ABSTRACT
The present study determined the effects of cross-linking with sodium trimetaphosphate (STMP)/sodium tripolyphosphate (STPP) on the structural, rheaological, and pasting properties of potato starch. Starches cross-linked by STMP/STPP at 0.0125%, 0.025%, 0.05%, 0.5%, 5%, and 10% were designated as the cross-linking of potato starch (CLPS)-0.0125, CLPS-0.025, CLPS-0.05, CLPS-0.5, CLPS-5, and CLPS-10, respectively. Scanning electron microscope revealed that STMP/STPP treatment resulted in some changes in the surface of the native potato starch (NPS) granules. An analysis with a light microscope showed that NPS granules were completely ruptured and had disappeared but that the granules of CLPS-0.0125, CLPS-0.025, and CLPS-0.05 were scarcely ruptured after heating at 95°C for 30 min. The 31P nuclear magnetic resonance spectra of CLPS-5 and CLPS-10 showed new peaks of monostarch diphosphate, cyclic-monostarch monophosphate, and distarch monophosphate. Pastes of CLPS-0.0125, CLPS-0.025, and CLPS-0.05 exhibited significantly higher shear-thinning fluid characteristics than NPS. Steady and dynamic shear analysis showed that, compared to NPS, CLPS pastes (0.5, 5, and 10) had significantly greater apparent viscosity (ηa,100), consistency index (K), yield stress (σoc), dynamic moduli (G′, G″), and complex viscosity (η*). These results indicate that cross-linking with STMP/STPP can be used to improve the rheaological and pasting properties of potato starch for desired food applications.
Introduction
Potato starch is the third most important material used in food and nonfood industries, and it comprises an estimated 6% share of the global starch production. Potato starch has functional properties including thickening, coating, gelling, adhesion, and encapsulation.[Citation1,Citation2] However, unmodified potato starch has limited use in the food processing industry due to its physical and chemical properties, including inadequate shear and acid or thermal decomposition. Thus, potato starch requires physical and/or chemical modifications to improve its properties.
In the food industry, starch is commonly cross-linked to solve such problems. Cross-linking reinforces the already present hydrogen bonds in granules with new covalent bonds. As a result, cross-linked starch is more resistant to acid, heat, and shearing than natural starch. Therefore, it is more suitable for applications such as canned food, hot chilled food, and salad dressing.[Citation3,Citation4] The most widely used cross-linking reagents include phosphorus chloride (POCl3), sodium trimetaphosphate (STMP), sodium tripolyphosphate (STPP), epichlorohydrin (EPI), and a mixture of adipic acid and acetic anhydride that can form ether or ester linkages with hydroxyl groups within starch granules.[Citation5]
Chemical modification can lead to considerable changes in the rheaological properties of the final products because a small change in the molecular conformation and structure of the starch can result in dramatic changes in the rheaological properties.[Citation6] The viscosity of chemically modified starch paste can increase or decrease when a suitable chemical modification is made.[Citation7] Therefore, the rheaological properties of the cross-linked starches should be determined to understand their suitability for a given food product.[Citation8,Citation9]
Although many studies have been conducted on starch cross-linking, most of the earlier studies on starch modification with STMP/STPP were conducted using corn starch, wheat starch, and tapioca starch.[Citation10–Citation16] According to Wongsagonsup, the rheaological properties of tapioca starch cross-linked with STMP/STPP can maintain a higher viscosity and show a low change in viscosity. To the best of our knowledge, little information is available on the rheaological, pasting, and structural properties of potato starch cross-linked via STMP/STPP. Therefore, the objective of the present study was to prepare cross-linked potato starch (CLPS) with different concentrations of STMP/STPP and to determine the effects of cross-linking on the physicochemical, structural, rheaological, and pasting properties of CLPS.
Materials and methods
Materials
Potato (Goun) was obtained from the National Institute of Highland, Pyeongchang, Korea. STMP, STPP, and porcine pancreatin (P-7545, activity 8 × USP g−1) were obtained from Sigma-Aldrich Chemical Co. (St. Louis, MO, USA). Amyloglucosidase (300L, 300 AGU mL−1) was purchased from Novozymes (Basvaerd, Denmark). A total starch assay kit was obtained from Megazyme International Ireland Ltd. (Bray, Ireland). All other chemical reagents were of analytical grade.
