ABSTRACT
The medicinal potential of the ethanol extract of Micromeria graeca (L.) Benth. ex Rchb., harvested in Algeria (MG extract), was evaluated by assessing in vitro its antioxidant, antibacterial, and antityrosinase properties. The total phenolic (= 430 ± 30 mg gallic acid equivalent/100 g of dry weight) and flavonoid (= 190 ± 10 mg quercetin equivalent/100 g of dry weight) contents were evaluated by the Folin–Ciocalteu and the aluminium chloride methods, respectively. Silica gel thin-layer chromatography revealed the presence of chlorogenic, caffeic, and rosmarinic acids and diosmin; the presence of these compounds was confirmed by reverse phase high-performance liquid chromatography. The IC50 values indicate that the ethanol extract of M. graeca is highly potent in neutralizing the 2,2ʹ-azinobis (3-ethylbenzothiazoline-6-sulfonic acid) cation radicals (ABTS•+; IC50 = 30.5 ± 0.9 µg/mL) and the 2,2ʹ-diphenyl-1-picrylhydrazyl radicals (DPPH•; IC50 = 65.8 ± 2.4 µg/mL). The MG extract was also very active on a lipid peroxidation model (IC50 = 23.4 ± 1.9 µg/mL). The superoxide quenching index values (electrochemically measured) were 332 ± 37 (AI30) and 638 ± 53 µg/mL (AI50). The studied extract showed a moderate tyrosinase inhibitory activity (IC50 = 302 ± 62 µg/mL) and a low antibacterial effect while the combination of this extract with antibiotics restored the activities of cefotaxime and streptomycin on resistant Staphylococcus aureus and Pseudomonas aeruginosa.
Introduction
Plants of the genus Micromeria are perennial herbs belonging to the family Lamiaceae, tribe Mentheae, and subfamily Nepetoideae.[Citation1] This genus includes about 130 species, widespread in the Mediterranean region.[Citation2] Micromeria is part of a complex group of genera that comprises herbs, subshrubs, and shrubs with medicinal value, used in popular medicine against heart disorders, headache, wound skin, infections, and as antispasmodic, stimulant, expectorant,[Citation3] and to treat colds.[Citation4] Furthermore, the leaves of these plants have been reported to possess anti-inflammatory and antimicrobial properties.[Citation5] The genus Micromeria grows naturally in Algeria, where its leaf decoction has been generally used in folk medicine as a substitute for mint, notably in the treatment of wounds, stomach ache, colds, and fevers and as condiment.[Citation6]
As for many Lamiaceae, the studies on Micromeria sp., including Micromeria graeca,[Citation7,Citation8] have mostly concerned their essential oils; nevertheless, previous researches have established the genus Micromeria to be a rich source of polyphenolic compounds.[Citation4,Citation9] Polyphenols are bioactive molecules attracting high scientific attention. These compounds exhibit a wide range of pharmacological effects that are linked to their antioxidant properties.[Citation10,Citation11] Phenolic compounds have been reported to be excellent free radical scavengers due to their strong hydrogen-donating ability.[Citation10,Citation12]
Reviewing the literature, nothing was reported concerning the phenolics of M. graeca (L.) Benth. ex Rchb., except a few papers concerning the occurrence of flavonoidal glycosides.[Citation1,Citation13] For that reason, the objectives of the present study were (i) to analyse the phenolic composition of M. graeca (MG) collected from the North-Eastern Bejaia region in Algeria; and (ii) to investigate the antibacterial, tyrosinase inhibitory, and antioxidant activities of a polar extract of this plant.
Materials and methods
Plant material
The aerial parts were collected at the flowering stage in Smaoun (latitude: 36°36ʹN; longitude: 4°49ʹE) in Bejaia, Northeastern Algeria, August 2009. The plant specimen was further authenticated by Professor Lejoly in the Laboratory of Systematical Botany and Phytosociology, Free University of Brussels (ULB). A voucher specimen (BR 000000 6946197) has been deposited in the Herbarium of the National Botanical Garden of Meise (Belgium). The sample was dried in the shade at room temperature until constant weight and ground in an electric mill to pass a 0.25 mm sieve.
Chemicals
Folin–Ciocalteus’s phenol reagent and sodium carbonate were purchased from Biochem Chemopharma (Montreal, Quebec). Gallic acid was purchased from BDH, Prolabo (Linars del Vallés, Spain), rosmarinic acid and kaempferol were purchased from Carl Roth (Karlsruhe, Germany), apigenin-7-glucoside, apigenin, chlorogenic acid, ellagic acid, diosmin, epicatechin, and naringenin from Sigma-Aldrich (St. Louis, USA), caffeic acid from Janssen Chimica (Beerse, Belgium), ferulic acid from Fluka (Buchs, Switzerland), rutin from Alfa Aesar (Ward Hill, MA), and quercetin from Riedel-de Haen (Germany). All standards were prepared as stock solutions in methanol (1 mg/mL). Mushroom tyrosinase (5.370 U/mg), L-tyrosine, l-3,4-dihydroxyphenylalanine (L-DOPA), and kojic acid were purchased from Sigma-Aldrich (St. Louis, USA); ABTS•+ and DPPH•, N,N-dimethylformamide extra dry (≤0.005% H2O) and tetrabutyl ammonium hexafluorophosphate (electrochemical grade) from Fluka (Buchs, Switzerland); Trolox® [(±)-6-hydroxy-2,5,7,8-tetramethylchroman-2-carboxylic acid] from Acros Organics (Morris Plains, NJ, USA). The Mueller–Hinton broth was purchased from Oxoid (Hampshire, UK). Cefotaxime and streptomycin were used as control for the microdilution assays (Taastrup, Denmark). All other reagents were obtained from Sigma-Aldrich (St. Louis, USA) or Merck (Darmstadt, Germany) and were of analytical grade.
Preparation of plant extract
The aerial parts powder (25 g) was first defatted with 600 mL n-hexane, and then extracted by stirring with 400 mL of ethanol, at room temperature (130 rpm, 24 h). The crude extract was filtered. The filtrate was concentrated with a rotary vacuum evaporator, lyophilized, and kept in the dark at +4°C until tested. The extraction yield was 4.8%.
