten and double reciprocal plots were constructed using guaiacol and H2O2 as substrate at various concentrations ( and ). The calculated Km for guaiacol and H2O2 were 0.0154 mM and 0.065 mM, respectively. In the literature, Km values reported as 0.08 mM and 8.4 µM for horseradish POD[Citation42] and Turkish black radish POD,[Citation24] respectively.
Effect of various chemicals on POD enzyme activity
Studies on the inhibition of POD have been also investigated using different inhibitors, namely citric acid, EDTA, CTAB, and sodium azide on the activity of POD (). The most effective inhibitor was found to be sodium azide by 99% inhibition at 5 mM concentration. CTAB was a weak inhibitor as compared to sodium azide at 20 mM concentration.
Conclusion
Due to the ability of catalysing a wide range of biochemical reaction, POD is a valuable enzyme in industry. The discovery of POD sources with different characteristics is important to provide new sources for future applications. In this study, a novel POD was purified from haricot bean (Phaseolus vulgaris L.) and its optimum catalytic and kinetic activities were investigated. The comparison of enzyme characteristics was similar to those reported in the literature. Information provided here could be useful for purification of POD from a different source and for application that POD is utilized. The authors declare that no conflict of interest.
References
- Köksal, E.; Ağgül, A. G.; Bursal, E.; Gülçin, İ. Purification and Characterization of Peroxidase from Sweet Gourd (Cucurbita Moschata Lam. Poiret). International Journal of Food Properties 2012, 15, 1110–1119.
- Gülçin, İ.; Yıldırım, A. Purification and Characterization of Peroxidase from Brassica Oleracea Var. Acephala. Asian Journal of Chemistry 2005, 17, 2175–2183.
- Manu, B.; Rao, U. P. Calcium Modulated Activity Enhancement and Thermal Stability Study of a Cationic Peroxidase Purified from Wheat Bran. Food Chemistry 2009, 114, 66–71.
- Fernandes, C. F.; Moraes, V. C. P.; Vasconcelos, I. M.; Silveira, J. A. G.; Oliveira, J. T. A. Induction of an Anionic Peroxidase in Cowpea Leaves by Exogenous Salicylic Acid. Journal of Plant Physiology 2006, 163, 1040–1048.
- Şişecioğlu, M.; Çankaya, M.; Gülçin, İ.; Özdemir, M. Interactions of Melatonin and Serotonin to Lactoperoxidase Enzyme. Journal of Enzyme Inhibition and Medicinal Chemistry 2010, 25, 779–783.
- Şişecioğlu, M.; Gülçin, İ.; Çankaya, M.; Özdemir, H. The Inhibitory Effects of L-Adrenaline on Lactoperoxidase Enzyme (LPO) Purified from Buffalo Milk. International Journal of Food Properties 2012, 15, 1182–1189.
- Hiner, A. N.; Ruiz, J. H.; Arnao, M. B.; Garcia-Canovas, F.; Manuel, A. A Comparative Study of the Purity, Enzyme Activity, and Inactivation by Hydrogen Peroxide of Commercially Available Horseradish Peroxidase Isoenzymes A and C. Biotechnology and Bioengineering 1996, 50, 655–662.
- Maciel, H. P. F.; GouvêA, C. M. C. P.; Toyama, M.; Smolka, M.; Extraction, M. S. Purification and Biochemical Characterization of a Peroxidase from Copaifera Langsdorffii Leaves. Quimica Nova 2007, 30, 1067–1071.
- Altın, S.; Tohma, H.; GüLçIn, I. ̇.; KöKsal, E. Purification, Characterization and Inhibition Sensitivity of Peroxidase from Wheat (Triticum aestivum Ssp. Vulgare). International Journal of Food Properties 2016, 20, 1949–1959.
- Kermasha, S.; Metche, M. Studies on Seed Peroxidase Phaseolus vulgaris Cv, Haricot. Journal of Food Science 1998, 53, 247.
- Gülçin, İ.; Küfrevioğlu, Ö. İ.; Oktay, M. Purification and Characterization of Polyphenol Oxidase from Nettle (Urtica dioica L.) And Inhibition Effects of Some Chemicals on the Enzyme Activity. Journal of Enzyme Inhibition and Medicinal Chemistry 2005, 20, 297–302.
- Laemmli, U. K.;. Cleavage of Structural Proteins during the Assembly of the Head of Bacteriophage T4. Nature 1970, 227, 680–685.
- Gülçin, İ.; Beydemir, Ş.; Hisar, O. The Effect of α-tocopherol on the Antioxidant Enzymes Activities and Lipid Peroxidation of Rainbow Trout (Oncorhynchus mykiss). Acta Veterinaria Hungarica 2005, 53, 425–433.
- Gülçin, İ.; Beydemir, Ş.; Hisar, O.; Köksal, E.; Reiter, R. J. Melatonin Administration Increases Antioxidant Enzymes Activities and Reduce Lipid Peroxidation in the Rainbow Trout (Oncorhynchus Mykiss, Walbaum) Erythrocytes. Turkish Journal of Veterinary and Animal Sciences 2009, 33, 241–245.
- Koksal, E.; Gulcin, I. Purification and Characterization of Peroxidase from Cauliflower (Brassica Oleracea L. Var. Botrytis) Buds. Protein Peptide Letters 2008, 15, 320–326.
- Gülçin, İ.; Beydemir, Ş.; Çoban, T. A.; Ekinci, D. The Inhibitory Effect of Dantrolene Sodium and Propofol on 6-Phosphogluconate Dehydrogenase from Rat Erythrocyte. Fresenius Environmental Bulletin 2008, 17, 1283–1287.
- Öztürk Sarıkaya, S. B.; Gülçin, İ.; Supuran, C. T. Carbonic Anhydrase Inhibitors. Inhibition of Human Erythrocyte Isozymes I and II with a Series of Phenolic Acids. Chemical Biology and Drugs Design 2010, 75, 515–520.
- Bradford, M. M.;. Rapid and Sensitive Method for the Quantitation of Microgram Quantities of Protein Utilizing the Principle of Protein-Dye Binding. Analytical Biochemistry 1976, 72, 248–254.
- Şıktar, E.; Ekinci, D.; Şıktar, E.; Beydemir, Ş.; Gülçin, İ.; Günay, M. Protective Role of L-Carnitine Supplementation against Exhaustive Exercise-Induced Oxidative Stress in Rats. European Journal of Pharmacology 2011, 668, 407–413.
- Aydin, B.; Gulcin, I.; Alwasel, S. H. Purification and Characterization of Polyphenol Oxidase from Hemşin Apple (Malus communis L.). International Journal of Food Properties 2015, 18, 2735–2745.
- Öztürk Sarıkaya, S. B.; Topal, F.; Şentürk, M.; Gülçin, İ.; Supuran, C. T. In Vitro Inhibition of α-carbonic Anhydrase Isozymes by Some Phenolic Compounds. Bioorganic and Medicinal Chemistry Letters 2011, 21, 4259–4262.
- Mohamed, S. A.; Abulnaja, K. O.; Ads, A. S.; Khan, J. A.; Kumosani, T. A. Characterisation of an Anionic Peroxidase from Horseradish Cv. Balady. Food Chemistry 2011, 128, 725–730.
- Pandit, A. B.; Kharatmol, P. P. Extraction, Partial Purification and Characterization of Acidic Peroxidase from Cabbage Leaves (Brasicca olearacea Var. capitata). Journal of Biochemical Technology 2013, 4, 531–540.
- Şişecioğlu, M.; Gülçin, İ.; Çankaya, M.; Atasever, A.; Şehitoğlu, M. H.; Budak Kaya, H.; Özdemir, H. Purification and Characterization of Peroxidase from Turkish Black Radish (Raphanus sativus L.). Journal of Medicinal Plants Research 2010, 4, 1187–1196.
- Duarte-Vazquez, M. A.; García-Padilla, S.; García-Almendárez, B. E.; Whitaker, J. R.; Regalado, C. Broccoli Processing Wastes as a Source of Peroxidase. Journal of Agricultural and Food Chemistry 2007, 55, 10396–10404.
- Leon, J. C.; Alpeeva, I. S.; Chubar, T. A.; Galaev, I.; Csöregi, E.; Sakharov, I. Y. Purification and Substrate Specificity of Peroxidase from Sweet Potato Tubers. Plant Science 2002, 163, 1011–1019.
- Thongsook, T.; Barrett, D. M. Purification and Partial Characterization of Broccoli (Brassica oleracea Var. Italica) Peroxidases. Journal of Agricultural and Food Chemistry 2005, 53, 3206–3214.
- Osuji, A. C.; Eze, S. O. O.; Osayi, E. E.; Chilaka, F. C. Biobleaching of Industrial Important Dyes with Peroxidase Partially Purified from Garlic. Scientific World Journal 2014, 2014, 183163.
- Nar, M.; Çetinkaya, Y.; Gülçin, İ.; Menzek, A. (3,4-Dihydroxyphenyl)(2,3,4-Trihydroxyphenyl)Methanone and Its Derivatives as Carbonic Anhydrase Isoenzymes Inhibitors. Journal of Enzyme Inhibition and Medicinal Chemistry 2013, 28, 402–406.
- Akıncıoğlu, A.; Akbaba, Y.; Göçer, H.; Göksu, S.; Gülçin, İ.; Supuran, C. T. Novel Sulfamides as Potential Carbonic Anhydrase Isoenzymes Inhibitors. Bioorganic and Medicinal Chemistry 2013, 21, 1379–1385.
- Welinder, K. G.;. Superfamily of Plant, Fungal and Bacterial Peroxidases. Current Opinion in Structural Biology 1992, 2, 388–393.
- Cardinali, A.; Tursi, N.; Ligorio, A.; Giuffrida, M. G.; Napolitano, L.; Caliandro, R.; Sergio, L.; Di Venere, D.; Lattanzio, V.; Sonnante, G. Purification, Biochemical Characterization and Cloning of a New Cationic Peroxidase Isoenzyme from Artichoke. Plant Physiology and Biochemistry 2011, 49, 395–403.
- Bhatti, H. N.; Najma, A.; Asgher, M.; Hanif, M. A.; Zia, M. A. Purification and Thermal Characterization of a Novel Peroxidase from a Local Chick Pea Cultivar. Protein and Peptide Letters 2006, 13, 799–804.
- Deepa, S. S.; Arumughan, C. Purification and Characterization of Soluble Peroxidase from Oil Palm (Elaeis guineensis Jacq) Leaf. Phytochemistry 2002, 61, 503–511.
- Fatima, A.; Husain, Q. Purification and Characterization of a Novel Peroxidase from Bitter Gourd (Momordica charantia). Protein Peptide Letters 2008, 15, 377–384.
- Kim, S. S.; Lee, D. J. Purification and Characterization of a Cationic Peroxidase Cs in Raphanus sativus. Journal of Plant Physiology 2005, 162, 609–617.
- Billaud, C.; Louarme, L.; Nicolas, J. Comparison of Peroxidases from Barley Kernel (Hordeum vulgare L.) And Wheat Germ (Triticum aestivum L.): Isolation and Preliminary Characterization. Journal of Food Biochemistry 1999, 23, 145–172.
- Koksal, E.;. Peroxidase from Leaves of Spinach (Spinacia oleracea): Partial Purification and Some Biochemical Properties. International Journal of Pharmacology 2011, 7, 135–139.
- Rudrappa, T.; Lakshmanan, V.; Kaunain, R.; Singara, M. S.; Bhagyalakshmi, N. Purification and Characterization of an Intracellular Peroxidase from Genetically Transformed Roots of Red Beet (Beta vulgaris L.). Food Chemistry 2007, 105, 1312–1320.
- Cai, F.; Yang, C. O.; Duan, P.; Gao, S.; Xu, Y.; Chen, F. Purification and Characterization of a Novel Thermal Stable Peroxidase from Jatropha curcas Leaves. Journal of Molecular Catalysis B: Enzymatic 2012, 77, 59–66.
- Pandey, V. P.; Dwivedi, U. N. Purification and Characterization of Peroxidase from Leucaena leucocephala, a Tree Legume. Journal of Molecular Catalysis B: Enzymatic 2011, 68, 168–173.
- Rodriguez-Lopez, J. N.; Smith, A. T.; Thorneley, R. N. Role of Arginine 38 in Horseradish Peroxidase a Critical Residue for Substrate Binding and Catalysis. Journal of Biological Chemistry 1996, 271, 4023–4030.