Isolation of potato starch and preparation of CLPS
The potato starch examined in this study was isolated using sodium bisulfite.[Citation17] CLPS was prepared according to a method by Woo and Seib[Citation18] with some modifications. Briefly, native potato starch (NPS) (100 g, db), sodium sulfate (10 g), and different amounts (0.0125, 0.025, 0.05, 0.5, 5, and 10 g) of mixture of STMP and STPP (99:1) were mixed with distilled water (140 mL). After the pH was adjusted to 11.0 with 1 N NaOH, the slurry was kept at 45°C for 3 h using a magnetic stirrer equipped with a temperature controller (PS-1000, EYELA, Tokyo, Japan). The suspension was neutralized with 1 N HCl to pH 6.5 to stop the reaction and centrifuged with distilled water for five times. After drying at 45°C for 48 h, dried samples were ground and passed through a 100-mesh sieve. Based on different amounts of STMP/STPP, samples cross-linked by STMP/STPP at 0.0125%, 0.025%, 0.05%, 0.5%, 5%, and 10% (w/v) were designated as CLPS-0.0125, CLPS-0.025, CLPS-0.05, CLPS-0.5, CLPS-5, and CLPS-10, respectively.
Chemical composition analysis
The chemical compositions of NPS including moisture, crude ash, crude protein, and crude lipid content were determined according to American Association of Cereal Chemists (AACC) Approved Methods 08-01, 30-10, 44-15A, and 46-13, respectively. Total starch content in NPS was determined using the AACC method 76-13.01.[Citation19] The amylose content was determined with a procedure described by Williams et al.[Citation20]
Determination of phosphorus content and degree of substitution
The phosphorus content of the NPS and CLPS samples was determined using inductively coupled plasma inductively coupled plasma optical emission spectrometry (ICP-OES) after hot nitric acid digestion. Starch samples (0.3 g) were digested with 10 mL 60% (w/v) nitric acid at 180°C for 2 h. After cooling, they were diluted with 30 mL of distilled water and analyzed with an Agilent 5100 ICP-OES (Agilent Technologies, Mulgrave, Australia). The degree of substitution (DS) was calculated as follows[Citation21]:
where P is the phosphorus content (%).
Swelling factor
The swelling factor (SF) of the NPS and CLPS samples was determined using the procedure proposed by Tester and Morrison[Citation22] with some modifications. The sample (100 mg) was combined with 5 mL of distilled water and incubated in a water bath at 70°C for 30 min. The mixture was vortexed thoroughly for 5 min. After cooling the sample in an ice bath, 0.5 mL of blue dextran solution (5 mg/mL) was added. The solution was then centrifuged at 3000 × g for 15 min, and the absorbance of the supernatant was measured at 620 nm. The SF was calculated using the following equation:
where w is the sample weight (mg), AS is the absorbance of the supernatant, and AR is the absorbance of the reference (without starch).
Scanning electron microscopy
The morphological features of the NPS and CLPS samples were observed using a scanning electron microscope (SEM, S-4700, Hitachi Co., Tokyo, Japan). Each sample was fixed on a specimen holder with the help of double-sided carbon tape. It was then sputtered with platinum powder using an ion sputter coater. Representative micrographs were taken for each sample at a magnification of 1000×.
Light microscopy
One percent (w/w) of dispersions of NPS and CLPS samples were prepared in distilled water. These dispersions were stirred at room temperature for 30 min and heated in a magnetic stirrer equipped with a temperature controller (PS-1000, EYELA, Tokyo, Japan) at 95°C for 30 min. Hot samples of NPS and CLPS were stained with Lugol’s solution (1 g I2, 2 g KI/300 mL H2O) diluted with distilled water to 50%. The samples were observed immediately after staining using a light microscope (ECLIPSE Ci-S, Nikon Co., Tokyo, Japan) at a magnification of 20×.
31P nuclear magnetic resonance spectroscopy
NPS and CLPS were converted to phosphodextrins and analyzed via 31P nuclear magnetic resonance (31P NMR) based on the method by Sang et al.[Citation23] Briefly, freeze-dried starch digest (1.0 g) was dissolved in deuterium oxide (1.0 mL) containing 20 mM ethylenediaminetetraacetic acid (EDTA) and 0.002% (w/v) sodium azide. The pH was adjusted to 8.0 by adding 0.1 M sodium hydroxide. 31P NMR data were recorded on a JMM-ECZ 500R spectrometer (JEOL Co., Tokyo, Japan). NMR data were processed with JEOL DELTA program version 5.0.4. 31P NMR experiments were performed at 25°C using a delay of 6 s between pulses with pulse width of 15.0 μs and sweep width of 500 MHz.
Pasting properties
Pasting properties of NPS and CLPS samples (CLPS-0.0125, CLPS-0.025, and CLPS-0.05) were analyzed using a Rapid Visco Analyzer (RVA4, Newport Scientific Inc., Warriewood, Australia) with a Standard 2 profile. The samples (5% db, 25 g total weight) were equilibrated at 50°C for 1 min, heated at 6°C min−1 to 95°C, held at 95°C for 5 min, cooled at 50°C at 6°C min−1, and held at 50°C for 2 min. The paddle speed was set at 160 rpm for the experiment.
Rheaological properties
Preparation of starch pastes for rheaological measurement
NPS and CLPS dispersions (5%, w/w) at different STMP/STPP concentrations (0.0125, 0.025, and 0.05) were prepared by mixing starch with distilled water. Starch dispersions were moderately stirred at room temperature for 30 min and then heated in a 95°C water bath for 30 min with constant mild agitation provided by a magnetic stirrer in order to avoid sedimentation and agglomeration. At the end of the heating period, hot pastes were immediately transferred to a rheometer plate to measure their rheaological properties.
Steady shear rheaological properties
Steady shear rheaological properties of the starch samples were determined using a rheometer (MCR-102, Anton paar, Graz, Austria) with a plate-to-plate system (diameter: 5 cm, gap: 0.5 mm). The samples were transferred to the rheometer plate at 25°C. Steady shear data were obtained over the shear rate in the range of 0.1–500 s−1. To describe the steady shear rheaological properties of samples, data were fitted to the well-known power law (Eq. 3) and Casson (Eq. 4) models:
where σ was the shear stress (Pa), was the shear rate (s−1), K was the consistency index (Pa sn), n was the flow behavior index (dimensionless), and (Kc)2 was the Casson plastic viscosity (ηc). Casson yield stress (σoc) according to the Casson model (Eq. 4) was determined as square of the intercept (Koc) obtained from the linear regression of the square roots of the shear rate–shear stress data. Using the magnitudes of K and n, the apparent viscosity (ηa,100) at 100 s−1 was calculated.[Citation24]
Dynamic shear rheaological properties
Dynamic shear rheaological properties of the starch samples were determined using a rheometer (MCR-102, Anton paar, Graz, Australia) with a plate-to-plate system (diameter: 5 cm, gap: 0.5 mm). Dynamic shear data were obtained from frequency sweeps over the range of 0.63–62.8 rad/s at 2% strain. The 2% strain was in the linear viscoelastic region. The dynamic shear rheaological properties [storage modulus (G′), loss modulus (G″), complex viscosity (η*), and tan δ (G″/G′)] of the starch samples were then determined.
Statistical analysis
All statistical analyses were performed using SAS version 9.1 (SAS Institute Inc., Cary, NC, USA). ANOVA was performed using the general linear models procedure to determine significant differences among the samples. The means were compared using Fisher’s least significant difference procedure. Significance was defined at the 5% level (p < 0.05).
Results and discussion
Chemical analysis
The potato starch contained 9.47% moisture, 0.20% crude ash, 0.50% crude protein, 0.31% crude fat, and 86.80% total starch (data not shown). The results of very low contents of protein and fat indicated that the obtained potato starch was very pure and that the residual protein had been thoroughly removed during isolation. The isolated potato starch contained 32.52% amylose and 67.48% amylopectin (data not shown).
Phosphorus content, DS, and SF
Potato starch generally contains 0.01–0.6% phosphorus content which can affect the physicochemical properties of starch.[Citation1] In the present study, the phosphorus contents of CLPS 0.0125 and CLPS 10 were 0.0531–0.3421%, respectively (), which were under the regulation limit.[Citation25] The DS was calculated by the phosphorus content of CLPS, as mentioned in Eq. (1). With an increase in the concentration of STMP/STPP, the DSs for the CLPS samples increased from 5.561 to 36.136 ().
Table 1. Phosphorus content, degree of substitution (DS) and swelling factor of native (NPS), and cross-linked potato starch (CLPS) samples prepared with different concentrations of STMP/STPP.
All CLPS samples had significantly (p < 0.05) lower SFs in comparison to NPS (). A reduced SF was observed in CLPS with an increase in the concentration of cross-linking reagents, consistent with results of cross-linked cassava starch[Citation26], waxy maize starch[Citation27], and taro starch.[Citation28] Cross-linking with STMP/STPP can reinforce starch granules with new covalent bonds as a bridge between starch molecules, consequently leading to less swelling than native granules.[Citation3]
SEM
SEM micrographs of NPS and CLPS prepared with different STMP/STPP concentrations at a 1000× magnification are shown in . SEM revealed that STMP/STPP treatments resulted in some changes in the surface of NPS granules. NPS granules had a smooth and rounded shape without any damage or fissures. In contrast, the CLPS granules exhibited slightly rough surfaces and cavities. The number of cavities increased, and small protuberances were found on the surface particularly for CLPS-0.5, CLPS-5, and CLPS-10. Such changes were not present in NPS.
Light microscopy
The micrographs of NPS and CLPS granules heated at 95°C for 30 min are shown in . After heating, NPS granules were completely ruptured and had disappeared. On the other hand, granules of CLPS-0.0125, CLPS-0.025, and CLPS-0.05 were scarcely ruptured. They remained as intact swollen granules, indicating their resistance to high temperature stress. These results were consistent with those of Morikawa and Nishinari,[Citation29] who showed that hydroxypropylated phosphate CLPS samples remained intact as swollen granules after heating at 100°C for 40 min. Cooreman et al.[Citation30] also reported that cross-linked corn starch in the DMSO–water mixture maintained its granules in the range of 25–75°C without dissolution. However, the granules of CLPS-0.5, CLPS-5, and CLPS-10 had dark and shell shapes, leaving ungelatinized granules intact due to excessive cross-linking.[Citation16]
31P NMR spectroscopy
31P NMR spectroscopy was used to demonstrate the location of phosphorylation in native and cross-linked starches. In general, starch phosphates present in different forms can lead to different chemical shifts in the 31P NMR spectrum.[Citation31] The 31P NMR spectra of the NPS and CLPS samples are shown in . All samples exhibited three strong peaks for monostarch monophosphate (MSMP, St-P) at 3.8–4.9 ppm. Specifically, signals at 4.6, 4.3, and 3.9 ppm indicated phosphorylation of the hydroxyl groups at C-2, C-3, and C-6, respectively.[Citation12,Citation31]
Figure 2. 31P-NMR spectra of native (a) and cross-linked potato starch (CLPS) prepared with 5% STMP/STPP concentration (b).
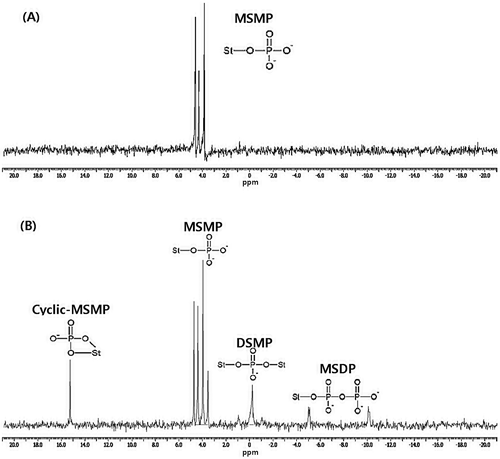
Interestingly, only CLPS-5 and CLPS-10 had four types of phosphate esters: monostarch diphosphate (P–St–P), cyclic-monostarch monophosphate (P > St), and distarch monophosphate (DSMP, St–P–St), which had a signal centered at −5.0 and −10.0, 15.4, and −0.1 ppm, respectively.[Citation12,Citation32] CLPS at 0.0125–0.5% did not have new peaks, probably due to considerably lower STMP/STPP concentrations. These results confirmed cross-linking (DSMP, St–P–St) within the starch granules when the cross-linking reaction was carried out with STMP/STPP.
Pasting properties
The pasting parameters of NPS and CLPS are shown in . The samples of CLPS-0.5, CLPS-5, and CLPS-10 showed no peaks in RVA viscosity patterns due to higher level of cross-linking which consequently inhibited the swelling and/or gelatinization from starch granules. For CLPS-0.0125, CLPS-0.025, and CLPS-0.05, the pasting temperatures were significantly increased compared to that for NPS. Cross-linking, interbonding, and intra-bonding between starches can make starch granules more ordered. Consequently, higher energy would be required to swell.[Citation26]
Table 2. Pasting properties of native (NPS) and cross-linked potato starches (CLPS) prepared with different STMP/STPP concentrations.
CLPS-0.0125, CLPS-0.025, and CLPS-0.05 samples showed significantly higher peak viscosity and final viscosity compared to NPS. Such higher pasting properties for these CLPS samples could be related to the intact swollen granules already shown in microscopic observations (). Cross-linking can enhance heat and shear tolerance against breakage and disintegration of starch granules due to new covalent bonds. Consequently, more energy is required to break these bonds.[Citation16] Thus, CLPS exhibited higher viscosities than NPS during heating and cooling due to new strong covalent bonds between starch granules that occurred through the cross-linking treatments. Similar results have been reported by Hazarika and Sit[Citation28] after cross-linking taro starch and by Jyothi et al.[Citation26] for cross-linking of cassava starch.
The breakdown viscosities of CLPS-0.0125, CLPS-0.025, and CLPS-0.05 (64–657 cP) were significantly lower than that of NPS (2061 cP). After reaching peak viscosity, these CLPS samples maintained higher viscosity than NPS. This finding suggested that cross-linking could strengthen the swollen granules against breakage under a high shear and temperature, thus minimizing or preventing loss in the viscosity. These results indicated that CLPS-0.0125, CLPS-0.025, and CLPS-0.05 could be used as thickeners to withstand severe processing conditions in the food industry.
Steady shear rheaological properties
As already mentioned earlier in microscopic observations () and pasting properties (), CLPS-0.5, CLPS-5, and CLPS-10 were not gelatinized due to higher degrees of cross-linking. Thus, they were not used to investigate the steady shear rheaological or dynamic shear rheaological properties. The shear stress versus shear rate data for NPS and CLPS (-0.125, -0.025, and -0.05) pastes at 25°C exhibited non-Newtonian flow behavior with yield stress (). They were well fitted to the power law (Eq. 1) and Casson (Eq. 2) models (). The n values of NPS and CLPS (-0.125, -0.025, and -0.05) pastes were obtained in the range of 0.48–0.63, suggesting that all samples exhibited shear-thinning behavior. The n values of these CLPS pastes were significantly lower than that of NPS, indicating that these CLPS pastes had higher shear-thinning behaviors. The higher shear thinning behavior of CLPS may be due to the lower level of disruption of the intramolecular and intermolecular bonding system.[Citation33] Zięba et al.[Citation34] observed similar results. They found that the n value of the potato starch cross-linked by adipic acid (0.61) was lower than that of native starch (0.81). According to Wongsagonsup et al.[Citation16], the n value of native tapioca starch paste was remarkably reduced after modification of starch with STMP/STPP.
Figure 3. Shear stress versus shear rate plots of native (NPS) and cross-linked potato starch (CLPS) pastes prepared with different STMP/STPP concentrations at 25°C: (○) NPS, (×) CLPS-0.0125, (◇) CLPS-0.025, and (△) CLPS-0.05.
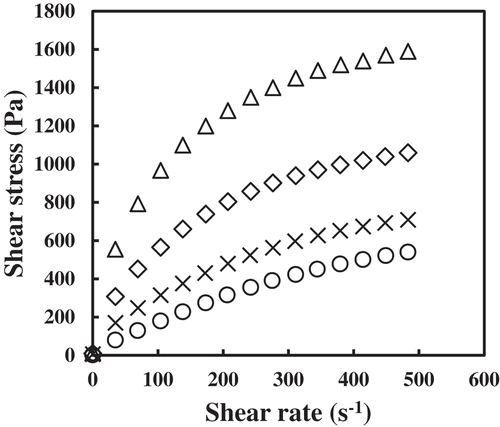
The values of the apparent viscosity (ηa,100), consistency index (K), and Casson yield stress (σoc) of NPS and CLPS (-0.125, -0.025, and -0.05) pastes are summarized in . Compared to NPS, the CLPS pastes showed significantly greater values of ηa,100, K, and σoc. The K values of CLPS pastes (27.12–93.40 Pa sn) were 2–9 times higher than that of NPS (10.76 Pa sn). The σoc values of the CLPS pastes (84.90–430.62 Pa) were also 5–25 times higher than that for NPS (17.81 Pa). Similar trends for ηa, K, and σoc values were observed for other modified starches, including octenylsuccinate corn starch[Citation8], acetylated rice starch[Citation35], acetylated corn starch, acetylated cross-linked corn starch, and hydroxypropylated cross-linked corn starch.[Citation36] According to Wlodarczyk-Stasiak,[Citation37] the cross-linking reaction can limit the loss of amylose released from starch and stabilize the new formed starch, consequently leading to an increase in viscosity. These findings obtained from steady shear rheaological properties showed that CLPS pastes (CLPS-0.125, -0.025, and -0.05) had highly shear-thinning fluids with high magnitudes of yield stresses. