ABSTRACT
In the study, phytase was purified in three simple steps comprising ammonium sulphate precipitation, anion exchange and gel filtration chromatography from Weissella halotolerans. The enzyme was obtained with a specific activity of 227.73 EU/mg and 6.52% recovery. The molecular mass of the enzyme was determined to be 41.52 kDa. The optimum pH and temperature for the enzyme were 6.0 and 50°C, respectively. Furthermore, the effects of metal ions on the enzyme were investigated. Ag+, Zn2+, Cr2+ and Fe2+ ions inhibited phytase by 21.86, 25.63, 32.82 and 90.43%, respectively, whereas Co2+, Cu2+, Pb2+, Cd2+ ve Mn2+ ions increased the enzyme activity.
Introduction
Phytic acid (myo-inositol hexakisphosphate) is a common compound of plant-derived foods and constitute 1–5% of edible cornflakes, pollen, and hazelnut oil and seeds in weight. It is an organic form of phosphorus that constitute 60–90% of the total phosphorus content and plays an anti-nutritive role.[Citation1–Citation3] Although they are important in many physiological functions, especially in seed germination, phytates are solely considered as anti-nutritive compounds because they bind starch and proteins. Phytases affect protein digestion and interact with proteins, and even inhibit the activity of digestive enzymes. They also have a strong chelating effect on divalent minerals including Ca2+, Mg2+, Zn2+ and Fe2+.[Citation4] Although it is a strong chelator of iron and zinc in herbal foods, as a result of being a mediator for these metals, phytate actually acts as an antioxidant that reduces their free radical forms.[Citation4] The effective degradation of phytic acid is obtained through both enzymatic and non-enzymatic degradation.[Citation5] Enzymatic degradation is obtained by using phytase that was derived from a variety of fungus and bacteria. Non-enzymatic hydrolysis is used during food processing or the physical removal of phytate from phytate-rich plant seeds to reduce the phytate levels in end food products.[Citation6]
Phytase (myo-inositol hexakisphosphate phosphohydrolase E.C. 3.1.3.8) disintegrates the phosphoric monoester bonds in phytic acid and phytate. This results in a series of phosphate ester release from myo-inositol; therefore, it gained a quick worldwide acceptance as a food additive and animal feed.[Citation7] In the last two decades, it drew the attention of scientists and entrepreneurs for its nutrition, environmental protection and other biotechnological applications. Phytases have many applications in myo-inositol phosphate preparation. Moreover, they are used as precursors and soil improvers in the semi-synthesis of peroxidases in the paper industry and in plant growth.[Citation2] In addition, along with their applications in aquaculture, [Citation8–Citation10] they have important applications in human nutrition; [Citation11] hence, phytases are widely used as food and feed additives and are used to reduce phosphorus pollution in animal feed.[Citation12]
Bacterial phytases have a significant advantage over fungal phytases thanks to their high temperature stability, phytate substrate specifity, resistance to proteolysis and wide pH profile; thus, the purification and characterisation of phytases, which have thermostability and are active at neutral pH, are important in industrial applications. In the study, phytase was purified from lactic acid bacteria, Weissella halotolerans, in three steps comprising ammonium sulphate precipitation, Q-Sepharose anion exchange chromatography and Sephadex G-100 gel filtration column. The characteristic properties of the enzyme were determined and the effects of metal ions on the enzyme activity were investigated.
Material and method
Chemicals
All chemicals used in the study were reagent grade and purchased from Sigma Chemical Co. (St. Louis, MO) and Merck Millipore (Darmstadt, FRG).
Microbiological methods
Isolation of bacterial microorganisms
The standard MRS (Man, Ragosa and Sharpe) Broth and MRS Agar media were used for isolation. After the dilutions were prepared from various sources, they were inoculated into the MRS agar. The pour plate and spread plate methods were used and isolations were carried out in triplicate. Microorganisms were incubated at 33-37°C and the resultant colonies were re-inoculated to obtain pure cultures.
Conservation of the bacterial culture
A loopful of bacteria were taken from the 24-h pure culture and transferred to Eppendorf tubes containing 500 µl of 30% glycerol and 500 µl of LB (Luria-Bertani) Broth (prepared by adding 10 g tryptone, 10 g NaCl and 5 g yeast extract to 1 L dH2O) and labelled; then, they were stirred in a stirrer and stored at –80°C for future use. Later, the bacteria grown on MRS agar were inoculated into the prepared MRS Broth (Oxoid) medium to carry out their transfer to a liquid medium and left for incubation at 33-35°C for 7 days.
Determination of some genetic properties of the isolates
For DNA sequencing, 16S rDNA regions were amplified with PCR. The resultant amplicons were sent to Macrogen Inc. for service procurement.
The Isolation of the Genomic DNA of the Isolates: The young cultures of the isolates were prepared for genomic DNA isolation. DNA isolation from the bacteria that were precipitated by centrifuging the young cultures was carried out based on the method.[Citation13]
The Amplification of the 16S rRNA gene with PCR: The region that synthesises 16s rRNA, which is valuable for the bacteria systematics, was chosen as the target region and amplified by using universal primers [27F (forward 5ˊ-AGA GTT TGA TCC TGG CTC AG-3ˊ), 1492R (reverse 5ˊ-GGT TAC CTT GTT ACG ACT T-3ˊ)] under in vitro conditions.[Citation13,Citation14]
Determination of the Base Sequence of the 16S rRNA Gene and Its Comparison to The Sequences in the GenBank Database: The base sequence analysis of the 16S rDNA genes amplified with PCR was carried out with automated sequence analysers (Automated sequence analysis was procured from Macrogen, Inc.). The resultant 16S rDNA sequences were compared to the bacterial sequences recorded in the GenBank database (http://blast.ncbi.nlm.nih.gov/blast.cgi) (BLAST analysis). The similarity rates of the sequences were determined based on this comparison and their GenBank accession numbers were recorded.[Citation15]
Purification of phytase
Ammonium sulphate precipitation
The entire purification process was carried out at +4°C unless otherwise indicated. Following the 7 days-long growth of Weissella halotolerans, phytase obtained from Weissella halotolerans was centrifuged at 24000xg for 30 minutes. The supernatant was taken after centrifugation and gradually precipitated with solid ammonium sulphate at 0–20%, 20–30%, 30–40%, 40–50%, 50–60%, 60–70% and 70–80% saturations, respectively.[Citation16,Citation17] During each precipitation, centrifugation was carried out at 24000xg for 20 minutes. The pellet was dissolved in 50 mM pH 5.5 sodium acetate buffer. The sample obtained with ammonium sulphate precipitation was transferred to a dialysis bag and dialysed against the dialysis buffer (10 mM pH 5.5 sodium acetate).
Q-Sepharose anion exchange chromatography
The enzyme solution obtained after dialysis was administered to the Q-Sepharose anion exchange column that was equilibrated with 50 mM pH 5.5 sodium acetate buffer. Gradient elution was carried out by using 0–1 M NaCI. Column flow rate was adjusted to 15 mL/h and the elutions were transferred to tubes with 3 mL volumes. The activity of each elution was determined at 700 nm. The tubes that showed activity were combined with each other.
Sephadex G-100 gel filtration chromatography
The enzyme solution obtained with Q-Sepharose was administered to the Sephadex G-100 column that was equilibrated with 50 mM pH 5.5 sodium acetate buffer. The Sephadex G-100 gel filtration column was prepared as in our previous studies.[Citation16,Citation18] Then, 1 mL elutes were transferred to tubes. The activity of each elutions was determined at 700 nm.[Citation19]
Determination of the phytase activity
Phytase activity was determined according to the study carried out by Zou et al.[Citation19] The phytase-containing samples (0.1 mL) were incubated at 50°C for 10 minutes after 0.25 mL of 2 mM sodium phytate (dissolved in 100 mmol/l sodium acetate at pH 5.5) was added. Following the hydrolysis, 10% (w/v) trichloroacetic acid (TCA) was added and the reaction was terminated. The inorganic phosphate that was released from sodium phytate was determined by adding ammonium molybdate, sulfuric acid, and ferrous sulphate, calorimetrically. After the 15 minutes reaction, the sample was measured against the blind sample at 700 destaining nm. The phytase unit was defined as the activity that releases 1 μmol of inorganic phosphorus per minute from sodium phytate.
Protein determination
The protein concentration was measured according to the Bradford method by staining with Coomassie Brilliant Blue G-250 and using bovine serum albumin as the standard.[Citation20]
SDS-PAGE analysis
After the enzyme purification, the purity degree of the enzyme was controlled according to the Laemmli’s method by using 3–8% batch sodium dodecyl sulphate polyacrylamide gel electrophoresis (SDS-PAGE).[Citation21] The electrophoresis process was carried out according to our previous articles.[Citation16,Citation22] The gel was stained with the silver staining method and photographed after destaining.
Optimum and stable pH study
In the optimum pH study, enzyme activity was measured by using 100 mM sodium acetate (pH 2.0–6.0), Tris-HCI (pH 7.0–9.0) and sodium carbonate (pH 10.0–12.0) buffer solutions and the pH value at which the enzyme shows the maximum activity was determined. In the stable pH study, the enzyme was kept in the above mentioned buffer solutions and its activity was measured every 12 h for 4 days.[Citation23]
Optimum temperature and temperature stability studies
The temperature was investigated using sodium phytate as the substrate at between 10 and 90°C. To determine the temperature stability, the enzyme was kept at each temperature range for 3 h and measured at 30 minute-intervals.[Citation23]
Effects of metal ions on enzyme activity
The effects of metal ions on phytase were determined by adding Ca2+, Mg2+, Cr2+, Co2+, Cu2+, Zn2+, Ag+, Pb2+, Ni2+, Mn2+, Hg2+, Cd2+ and Fe2+ metal ions at 1 mM and 5 mM final concentrations and measuring enzyme activity. The sample that did not contain metal ions was used as the control sample.
Results
Isolation of Weissella halotolerans strain from fermented food products and its identification
The bacteria were isolated from various sources. Identification studies were carried out after the isolated bacteria were cultured on MRS agar with the streak plate inoculation. DNA isolation was performed for identification. The resultant PCR product was sent to Macrogen Inc. The bacteria were identified according to the 99% sequence similarity (NR_040812.1). Its young culture was obtained and stored as a stock culture. The catalase test and gram staining of the phytase-positive strains were carried out to identify the strains as Weissella halotolerans.
Purification results
As summarised in , the purification of phytase was carried out in simple three steps comprising ammonium sulphate precipitation, Q-Sepharose anion exchange chromatography and Sephadex G-100 gel filtration chromatography. After ammonium sulphate precipitation, approximately 25% of the total protein was removed; after Q-Sepharose, the removal of approximately 77% of the total protein was achieved. The enzyme was purified 11.38-fold with a specific activity of 227.73 EU/mg and 6.52% recovery.
Table 1. Purification steps for phytase from Weissella halotolerans.
shows the elution profiles of Q-Sepharose anion exchange column chromatography and Sephadex G-100 gel filtration chromatography. As seen in , the tubes that showed phytase activity were combined with each other and administered to the Sephadex G-100 column, which was the last step of purification. As seen in , the tubes between 20 and 28 showed the highest phytase activity when compared to the other tubes. The purified enzyme showed a single band. The molecular mass of the enzyme was determined to be 41.52 kDa ().
Figure 1. (a) Elution profile of Q-Sepharose anion exchange chromatography. The Q-Sepharose anion exchange column was equilibrated with 50 mM pH 5.5 sodium acetate buffer. Gradient elution was carried out by using 0–1 M NaCI. (b) Elution profile of Sephadex G-100 gel filtration column chromatography. The Sephadex G-100 gel was equilibrated with 50 mM pH 5.5 sodium acetate buffer. The elutions obtained the Q-Sepharose column was applied to the Sephadex G-100 column for purification.
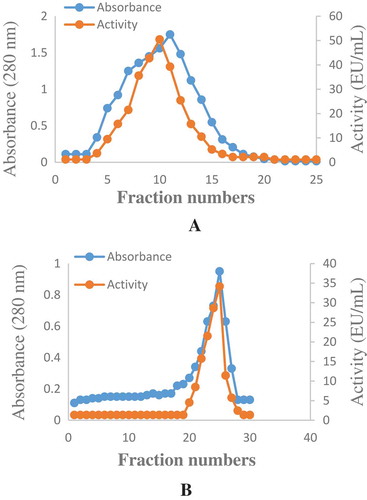
Figure 2. Optimum and pH stability studies were carried out by using 100 mM sodium acetate (pH 2.0–6.0), Tris–HCI (pH 7.0–9.0) and sodium carbonate (pH 10.0–12.0) buffer solutions. a-) Optimum pH profile for the purified phytase b-) pH Stability study for the purified phytase.
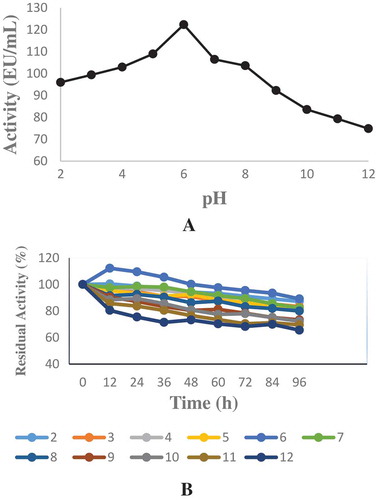
The effect of pH and temperature on the activity and stability of phytase
Weissella halotolerans showed maximum phytase activity at pH 6.0 (). Enzyme activity did not show a significant change between pH 2.0 and 8.0 and maintained its stability (). In this range, it lost approximately 20% of its activity. This result was similar to the result obtained with E. coli ATCC 33965 phytase.[Citation24] This result showed that the purified enzyme was acidic phytase. This property allows the enzyme to show activity in the stomach (pH 2.0–4.5) and is noteworthy in terms of using the enzyme as animal feed. Optimum temperature varies between 33 and 37°C for most phytases.[Citation25] The optimum temperature for phytase purified from Weissella halotolerans was 50°C (). The temperature stability showed that enzyme maintained its activity after being kept at 10-90°C for 3 h (). This result showed that the phytase purified from Weissella halotolerans had thermostability and can be preferred in the food industry.
Effects of metal ions on the phytase activity
The effects of metals on phytase are given in . This results showed that the enzyme activity was inhibited in the presence of Ag+, Zn2+, Cr2+ and Fe2+ by 21.86, 25.63, 32.82 and 90.43%, respectively, whereas it showed an increase in the presence of Co2+, Cu2+, Pb2+, Cd2+ and Mn2+. The enzyme activity showed no significant change at the 1 and 5mM concentrations of Mg2+ and Ca2+ ions. This result revealed that the enzyme maintained its activity in the presence of Mg2+ and Ca2+-phytic acid complexes.
Table 2. The effects of metal ions on phytase purified from Weissella halotolerans.
Discussion
Phytases catalyse the gradual separation of phosphate from phytic acid or the hydrolysis of phytate to degraded inositol phosphate esters and inorganic phosphate.[Citation12] Phytases usually have 40–100 kDa molecular weight and are considered to be monomeric proteins. They have a wide substrate specifity and their optimum pH and temperature are usually between 4.5 and 6.0; 45 and 60°C, respectively.[Citation26] It was reported that phytases could be found in plants, animal tissues and microorganisms. Many researchers found microbial phytase promising for commercial production.[Citation27]
In the study, phytase was simply purified from Weissella halotolerans lactic acid bacteria and the effects of some metals on its activity were investigated. In other studies, phytase was purified from different sources and characterised to determine its biochemical properties and investigate its possible applications in the industry. Phytase was purified at 11.38-fold with a specific activity of 227.73 EU/mg and the molar mass of the enzyme was found 41.52 kDa in the present study.
Similar to our study, Boukhris et al.[Citation28] partially purified phytase from Bacillus amyloliquefaciens US573 at 6-fold with a specific activity of 27 EU/mg and 52% recovery and the molecular mass of the enzyme was 42 kDa. Also, Escobin-Mopera et al.[Citation29] purified phytase from Klebsiella pneumoniae 9-3B at 241-fold and with 1.81% recovery in four steps using ammonium sulphate precipitation and HiPrep SPFF, HiPrep QXL and Superdex 75 columns and the molar mass of the enzyme was 45 kDa. In another study, Parhamfar et al.[Citation30] purified phytase from Geobacillus stearothermophilus strain DM12 at 57.2-fold and with 71.5 EU/mg specific activity by using the same method used in this study and determined the molecular mass of the enzyme to be 28 kDa. The molecular mass of the enzyme purified from Lactobacillus sanfranciscensis CB1 was 50 kDa.[Citation31] Roy et al.[Citation32] determined the molecular mass of the enzyme purified from Shigella sp. CD2 to be 43 kDa. In terms of molar mass, our finding are compatible with the results reported in the literature.
As it is well known, enzyme purification is a difficult process. Enzymes are susceptible to changing conditions; therefore, the presence of enzymes is determined depending on the substrate molecule affected by the enzyme, the product occurring after the reaction or other precursor parameters for the enzymatic reaction. The factors that affect enzyme activity are enzyme concentration, substrate concentration, temperature, pH, allosteric effects, ionic strength and the presence of inhibitors and activators.
Every enzyme has a maximum pH at which its activity is optimum. Above this pH, the three-dimensional structure of the enzyme deteriorates and its activity decreases. In the study, the optimum pH value of the purified phytase was 6.0 and its stable pH value was between 2.0 and 8.0. In the literature, the preceding studies on phytase found different values from our study for optimum pH and stable pH. The maximum activity was found as 5.5 for extracellular L. plantarum phytase [Citation33], whereas L. sanfranciscensis CB1 (intracellular) phytase had maximum activity at pH 4.0.[Citation31] The optimum pH of the intracellular phytase isolated from Lactobacillus fermentum DSM 20052 and Lactobacillus casei 40W was 6.5; the activity of the phytase isolated from Lactobacilllus casei reached its maximum at two different pH values as 5.5 and 7.0; the optimum pH of the phytase isolated from L. plantarum W42 and L. plantarum JBPRS was 6.0–6.5; the optimum pH of the phytase isolated from L. plantarum 110 was 7.5.[Citation34] In et al. reported that the optimum pH of the phytase from Pseudomonas fragi Y9451 was 4.5 and its stable pH was in the 4.0–6.0 range.[Citation35] Kim et al. determined that the pH at which the phytase from Citrobacter braakii YH-15 showed maximum activity was 4.0.[Citation36]
The optimum temperature for phytase activity was determined to be 50°C. In the present study, this result showed a similarity to phytases from Aspergillus japonicus BCC18313 (TR86), A. niger BCC18081(TR170) and E. parvum BCC17694.[Citation37,Citation38] Although the optimum temperature of phytase obtained from Enterobacter was same as the phytase obtained in our study, it showed activity at neutral pH.[Citation27] Seo et al.[Citation39] determined that the optimum activity temperature of the phytase isolated from Aeromonas sp. LIK 1–5 was 50°C and the activity significantly decreased at 60°C. Kim et al.[Citation36] determined that the optimum activity temperature of the phytase isolated from Citrobacter braakii YH-15 was 50°C and the activity significantly decreased at 55°C.
In the temperature stability study for the purified phytase, although the enzyme was subjected to temperatures between 10 and 90°C for 3 h, it lost its activity approximately 25%. Thermostability can be viewed as an important criterion of the usability of phytase in industrial applications. Similar to our finding, in another study, it was determined that phytase derived from L. sanfranciscensis CB1 maintained 70% of its activity even at 70°C.[Citation29]
For catalytic activity, 75% of all of the known enzymes require metal ions. Metal ions immensely affect enzyme activity through various pathways. By donating and receiving electrons, they activate as electrophiles or nucleophiles, can suppress the effect of nucleophiles to prevent undesirable side reactions, can gather enzymes and substrates together through coordinate bonds and simply stabilise the catalytic active conformations of enzymes.[Citation40] The effect of metal ions on phytase depends on microbial species, chain and phytase production capacity.[Citation41]
The investigation of the effects of metal ions on phytase by adding metal ions at 1 and 5 mM final concentrations revealed that the activity of phytase decreased in the presence of Ag+, Zn2+, Cr2+ and Fe2+ ions and increased in the presence of Co2+, Cu2+, Pb2+, Cd2+ ve Mn2+ ions. Yu and Chen [Citation42] investigated the effects of Cu2+, Co2+, Mn2+, Ba2+, Zn2+ ve Ni2+ ions on the phytase purified from Bacillus nealsonii zj0702 and reported that, at their 5 mM concentrations, Co2+, Ba2+, Zn2+, Ni2+ ions strongly inhibited the enzyme.
Roy et al[Citation32] determined that Fe2+, Zn2+ and Cu2+ addition to phytase from Shigella sp. CD2 decreased enzyme activity by 65, 72 and 82%, respectively, whereas Ca2+, Mn2+, Mg2+ and Co2+ increased enzyme activity. The phytase from A. japonicas BCC18313 and A. Niger BCC18081 was inhibited by Zn2+ and Cu2+, [Citation24] whereas the phytase from Selenomonas ruminantium JY35 was inhibited by Zn2+, Cu2+, Fe2+ and Hg2+ metal ions.[Citation43]
Our results showed that Ag+, Zn2+ and Fe2+ ions significantly inhibited the enzyme. Similar results can be found in the related literature. A similar characteristic property was observed in the phytase from Klebsiella oxytoca MO-3.[Citation44] The inhibitory effect of Fe2+, Zn2+ and Ag+ metal ions may have originated from reducing the usability of phytate by binding with the enzyme and forming poorly soluble metal-phytate complexes. In addition, Co2+ metal ion increased enzyme activity. This result is similar to the result obtained with the phytase from Klebsiella pneumonia.[Citation45]
In this study, the phytase purified from Weissella halotolerans was characterised. As a result of the advancing enzyme technology, the diversity of enzyme applications and the considerably high economic value of enzymes, the biotechnology studies on industrial enzymes is gaining further importance. Our findings indicate that the purified phytase can be used in the industry and its economic value can be utilised thanks to its characteristic properties.
Acknowledgements
The authors report no conflicts of interest.
References
- Vohra, A.; Satyanarayana, T. Phytases: Microbial Sources, Production, Purification and Potential Biotechnological Applications. Critical Reviews in Biotechnology 2003, 23 (1), 29–60. doi:10.1080/713609297
- Singh, B.; Kunze, G.; Satyanarayana, T. Developments in Biochemical Aspects Andbiotechnological Applications of Microbial Phytases. Biotechnology and Molecular Biology Reviews 2011, 6 (3), 69–87.
- Singh, N. K.; Joshi, D. K.; Gupta, R. K. Isolation of Phytase Producing Bacteria Andoptimization of Phytase Production Parameters. Jundishapur Journal of Microbiology 2013, 6, 6419. doi:10.5812/jjm.6419
- Lei, X. G.; Porres, J. M. Phytase Enzymology. Applications, and Biotechnology, Biotechnology Letters 2003, 25, 1787−1794.
- Greiner, R.; Farouk, A.; Carlsson, N.; Konietzny, U. Myo-Inositolphosphate Isomers Generated by the Action of a Phytase from a Malaysian Wastewater Bacterium. The Protein Journal 2007, 26, 577−584. doi:10.1007/s10930-007-9100-5
- Haros, M.; Bielecka, M.; Honke, J.; Sanz, Y. Myo-Inositolhexakisphosphate Degradation by Bifidobacterium Infantis ATCC15697. International Journal of Food Microbiology 2007, 117, 76−84. doi:10.1016/j.ijfoodmicro.2007.02.021
- Yi, Z.; Kornegay, E. T.; Ravindran, V.; Denbow, D. M. Improving Phytate Phosphorus Availability in Corn and Soybean Meal for Broilers Using Microbial Phytase and Calculation of Phosphorus Equivalency Values for Phytase. Poultry Science 1996, 75 (2), 240–249. doi:10.3382/ps.0750240
- Da Silva, L. G.; Trugo, L. C.; Da Costa, T. S.; Couri, S. Low Phytate Lupin Flour Based Biomass Obtained by Fermentation with a Mutant of Aspergillus Niger. Process Biochemistry 2005, 40 (2), 951–954. doi:10.1016/j.procbio.2004.02.016
- Vohra, A.; Satyanarayana, T. Phytase Production by the Yeast, Pichiaanomala. Biotechnology Letters 2001, 23 (7), 551–554. doi:10.1023/A:1010314114053
- Vohra, A.; Rastogi, S. K.; Satyanarayana, T. Amelioration in Growth and Phosphorus Assimilation of Poultry Birds Using Cell-Bound Phytase of Pichia Anomala. World Journal of Microbiology and Biotechnology 2006, 22 (6), 553–558. doi:10.1007/s11274-005-9070-8
- Yoo, G. Y.; Wang, X. J.; Choi, S. Y.; Han, K.; Kang, J. C.; Bai, S. C. Dietary Microbial Phytase Increased the Phosphorus Digestibility in Juvenile Korean Rock Fish Sebastes Schlegeli Fed Diets Containing Soybean Meal. Aquaculture 2005, 243, 315–322. doi:10.1016/j.aquaculture.2004.10.025
- Konietzny, U.; Greiner, R. Bacterial Phytase: Potential Application, in Vivo Function and Regulation of Its Synthesis. Brazilian Journal of Microbiology 2004, 35, 11–18. doi:10.1590/S1517-83822004000100002
- Bischoff, K. M.; Rooney, A. P.; Li, X. L.; Liu, S.; Hughes, R. Purification and Characterization of a Family 5 Endoglucanase from a Moderately Thermophilic Strain of Bacillus Licheniformis. Biotechnology Letters 2006, 28, 1761–1765. doi:10.1007/s10529-006-9153-0
- Adiguzel, A.; Ozkan, H.; Baris, O.; Inan, K.; Gulluce, M.; Sahin, F. Identification and Characterization of Thermophilic Bacteria Isolated from Hot Springs in Turkey. Journal of Microbiological Methods 2009, 79, 321–328. doi:10.1016/j.mimet.2009.09.026
- Altschul, S. F.; Gish, W.; Miller, W.; Myers, E. W.; Lipman, D. J. Basic Local Alignment Search Tool. Journal of Molecular Biology 1990, 215, 403–410. doi:10.1016/S0022-2836(05)80360-2
- Beydemir, Ş.; Demir, Y. Antiepileptic Drugs: Impacts on Human Serum Paraoxonase-1. Journal of Biochemical and Molecular Toxicology 2016,e21889. doi:10.1002/jbt.21889
- Kırıcı, M.; Kırıcı, M.; Demir, Y.; Beydemir, Ş.; Atamanalp, M. The Effect of Al3+ and Hg2+ on Glucose 6-Phosphate Dehydrogenase from Capoetaumbla Kidney. Applied Ecology and Environmental Research 2016, 14 (2), 253–264. doi:10.15666/aeer/1402_253264
- Alim, Z.; Beydemir, Ş. Some Anticancer Agents Act on Human Serum Paraoxonase-1 to Reduce Its Activity. Chemical Biology & Drug Design 2016, 88 (2), 188–196. doi:10.1111/cbdd.12746
- Zou, L. K.; Wang, H. N.; Pan, X.; Tian, G. B.; Xie, Z. W.; Wu, Q.; Chen, H.; Yang, Z. R. Expression, Purification and Characterization of a phyAm−phyCs Fusion Phytase. Journal of Zhejiang University Science B 2008, 9, 536−545. doi:10.1631/jzus.B0720006
- Bradford, M. M. A.;. Rapid and Sensitive Method for the Quantitation of Microgram Quantities of Protein Utilizing the Principle of Protein-Dye Binding. Analytical Biochemistry 1976, 72, 248–251. doi:10.1016/0003-2697(76)90527-3
- Laemmli, D. K.;. Cleavage of Structural Proteins during the Assembly of the Head of Bacteriophage T4. Nature 1970, 227, 680–683. doi:10.1038/227680a0
- Demir, Y.; Beydemir, Ş. Purification, Refolding, and Characterization of Recombinant Human Paraoxonase-1. Turkish Journal of Chemistry 2015, 39 (4), 764–776. doi:10.3906/kim-1501-51
- Sarıbuga, E.; Nadaroglu, H.; Dikbas, N.; Senol, M.; Cetin., B. Purification, Characterization of Phytase Enzyme from Lactobacillus Plantarum Bacteria and Determination of Its Kinetic Properties. African Journal of Biotechnology 2014, 13 (23), 2373–2378. doi:10.5897/AJB2014.13821
- Golovan, S.; Wang, G.; Zhang, J.; Forsberg, C.; Characterization and over Production of the Escherichia coli 365 appA Encoded Bifunctional Enzyme that Exhibits Both Phytase and Acid Phosphatase Activity. Canadian Journal of Microbiology 2000, 46, 59–71. doi:10.1139/cjm-46-1-59
- Hara, A.; Ebina, S.; Kondo, A.; Funaguma, T.; A new type of phytase from pollen of Typha latifolia L. Agricultural and Biological Chemistry 1985, 49, 3539–3544.
- Pandey, A.; Szakacs, G.; Soccol, C. R.; Rodriguez-Leon, J. A.; Soccol, V. T. Production, Purification and Properties of Microbial Phytases. Bioresource Technology 2001, 7, 203–214. doi:10.1016/S0960-8524(00)00139-5
- Yao, M. Z.; Zhang, Y. H.; Lu, W. L.; Hu, M. Q.; Wang, W.; Liang, A. H. Phytases: Crystal Structures, Protein Engineering and Potential Biotechnological Applications. Journal of Applied Microbiology 2012, 112 (1), 1–14. doi:10.1111/j.1365-2672.2011.05181.x
- Boukhris, I.; Farhat-Khemakhem, A.; Blibech, M.; Bouchaala, K.; Chouayekh, H. Characterization of an Extremely Salt-Tolerant and Thermostable Phytase from Bacillus Amyloliquefaciens US573. International Journal of Biological Macromolecules 2015, 80, 581–587. doi:10.1016/j.ijbiomac.2015.07.014
- Escobin-Mopera, L.; Ohtani, M.; Sekiguchi, S.; Sone, T.; Abe, A.; Tanaka, M.; Meevootisom, V.; Asano, K. Purification and Characterization of Phytase from Klebsiella Pneumoniae 9-3B. Journal of Bioscience and Bioengineering 2012, 113 (5), 562–567. doi:10.1016/j.jbiosc.2011.12.010
- Parhamfar, M.; Badoei-Dalfard, A.; Khaleghi, M.; Hassanshahian, M. Purification and Characterization of an Acidic, Thermophilic Phytase from a Newly Isolated Geobacillus Stearothermophilus Strain DM12. Program in Biological Sciences 2015, 5 (1), 61–73.
- De Angelis, M.; Gallo, G.; Corbo, M. R.; McSweeney, P. L.; Faccia, M.; Giovine, M.; Gobbetti, M. Phytase Activity in Sourdough Lactic Acid Bacteria: Purification and Characterization of a Phytase from Lactobacillus Sanfranciscensis CB1. International Journal of Food Microbiology 2003, 87 (3), 259–270. doi:10.1016/S0168-1605(03)00072-2
- Roy, M. P.; Poddar, M.; Singh, K. K.; Ghosh, S. Purification, Characterization and Properties of Phytase from Shigella Sp. CD2. Indian Journal of Biochemistry & Biophysics 2012, 49 (4), 266–271.
- Zamudio, M.; Gonzales, A.; Medina, J. A. Lactobacillus Plantarum Phytase Activity Is Due to Non-Specific Acid Phosphatase. Letters in Applied Microbiology 2001, 32, 181–184. doi:10.1046/j.1472-765x.2001.00890.x
- Haros, M.; Bielecka, M.; Honke, J.;, et al. Phytate-Degrading Activity in Lactic Acid Bacteria. Polish Journal Of Food And Nutrition Sciences 2008, 58, 33–40.
- In, M. J.; Jang, E. S.; Kim, Y. J.; Oh, N. S. Purification and Properties of an Extracellular Acid Phytase from Pseudomonas Fragi Y9451. Journal of Microbiology and Biotechnology 2004, 14 (5), 1004–1008.
- Kim, H. W.; Kim, Y. O.; Lee, J. H.; Kim, K. K.; Kim, Y. J. Isolation and Characterization of a Phytase with Improved Properties from Citrobacter Braakii. Biotechnology Letters 2003, 15, 1231–1234. doi:10.1023/A:1025020309596
- Fugthong, A.; Boonyapakron, K.; Sornlek, W.; Tanapongpipat, S.; Eurwilaichitr, L.; Pootanakit, K. Biochemical Characterization and in Vitro Digestibility Assay of Eupenicillium Parvum (BCC17694) Phytase Expressed in Pichia Pastoris. Protein Expression and Purification 2010, 70, 60−67. doi:10.1016/j.pep.2009.10.001
- Promdonkoy, P.; Tang, K.; Sornlake, W.; Harnpicharnchai, P.; Kobayashi, R. S.; Ruanglek, V.; Upathanpreecha, T.; Vesaratchavest, M.; Eurwilaichitr, L.; Tanapongpipat, S. Expression and Characterization of Aspergillus Thermostable Phytases in Pichia Pastoris. FEMS Microbiology Letters 2009, 290, 18−24. doi:10.1111/j.1574-6968.2008.01399.x
- Seo, M. J.; Kim, J. N.; Cho, E. A.; Park, H.; Choi, H. J.; Pyun, Y. R. Purification and Characterization of a Novel Extracellular Alkaline Phytase from Aeromonas Sp. Journal of Microbiology and Biotechnology 2005, 15 (4), 745–748.
- Bajaj, B. K.; Manhas, K. Production and Characterization of Xylanase from Bacillus Licheniformis P11(C) with Potential for Fruit Juice and Bakery Industry. Biocatalysis and Agricultural Biotechnology 2012, 1 (4), 330–337. doi:10.1016/j.bcab.2012.07.003
- Hayek, S. A.; Shahbazi, A.; Worku, M.; Ibrahim, S. A. Enzymatic Activity of Lactobacillus reuteri Grown in a Sweet Potato Based Medium with the Addition of Metal Ions. Springer Plus 2013, 2, 465. doi:10.1186/2193-1801-2-465
- Yu, P.; Chen, Y. Purification and Characterization of a Novel Neutral and Heat-Tolerant Phytase from a Newly Isolated Strain Bacillus Nealsonii ZJ0702. BMC Biotechnology 2013, 13 (78), 1–7. doi:10.1186/1472-6750-13-78
- Yanke, L. J.; Selinger, L.; Cheng, K. Phytase Activity of Selenomonas Ruminantium: A Preliminary Characterization. Letters in Applied Microbiology 1999, 29, 20−25. doi:10.1046/j.1365-2672.1999.00568.x
- Jareonkitmongol, S.; Ohya, M.; Watanabe, R.; Takagi, H.; Nakamori, S. Partial Purification of Phytase from Soil Isolate Bacterium Klebsiella Oxytoca MO-3. Journal of Fermentation and Bioengineering 1997, 83, 393–394. doi:10.1016/S0922-338X(97)80149-3
- Wang, X.; Upatham, S.; Panbangred, W.; Isarangul, D.; Summpunn, P.; Wiyakrutta, S.; Meevootisom, V. Purification, Characterization, Gene Cloning and Sequencing Analysis of a Phytase from Klebsiella pneumoniae Subsp. Pneumoniae XY-5. Science Asia 2004, 30, 383–390. doi:10.2306/scienceasia1513-1874.2004.30.383