Estimation of the content in phenolic compounds
Total phenolics content
Total phenolic content (TPC) was estimated as gallic acid equivalents according to the Folin–Ciocalteu method as described in our previous study.[Citation14] First, 100 µL of sample (1 mg/mL) or gallic acid (1–10 µg/mL) were transferred to a 10.0 mL volumetric flask containing 6.0 mL of H2O, to which were subsequently added 500 µL of undiluted Folin–Ciocalteu reagent. After 1 min, 1.5 mL of 20% aqueous Na2CO3 was added, and the volume was made up to 10.0 mL with H2O. The control contained all the reagents except the extract. After 2 h incubation at 25°C, the absorbance was measured at 760 nm and compared to a gallic acid calibration curve. TPC was determined as milligram gallic acid equivalent per 100 g of dry matter (mg GAE/100 g DM).
Total flavonoids content
Total flavonoids content (TFC) was measured by a colorimetric assay as reported in our previous study.[Citation14] 1.5 mL of diluted sample (250 µg/mL) or standard solution (5–25 µg/mL) was added to 1.5 mL of AlCl3 solution (2%) and mixed. After 15 min, the absorbance of the mixture was determined at 430 nm against the blank. TFC was expressed as milligram quercetin equivalent per 100 g of dry matter (mg QE/100 g DM), based on the quercetin calibration curve.
Determination of antioxidant activity
Inhibition of linoleic acid peroxidation
The inhibition of the Fenton reaction-induced linoleic acid peroxidation was measured by an indirect in vitro colorimetric method.[Citation15] The reaction mixture contained 125 µL phosphate buffer (0.4 M, pH 6.75, air-saturated), 25 µL of extract solutions (serial dilutions ½ in methanol; initial concentration, 10 mg/mL), 10 µL of hydrogen peroxide (16 mM), 10 µL of ferrous sulfate (16 mM)–EDTANa2H2 (15 mM), and 80 µL of linoleic acid (8 mM in 50 mM borate, nitrogen-saturated, pH 9). After 10 min at room temperature in the dark, 75 µL of each reaction well were transferred to another plate containing 150 µL of a benzoyl leucomethylene blue–haemoglobin solution (5 mg benzoyl leucomethylene blue, in 8 mL dimethyl formamide, added to 80 mL phosphate buffer, 0.2 M, pH 5, containing 1.4 g Triton X-100 and 5.6 mg of haemoglobin; diluted to 100 mL with phosphate buffer; to be prepared extemporaneously, strictly observing the order of reagents addition) per well. After 30 min at room temperature in the dark, the absorbance was measured with a LabsystemsiEMS reader/dispenser MF (Labsystems, Finland) at 660 nm. All the experimental results obtained from two separate experiments in triplicate were fitted to a parametric function in Systat 7.1 (Systat Software) as described in . The IC50 (the concentrations of plant extract that inhibit 50% of methylene blue formation) were computed. Results were expressed as Trolox® equivalent antioxidant capacity (TEAC), calculated as IC50Trolox®/IC50 plant extract.
Figure 1. Lipid peroxidation model of M. graeca extract. The experimental results obtained from two separate experiments in triplicate were fitted to a parametric function in Systat 7.1 (Systat Software), N = N° × exp(–k × C), where C is the concentration, N is the percentage of linoleic acid remaining at concentration C, N° is the percentage of linoleic acid at concentration 0, and k is the parameter. Trolox® was used as a reference.

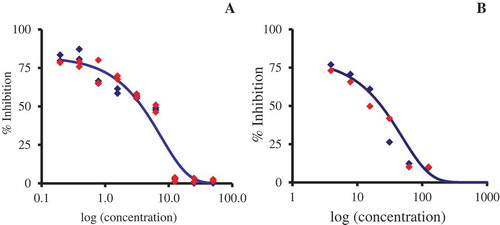
Quenching of the ABTS•+ radical cation
The TEAC was performed using discoloration assay.[Citation14] ABTS•+ radical cation was produced by reacting ABTS stock solution of 7 and 2.45 mM potassium persulfate and allowing the mixture to stand in the dark at room temperature for 12–16 h before use. The solution was then diluted to obtain an absorbance of 0.70 ± 0.02 units at 734 nm. Ten microliters of extract (10–60 µg/mL) were added to 990 μL of ABTS•+ solution, and the decrease in absorbance at 734 nm was monitored for 30 min. The TEAC of gallic acid (2–6 mM) and quercetin (5–10 mM) samples were also assayed for comparison. A concentration–response curve as a function of different Trolox® concentrations (5–20 µM) was used for calculating the TEAC. All determinations were performed in triplicate. The percentage inhibition was calculated as:
where Abscontrol is the absorbance of ABTS•+ + ethanol and Abssample is the absorbance of ABTS•+ + sample extract/standard.
Quenching of the DPPH• radical
The DPPH• radical-scavenging activity was measured using the technique described in our previous study.[Citation14] 0.5 mL of the DPPH• solution (1.10–3 M) was added to 1 mL of plant extract (10–80 µg/mL) or 1 mL of ethanol. The mixture was incubated at room temperature in the dark. The decrease in absorbance of the resulting solution was monitored at 517 nm at 60 min. The scavenging activity was estimated based on the percentage of DPPH• radical scavenged as the following equation:
The IC50 value is the effective concentration that could scavenge 50% of the DPPH• radicals. Gallic acid (10–80 µg/mL), quercetin (0.5–2.5 µg/mL), and Trolox® (2.0–8.0 µg/mL) standards were used as positive references.
Quenching of electrochemically generated superoxide anion
Cylic voltammetry experiments were performed on dual potentio-galvanostat PGSTAT 100 (Autolab instrument, Eco Chemie B.V., Utrecht, The Netherlands). All measurements were carried out on a three-electrode thermostated cell. A glassy carbon disk working electrode (diameter 2 mm), a platinum wire counter electrode, and a reference electrode, Ag/AgCl in EtOH saturated by LiCl were used for all electrochemical experiments. The reference electrode was separated from the solution by a salt bridge containing 0.5 M Bu4NPF6 in N,N-dimethylformamide (DMF). The glassy carbon disk working electrode was polished using silicon carbide 4000 paper with a laboPol-5 (Struers, Ballerup, Denmark), washed with distilled water, and then dried. For all measurements, the temperature was maintained at 20 ± 0.02°C with a Julabo heating circulator MP-5 (Julabo, Seelbach, Germany).
A voltammetric technique was used to generate O2•- by reduction of molecular oxygen in an aprotic medium (DMF extra dry (≤0.01%H2O)). The reactivity of the radical O2•- was directly measured in cyclic voltammetry at the backward scan by the anodic current decay from its oxidation in the presence of extract.
A solution of 10 mL of an extra dry DMF containing the supporting electrolyte 0.1 M Bu4NPF6 was saturated by dry air during 10 min. In these conditions, the solubility of oxygen was assumed to be CO2 ≈ 9.4 × 10–4 mol/L, this value corresponding to a partial pressure of 0.2 bar.[Citation14] The cyclic voltammogram (CV) of the oxygen reduction was then recorded at a scan rate 0.1 V/s, with the initial potential at 0 V and the reverse one at −1.5 V vs. Ag/AgCl.
Stock solution of the plant extract was prepared at about 5 mg/mL. Aliquots of stock solution were successively added to the 10 mL oxygen solution in order to get a plant extract concentration in the range of 50–600 µg/mL. The CV of oxygen without phenolic compounds or extract is first recorded in order to get the initial cathodic and anodic peak currents. Then, successive aliquot additions are performed and CV recorded at a scan rate of 0.1 V/s after each aliquot addition.
Inhibition of tyrosinase activity
The tyrosinase inhibitory activity of the extract was evaluated by using L-DOPA.[Citation14,Citation16] In a 96-well microplate, 80 µL of the sample (50, 100, and 500 µg/mL) or kojic acid (142 and 1421 µg/mL) dissolved in 20 mM phosphate buffer, pH 6.8, were rapidly added with 90 µL of mushroom tyrosinase (45 units/mL, dissolved in the pH 6.8 buffer). After the rapid addition of 80 µL of a mixture of L-DOPA/L-tyrosine in the same buffer and incubation at 37°C for 5 min, the absorbances were measured at 450 nm every 20 min over 180 min with a Multi-Mode Microplate Reader (Multiskan Ex., USA). The following equation was used to determine the inhibition of the tyrosinase activity.
Determination of the antibacterial activity
The antibacterial activity was screened against Escherichia coli ATCC 25922, Pseudomonas aeruginosa ATCC 9027, Staphylococcus aureus ATCC 6538 obtained from the American Type Culture Collection, and S. aureus C 100459, a methicillin-resistant S. aureus (MRSA) clinical isolate, a generous gift from the Centre Hospitalier Universitaire of Charleroi, Belgium.
The direct antibacterial effect was evaluated by a broth microdilution method according to Okusa et al.;[Citation17] the plant extract was dissolved in DMSO (20 mg/250 μL) and diluted to 5 mL with Mueller–Hinton broth, the final DMSO concentration being 5%. This solution was transferred in 96-well plates (200 μL/well) and serially diluted with Mueller–Hinton broth. Bacterial strains cultures were stirred with 0.9% NaCl to achieve 0.5 McFarland (108 cells/mL), diluted 1/100 to achieve 106 cells/mL, and inoculated in the 96-well plates (100 μL/well). The cultures were incubated at 37°C for 24 h in an oven. The minimum inhibitory concentration (MIC) was the lowest concentration of extract that completely inhibited the growth of microorganisms in the microdilution wells, detected by the unaided eye; the minimal bactericidal concentration (MBC) was determined by sub-culturing the negative wells on a Mueller–Hinton agar plate as the lowest concentration that yielded negative sub-cultures. Controls were set up with streptomycin and cefotaxime.
The analysis of antibacterial combinations analysis was performed according to Okusa et al. [Citation17] This requires experimental data for agents used alone and in different dose combinations at equieffective levels. The strains S. aureus ATCC 6538 and P. aeruginosa ATCC 9027 were used to test the combination of antibiotics and MG extract. Compounds were placed into 96-well tissue culture plate to obtain mixtures covering a broad range of suboptimal concentrations of both compounds.
Identification of phenolic compounds
TLC analysis
The qualitative investigation of the MG extract with regards to the presence of flavonoids and phenolic acids was carried out on Silica gel 60 F254 plates 0.25 mm (Merck, Germany), using ethyl acetate–formic acid–water (90:6:6, v/v/v) as a solvent system. After spraying the developed plates with a 1% methanolic solution of diphenyl-boric acid-ethanolamine complex and additionally with a 5% ethanolic solution of PEG 400, the plates were visualized under UV 254/366 and daylight.[Citation18]
RP-HPLC analysis
Separation and identification of the polyphenolic components in the extract were carried out by HPLC (Kontron), on a silica-based C18-bonded phase column (Alltima 250 × 4.6 i.d. mm; 5 µm; Grace Alltech, Columbia, USA) at 40°C, using UV detector operating at different wavelengths (280 and 330 nm). The injection system used was a 20 µL sample loop. The solvent system comprised mobile phases A (acetonitrile: H2O, 19:80, v/v with CH3COOH 1%) and B (acetonitrile: MeOH, 59:40, v/v with CH3COOH 1%) at a flow rate of 1.2 mL/min. The elution gradient was 0–12% B, 0–8 min; 12–12% B, 8–12 min; 12–45%, 12–20 min; 45–100% B, 20–35 min; 100–0% B, 35–40 min. Reference standards were used for the identification of major phenolic compounds in the sample.
Statistical analysis
The experimental results were given as mean ± SD of three replicate measurements. The experimental values were evaluated by using one-way analyses of variance (ANOVA). p values < 0.05 were regarded as ‘significant’. The eventual correlations of the radical scavenging (ABTS•+, DPPH•, and O2•-) and inhibition (linoleic acid peroxidation and tyrosinase) capacities with total phenolics and flavonoids were also analysed.
Results and discussion
Total phenolic and flavonoid contents
The TPC of Algerian MG is 430 ± 30 mg GAE/100 g dry weight. The most widespread and diverse phenolics are flavonoids, polyphenolic secondary metabolites widely dispersed throughout the plant kingdom. The TFC of MG was 190 ± 10 mg in terms of quercetin equivalents/100 g of dry weight. As far as we know, there are no reports on the TPC and TFC of MG extract.
Vladimir-Knežević et al.,[Citation19] studying three Micromeria species (Micromeria croatica, Micromeria juliana, and Micromeria thymifolia) from Croatia, showed that the total polyphenols in all plant samples were considerable, ranging between 9.7% and 13.1%. The most abundant compounds were phenolic acids (5.3–6.8%), followed by tannins (3.1–6.1%), contrasting with a very low amount of flavonoids (0.01–0.09%).
Antioxidant activity
In complex biological systems, various mechanisms may contribute to oxidative processes, for example, electrons leakages from electron-transport chains, photodynamical effects or Fenton reactions, in which transition metal ions play a major role; different reactive oxygen species (ROS) might be generated targeting various biomolecules, lipids, proteins, and nucleic acids. Depending on their site of generation (aqueous phase or lipid membranes), the disposal of ROS is quite different. Therefore, it is important to characterize the extracts by a variety of antioxidant assays.[Citation20] To the best of our knowledge, the antioxidant potentialities of MG extract have seldom been studied; Couladis et al.[Citation21] showed, by a method based on the peroxidation of arachidonic acid, that the methanol extract of MG was similar in activity to α-tocopherol. In the present work, four antioxidant assays were carried out.
Inhibition of linoleic acid peroxidation
Lipid peroxidation can be inhibited by suppressing chain initiation and chain propagation and/or by enhancing chain termination. A haemoglobin-catalysed peroxidation of linoleic acid assay indicated the ability of MG extract to protect unsaturated fatty acids from peroxidation. Curve fitting () allowed computing IC50 values for the plant extract and Trolox® (); the IC50 value (23.4 ± 1.9 µg/mL) indicates the ability of the plant extract to inhibit lipid peroxidation and corresponds to 0.16 Trolox® equivalents. Previous results obtained on Micromeria nervosa (Desf.) Benth. extracts and isolated compounds indicate anti-lipid peroxidation activities.[Citation4] The acetone extract of Micromeria cilicica Hausskn. ex P.H. Davis (a synonym of Clinopodium cilicicum (Hausskn. ex P.H. Davis) Bräuchler & Heubl.) showed a strong inhibition of lipid peroxidation in a β-carotene bleaching assay, with quasi equivalence to (+)-catechin.[Citation22] The methanolic extract of Micromeria fruticosa ssp. serpyllifolia (M. Bieb.) P. H. Davis inhibited linoleic acid oxidation, while the essential oil of this species exhibited practically no activity.[Citation23]
Table 1. Antioxidant activity and tyrosinase inhibition of M. graeca extract and standards.
Quenching of the ABTS•+ and DPPH• radicals
The ABTS•+ and DPPH• assays, based on single-electron transfer mechanisms, are simple and common methods for evaluating antioxidant activities.[Citation20] The IC50 values indicate that the ethanol extract of MG is potent in neutralizing ABTS•+ (IC50, 30.5 ± 0.9 µg/mL) and DPPH• (IC50, 65.8 ± 2.4 µg/mL) radicals (). These assays indicate a hydrogen and/or electron donation capacity of the MG polar extract, an important factor to protect biomolecules from ROS in susceptible biological and food systems.
A DPPH• radical-scavenging activity was previously recorded for polar extracts of other Micromeria species. The acetone extract of M. nervosa (from Egypt) quenched DPPH• with the same potency as α-tocopherol.[Citation4] The ethanolic extracts of M. croatica, M. juliana, and M. thymifolia (from Croatia)[Citation19] and the methanolic extract of M. fruticosa ssp. serpyllifolia (from Turkey)[Citation23] exhibited considerable scavenging of DPPH•; M. croatica was the most active species (IC50 = 4.7 μg/mL). The ethanolic extract of Micromeria myrtifolia (from Lebanon) was quite active towards DPPH•, whereas the essential oils and apolar (hexane and chloroform) extracts showed only marginal antiradical activities.[Citation3] Vladimir-Knezevic et al.[Citation19] showed that the radical-scavenging activity of Micromeria species largely depends on their major compound, rosmarinic acid, a powerful free antioxidant.
Quenching of the O2•- radical
This assay for antioxidant capacity, based on the quenching of electrogenerated superoxide, was previously applied to seaweed’s, mint, and Ericaceae extracts.[Citation14,Citation24,Citation25] Biological samples are complex mixtures of compounds producing a broad voltammetric wave; the area beneath the major anodic peak correlates with the number of electrons used up in the oxidation reaction and so depends on the quenching capacity of the antioxidant sample.[Citation26] The measurement of the antioxidant activity was estimated by antioxidant index values AI30 and AI50 (), defined as the extract concentrations needed to consume 30% or 50% of the electrogenerated radical, respectively [corresponding to (Ipa° – IpaS)/Ipa° = 0.3 or 0.5 where Ipa° is the intensity of the anodic current peak of O2•- and IpaS is the intensity of the anodic current peak of O2•- for the concentration S of the sample]. With this characterization, the lower the AI30 or AI50 value, the more the sample has a strong reactivity towards superoxide ().
The content of total phenolics correlated with quenching of ABTS•+ (R2 = 0.996), superoxide (R2 = 0.992), and lipid peroxidation (R2 = 0.977), but not with DPPH• (R2 = 0.093). By contrast, the content of total flavonoids correlated with quenching of DPPH• (R2 = 0.770) and neither with ABTS•+ (R2 = 0.059), superoxide (R2 = 0.075), nor lipid peroxidation (R2 = 0.111). The measured AI30 and AI50 () were considerably higher than the concentrations required to quench DPPH• and ABTS•+, indicating a lower antioxidant capacity towards superoxide. In line with previous reports on Mentha[Citation14] and Erica[Citation25] species, these data confirm that there is no correlation between the reactivity towards large stable radicals (neutral or positively charged) and the reactivity towards the small, chemically reactive and negatively charged superoxide radical. Because of their simplicity, methods based on stable radicals are widely used but we agree with the conclusions of Tohaya and Ochiai,[Citation27] who preconized caution in interpreting such data. Our data corroborate those of Vladimir-Knezevic et al.,[Citation19] who showed that the obvious antioxidant capabilities of tested Micromeria species were not correlated to flavonoids but to phenolic acids, notably rosmarinic acid.
Inhibition of tyrosinase
Tyrosinase is the limiting enzyme in the series of reactions leading to melanin production. As melanin overproduction is observed in many skin disorders, the inhibition of tyrosinase activity would appear to be the most rational and explicit way of overcoming these issues.[Citation28] Also many skin-whitening agents, mostly based on chemical tyrosinase inhibitors (hydroquinone and kojic acid, notably), are used by African and Asian populations with many adverse and toxic effects; the search for natural tyrosinase inhibitors may lead to safer cosmetics.[Citation16] Compared to kojic acid, a reference inhibitor (IC50, 8.9 µg/mL), the MG extract was found to exhibit a moderate tyrosinase inhibition with IC50 value of 302 ± 62 µg/mL. As tyrosinase catalyses oxidative reactions,[Citation11] there should be a close relationship between the antioxidant phenolic compounds of natural extracts and their inhibitory effects.[Citation28,Citation29] Previous studies have supported the role of metal ion chelation in the process of tyrosinase inhibition, and a linear correlation was found between chelating activity and tyrosinase inhibition.[Citation30] Polyphenolic compounds, such as flavonoids and acid phenols, are known to form complexes with metal ions to exhibit antityrosinase action.[Citation29]
Antibacterial activity
The antibacterial activity of the MG extract was tested against four pathogenic bacteria, namely E. coli ATCC 25922, P. aeruginosa ATCC 9027, S. aureus ATCC 6538, and S. aureus C 100459; presents the direct antibacterial effects of the extract, measured by a broth microdilution technique. The extract was inactive against all tested microorganisms (MIC ˃ 2000 µg/mL). Published data generally indicate that apolar extracts from plants are considerably more antimicrobial than polar extracts. This is often true for plants belonging to the genus Micromeria; in fact, the antibacterial activity of these plants is mostly due to their essential oils rather than polar compounds. For instance, apolar extracts and essential oils of M. cilicica Hausskn. ex P. H. Davis (origin, Turkey) showed antibacterial activity against all of the tested bacteria (agar diffusion test; 10–25 µL essential oils; inhibition diameters, 9–21 mm).[Citation31] The essential oils from herbal parts of Micromeria cristata subsp. Phrygia P.H. Davis collected from three different Turkish localities were active against all pathogenic microorganisms tested (MICs, 31–250 µg/mL).[Citation32] According to Ali-Shtayeh et al.,[Citation5] the least active extract of M. nervosa (Desf.) Benth. (origin, Palestine) was the freeze-dried water extract.
Table 2. The minimal inhibitory concentrations MICs (µg/mL) and minimal bactericide concentrations MBCs (µg/mL) of M. graeca extract and antibiotics.
The lack of significant activity of our polar MG extract may be related, at least in part, to hydrophilicity, a factor that may reduce recognition and transport of active compounds. Indeed lipophilicity appears as a key factor for bacterial inhibition, but other factors, such as structural features of the compounds, have to be considered.[Citation33] According to Rauha et al.,[Citation34] prenyl-substituted flavanones have been shown to possess more effective antibacterial activity against S. aureus than simple flavanones and flavonols; flavonoid glycosides (different glycosides of quercetin), such as found in polar extracts, notably failed to show any activity against the microbial strains tested.[Citation34] Regarding tannins, which are generally known to present antimicrobial activity, paradoxically some organisms are capable of using tannins as a carbon source.[Citation34]
Multidrug therapy has become of paramount importance in the fight against multidrug resistant microbial strains[Citation35] and such synergies will probably play an important role in the coming years. Extracts contain complex mixtures of components and thus have multiple antimicrobial properties, direct but also indirect, for example, by restoring the efficacy of antibiotics on multi-resistant strains[Citation17] or by modulating bacterial communication mechanisms and biofilm formation.[Citation36] The combination of the MG extract with antibiotics restored or enhanced the activities of streptomycin and cefotaxime by factors 4–32 (). The obtained data are in line with growing evidence that phenolic compounds (notably flavonoids) act synergistically with various antibiotics against multidrug-resistant microorganisms. For example, in vitro, two flavonols (kaempferol and quercetin) synergized with rifampicin against MRSA clinical isolates; combining these flavonols with rifampicin significantly increased their β-lactamase inhibitory effect.[Citation37] The hydroxylation and glycosylation patterns strongly influence this activity.[Citation38] On the other hand, lipophilicity is a common feature of several active compounds; this quality is important for their solubilization in bacterial membranes. The amphipathic kaempferol glycoside modulated the activities of the drugs by reducing the concentrations needed to inhibit the growth of bacteria.[Citation33]
Table 3. The effect of combination between M. graeca extract (MG) and antibiotics on the minimal inhibitory concentrations MICs (µg/mL).
Identification of polyphenolic compounds
TLC screening of the MG crude extract before and after acid hydrolysis indicated the presence of phenolic acids and flavonoids. Four phenolic acids were identified as rosmarinic, caffeic, chlorogenic, and gallic acids, verified by their intense blue fluorescent bands in UV light at 365 nm (Rf values 0.77, 0.80, 0.30, and 0.70, respectively). The presence of the phenol acids was confirmed by HPLC-UV, with rosmarinic acid as the most abundant compound (). The phenolic acids such as gallic, chlorogenic, vanilic, hydroxy benzoic, sinapic, ferulic, p-coumaric, cinnamic, and ellagic acids were revealed previously in Micromeria species.[Citation1,Citation9,Citation13,Citation19,Citation39]
Figure 2. High-performance liquid chromatography profile of M. graeca extract. (1) gallic acid; (2) caffeic acid; (3) chlorogenic acid; (4) rosmarinic acid; (5) diosmin; (6) apigenin-7-glucoside; (7) apigenin. Detection at 280 nm.
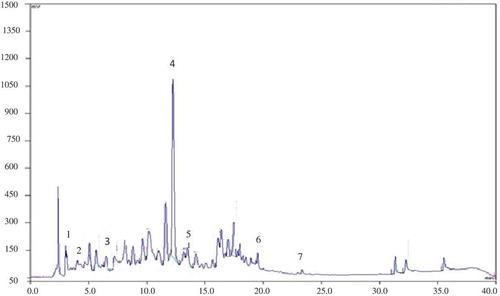
After derivatization, the flavonoids were detected on TLC as orange-yellow zones with distinct orange fluorescence. The flavonoids detected in the raw ethanolic MG extract do not match the standards used; after hydrolysis, two compounds were identified as apigenin and diosmin (Rf values 0.88 and 0.43, respectively). By comparing retention times and UV spectral characteristics with reference compounds, the flavonoids revealed by HPLC analysis in the MG extract were diosmin, apigenin-7-glucoside, and apigenin (). Tomas-Barberan et al.[Citation13] also detected in MG flavonoid glycosides as major constituents (luteolin and apigenin glycosides). A literature survey revealed that Micromeria sp. flavonoids are derivatives of acacetin, apigenin, and luteolin.[Citation1,Citation13,Citation19] Furthermore, previously, Micromeria species were reported to contain various flavonoids: isosakuranetin 7-rutinoside, naringenin,[Citation40] 5,6,4ʹ-trihydroxy-7,3ʹ-dimethoxyflavone, thymusin, thymonin, genkwanin, 5,6-dihydroxy-7,8,3ʹ,4ʹ-tetramethoxyflavone, ladanein, 5,4ʹ-dihydroxy-6,7,8,3ʹ-tetramethoxyflavone, 5-demethylnobiletin, 5-hydroxy-6,7,3ʹ,4ʹ-tetramethoxyflavone, chrysoeriol,[Citation41] 5,6,4ʹ-trihydroxy-7,3ʹ-dimethoxyflavone, 5,6-dihydroxy-7,3ʹ,4ʹ-trimethoxyflavone,[Citation42] myrcetin, hesperetin, and quercetin.[Citation9]
Table 4. Phenolic compounds identified by HPLC in M. graeca extract.
These metabolites were shown to be responsible for several pharmacological activities, notably antioxidant, antimicrobial, and enzyme inhibitory.[Citation43] The most abundant compounds were characterized as rosmarinic acid, diosmin, and apigenin-7-glucoside. A broad spectrum of significant pharmacological activities have been reported for these bioactive compounds.[Citation15,Citation44] Accordingly the above-mentioned phenolics could be responsible for the antioxidant, antibacterial, and enzyme inhibitory effects we measured in our ethanolic extract.
Conclusion
This study was conducted to investigate the antioxidant and antibacterial properties of a M. graeca L.) Benth. ex Rchb. ethanolic extract. With a significant phenolic content, the MG polar extract showed important scavenging properties towards ABTS•+, DPPH•, and electrochemically generated O2•−, translating into inhibition of lipid peroxidation and tyrosinase. Although inactive towards all tested bacteria (MICs ˃ 2000 µg/mL), the MG polar extract restored the activities of cefotaxime and streptomycin on resistant S. aureus and P. aeruginosa. This promising property should be investigated in more details. The observed activities probably reflect the qualitative profile of the phenolic compounds present in this species; effectively, seven compounds (flavonoids and phenolic acids) were identified from MG, from which rosmarinic acid and diosmin were predominant.
Acknowledgement
We thank Pr. J. Lejoly for his confirmation of the identification of plant studied and all members of the Pharmacognosy, Bromatology and Human Nutrition laboratory from ULB for their help, especially M. Faes and O. Vaillant for maintaining good conditions of work in the laboratory. We thank the members of Chemical Sciences Laboratory of Rennes UMR CNRS 6226. We also thank Dr Elsebai Mahmoud Fahmi for English-language editing of the manuscript.
References
- Marin, P. D.; Grayer, R. J.; Veitch, N. C.; Kite, G. C.; Harborne, J. B. Acacetin Glycosides as Taxonomic Markers in Calamintha and Micromeria. Phytochemistry 2001, 58, 943–947. doi:10.1016/S0031-9422(01)00352-1.
- Slavkovska, V.; Couladis, M.; Bojovic, S.; Tzakou, O.; Pavlovic, M.; Lakusic, B.; Jancic, R. Essential Oil and Its Systematic Significance in Species of Micromeria Bentham from Serbia & Montenegro. Plant Systematics and Evolution 2005, 255, 1–15. doi:10.1007/s00606-005-0303-y.
- Formisano, C.; Oliviero, F.; Rigano, D.; Saab, A. M.; Senatore, F. Chemical Composition of Essential Oils and in Vitro Antioxidant Properties of Extracts and Essential Oils of Calamintha Origanifolia and Micromeria Myrtifolia, Two Lamiaceae from the Lebanon Flora. Industrial Crops and Products 2014, 62, 405–411. doi:10.1016/j.indcrop.2014.08.043.
- Abdelwahab, M. F.; Hussein, M. H.; Kadry, H. H. Cytotoxicity and Antioxidant Activity of New Biologically Active Constituents from Micromeria Nervosa Grown in Egypt. Bulletin of Faculty of Pharmacy, Cairo University 2015, 53, 185–194. doi:10.1016/j.bfopcu.2015.11.001.
- Ali-Shtayeh, M.; Al-Nuri, M.; Yaghmour, -R. M.-R.; Faidi, Y. Antimicrobial Activity of Micromeria Nervosa from the Palestinian Area. Journal of Ethnopharmacology 1997, 58, 143–147. doi:10.1016/S0378-8741(97)00088-3.
- Benomari, F. Z.; Djabou, N.; Medbouhi, A.; Khadir, A.; Bendahou, M.; Selles, C.; Desjobert, J. M.; Costa, J.; Muselli, A. Chemical Variability and Biological Activities of Essential Oils of Micromeria Inodora (Desf.). Benth. From Algeria. Chemistry & Biodiversity. 2016, 13, 1559–1572. doi:10.1002/cbdv.201600098.
- Tzakou, O.; Couladis, M. The Essential Oil of Micromeria Graeca (L.) Bentham Et Reichenb. Growing in Greece. Flavour and Fragrance Journal 2001, 16, 107–109. doi:10.1002/(ISSN)1099-1026.
- Vuko, E.; Dunkić, V.; Bezić, N.; Ruscić, M.; Kremer, D. Chemical Composition and Antiphytoviral Activity of Essential Oil of Micromeria Graeca. Natural Product Communications 2012, 7, 1227–1230.
- Al-Hamwi, M.; Aboul-Ela, M.; El-Lakany, A.; El-Achi, N.; Ghanem, N.; El Hamaoui, B.; Bakkour, Y.; El-Omar, F. Chemical Composition, Antimicrobial and Antioxidant Activities of the Ethanolic Extract of Micromeria Fruticosa Growing in Lebanon. International Journal of Chemical Sciences 2015, 13 (1), 325–335.
- Abdullah, M. Z.; Mohd Ali, J.; Abolmaesoomi, M.; Abdul-Rahman, P. S.; Hashim, O. H. Anti-Proliferative, in Vitro Antioxidant and Cellular Antioxidant Activities of the Leaf Extracts from Polygonum Minus Huds: Effects of Solvent Polarity. International Journal of Food Properties 2017, 1–17. doi:10.1080/10942912.2017.1315591.
- Prasad, K. N.; Yang, B.; Shi, J.; Yu, C.; Zhao, M.; Xue, S.; Jiang, Y. Enhanced Antioxidant and Antityrosinase Activities of Longan Fruit Pericarp by Ultra-High-Pressure-Assisted Extraction. Journal of Pharmaceutical and Biomedical Analysis 2010, 51, 471–477. doi:10.1016/j.jpba.2009.02.033.
- Chen, M.; Zhu, Y.; Zhang, H.; Wang, J.; Liu, X.; Chen, Z.; Zheng, M.; Liu, B. Phenolic Compounds and the Biological Effects of Pu-Erh Teas with Long-term Storage. International Journal of Food Properties 2016, 1–14. doi:10.1080/10942912.2016.1217877.
- Tomas-Barberan, F. A.; Gil, M. I.; Marin, P. D.; Tomas-Lorente, F. Flavonoids from Some Yugoslavian Micromeria Species: Chemotaxonomical Aspects. Biochemical Systematics and Ecology 1991, 19, 697–698. doi:10.1016/0305-1978(91)90088-H.
- Brahmi, F.; Hauchard, D.; Guendouze, N.; Madani, K.; Kiendrebeogo, M.; Kamagaju, L.; Stévigny, C.; Chibane, M.; Duez, P. Phenolic Composition, in Vitro Antioxidant Effects and Tyrosinase Inhibitory Activity of Three Algerian Mentha Species: M. Spicata (L.), M. Pulegium (L.) And M. Rotundifolia (L.) Huds (Lamiaceae). Industrial Crops and Products 2015, 74, 722–730. doi:10.1016/j.indcrop.2015.04.038.
- Brahmi, F.; Madani, K.; Stévigny, C.; Chibane, M.; Duez, P. Algerian Mint Species: HPTLC Quantitative Determination of Rosmarinic Acid and in Vitro Inhibitory Effects on Linoleic Acid Peroxidation. Journal of Coastal Life Medicine 2014, 2 (12), 986–992.
- Kamagaju, L.; Morandini, R.; Gahongayire, F.; Stévigny, C.; Ghanem, G.; Pirotte, G.; Duez, P. Survey on Skin‐Lightening Practices and Cosmetics in Kigali, Rwanda. International Journal of Dermatology 2016, 55, 45–51. doi:10.1111/ijd.12833.
- Okusa, P.; Penge, O.; Devleeschouwer, M.; Duez, P. Direct and Indirect Antimicrobial Effects and Antioxidant Activity of Cordia Gilletii De Wild (Boraginaceae). Journal of Ethnopharmacology 2007, 112, 476–481. doi:10.1016/j.jep.2007.04.003.
- Wagner, H.; Bladt, S. Plant Drug Analysis: A Thin Layer Chromatography Atlas; Springer Science & Business Media: Berlin, Germany, 1996.
- Vladimir-Knežević, S.; Blažeković, B.; Bival Štefan, M.; Alegro, A.; Kőszegi, T.; Petrik, J. Antioxidant Activities and Polyphenolic Contents of Three Selected Micromeria Species from Croatia. Molecules 2011, 16, 1454–1470. doi:10.3390/molecules16021454.
- Kwon, S. H.; Wang, Z.; Hwang, S. H.; Kang, Y.-H.; Lee, J.-Y.; Lim, S. S. Comprehensive Evaluation of the Antioxidant Capacity of Perilla Frutescens Leaves Extract and Isolation of Free Radical Scavengers Using Step-Wise HSCCC Guided by DPPH-HPLC. International Journal of Food Properties 2017, 1–14. doi:10.1080/10942912.2017.1318289.
- Couladis, M.; Tzakou, O.; Verykokidou, E.; Harvala, C. Screening of Some Greek Aromatic Plants for Antioxidant Activity. Phytotherapy Research 2003, 17, 194–195. doi:10.1002/(ISSN)1099-1573.
- Öztürk, M.; Kolak, U.; Topçu, G.; Öksüz, S.; Choudhary, M. I. Antioxidant and Anticholinesterase Active Constituents from Micromeria Cilicica by Radical-Scavenging Activity-Guided Fractionation. Food Chemistry 2011, 126, 31–38. doi:10.1016/j.foodchem.2010.10.050.
- Güllüce, M.; Sökmen, M.; Şahin, F.; Sökmen, A.; Adigüzel, A.; Özer, H. Biological Activities of the Essential Oil and Methanolic Extract of Micromeria Fruticosa (L) Druce Ssp Serpyllifolia (Bieb) PH Davis Plants from the Eastern Anatolia Region of Turkey. Journal of the Science of Food and Agriculture 2004, 84, 735–741. doi:10.1002/(ISSN)1097-0010.
- Blanc, N.; Hauchard, D.; Audibert, L.; Gall, E. A. Radical-Scavenging Capacity of Phenol Fractions in the Brown Seaweed Ascophyllum Nodosum: An Electrochemical Approach. Talanta 2011, 84, 513–518. doi:10.1016/j.talanta.2011.01.034.
- Guendouze-Bouchefa, N.; Madani, K.; Chibane, M.; Boulekbache-Makhlouf, L.; Hauchard, D.; Kiendrebeogo, M.; Stévigny, C.; Okusa, P. N.; Duez, P. Phenolic Compounds, Antioxidant and Antibacterial Activities of Three Ericaceae from Algeria. Industrial Crops and Products 2015, 70, 459–466. doi:10.1016/j.indcrop.2015.03.053.
- Piljac-Žegarac, J.; Valek, L.; Stipčević, T.; Martinez, S. Electrochemical Determination of Antioxidant Capacity of Fruit Tea Infusions. Food Chemistry 2010, 121, 820–825. doi:10.1016/j.foodchem.2009.12.090.
- Tohaya, Y.; Ochiai, T. A Correlation between the Superoxide Anion Scavenging Capacity of Antioxidants and Their Antioxidant Capacity as Measured by the Galvinoxyl or DPPH Method. Japanese Journal of Food Chemistry and Safety 2013, 20, 61–65.
- Ullah, S.; Son, S.; Yun, H. Y.; Kim, D. H.; Chun, P.; Moon, H. R. Tyrosinase Inhibitors: A Patent Review (2011-2015). Expert Opinion on Therapeutic Patents 2016, 26, 347–362. doi:10.1517/13543776.2016.1146253.
- Wang, B.-S.; Juang, L.-J.; Yang, -J.-J.; Chen, L.-Y.; Tai, H.-M.; Huang, M.-H. Antioxidant and Antityrosinase Activity of Flemingia Macrophylla and Glycine Tomentella Roots. Evidence-Based Complementary and Alternative Medicine 2012, 2012, 1–7. doi:10.1155/2012/431081.
- Kubo, I.; Kinst-Hori, I.; Chaudhuri, S. K.; Kubo, Y.; Sánchez, Y.; Ogura, T. Flavonols from Heterotheca Inuloides: Tyrosinase Inhibitory Activity and Structural Criteria. Bioorganic & Medicinal Chemistry 2000, 8, 1749–1755. doi:10.1016/S0968-0896(00)00102-4.
- Duru, M. E.; Öztürk, M.; Uğur, A.; Ceylan, Ö. The Constituents of Essential Oil and in Vitro Antimicrobial Activity of Micromeria Cilicica from Turkey. Journal of Ethnopharmacology 2004, 94, 43–48. doi:10.1016/j.jep.2004.03.053.
- Tabanca, N.; Kırımer, N.; Demirci, B.; Demirci, F.; Başer, K. H. C. Composition and Antimicrobial Activity of the Essential Oils of Micromeria Cristata Subsp. Phrygia and the Enantiomeric Distribution of Borneol. Journal of Agricultural and Food Chemistry 2001, 49, 4300–4303. doi:10.1021/jf0105034.
- Falcão‐Silva, V. S.; Silva, D. A.; Souza, M. D. F. V.; Siqueira‐Junior, J. P. Modulation of Drug Resistance in Staphylococcus Aureus by a Kaempferol Glycoside from Herissantia Tiubae (Malvaceae). Phytotherapy Research 2009, 23, 1367–1370. doi:10.1002/ptr.2695.
- Rauha, J.-P.; Remes, S.; Heinonen, M.; Hopia, A.; Kähkönen, M.; Kujala, T.; Pihlaja, K.; Vuorela, H.; Vuorela, P. Antimicrobial Effects of Finnish Plant Extracts Containing Flavonoids and Other Phenolic Compounds. International Journal of Food Microbiology 2000, 56, 3–12. doi:10.1016/S0168-1605(00)00218-X.
- Van Vuuren, S.; Viljoen, A. Plant-Based Antimicrobial Studies–Methods and Approaches to Study the Interaction between Natural Products. Planta Medica 2011, 77, 1168–1182. doi:10.1055/s-0030-1250736.
- Rasamiravaka, T.; Labtani, Q.; Duez, P.; El Jaziri, M. The Formation of Biofilms by Pseudomonas Aeruginosa: A Review of the Natural and Synthetic Compounds Interfering with Control Mechanisms. BioMed Research International 2015, 2015, 1–17. Article ID 759348. doi:10.1155/2015/759348.
- Daglia, M.;. Polyphenols as Antimicrobial Agents. Current Opinion in Biotechnology 2012, 23, 174–181. doi:10.1016/j.copbio.2011.08.007.
- Lechner, D.; Gibbons, S.; Bucar, F. Plant Phenolic Compounds as Ethidium Bromide Efflux Inhibitors in Mycobacterium Smegmatis. Journal of Antimicrobial Chemotherapy 2008, 62, 345–348. doi:10.1093/jac/dkn178.
- Scognamiglio, M.; D’Abrosca, B.; Esposito, A.; Fiorentino, A. Chemical Composition and Seasonality of Aromatic Mediterranean Plant Species by NMR-based Metabolomics. Journal of Analytical Methods in Chemistry 2015, 2015, 1–9. Article ID 258570, 9 pages. doi:10.1155/2015/258570.
- Bellino, A.; Venturella, P.; Marceno, C. Naringenin and Neopocirin from Micromeria Species and Calamintha Nepeta. Fitoterapia 1980, 51, 163–165.
- Barberan, F.; Ferreres, F.; Tomas, F. TLC, UV and Acidic Treatment in the Differentiation of 5, 6-And 5, 8-Dihydroxyflavones, 3-Methoxyflavones and Flavonols. Tetrahedron 1985, 41, 5733–5740. doi:10.1016/S0040-4020(01)91379-1.
- Tomás-Barberán, F. A.; Husain, S. Z.; Gil, M. I. The Distribution of Methylated Flavones in the Lamiaceae. Biochemical Systematics and Ecology 1988, 16, 43–46. doi:10.1016/0305-1978(88)90115-9.
- Bahadori, M. B.; Dinparast, L.; Zengin, G.; Sarikurkcu, C.; Bahadori, S.; Asghari, B.; Movahhedin, N. Functional Components, Antidiabetic, anti-Alzheimer’s Disease, and Antioxidant Activities of Salvia Syriaca L. International Journal of Food Properties 2017, 20, 1761–1772. doi:10.1080/10942912.2016.1218893.
- Menichini, F.; Loizzo, M. R.; Bonesi, M.; Conforti, F.; De Luca, D.; Statti, G. A.; De Cindio, B.; Menichini, F.; Tundis, R. Phytochemical Profile, Antioxidant, Anti-Inflammatory and Hypoglycemic Potential of Hydroalcoholic Extracts from Citrus Medica L. Cv Diamante Flowers, Leaves and Fruits at Two Maturity Stages. Food and Chemical Toxicology 2011, 49, 1549–1555. doi:10.1016/j.fct.2011.03.048.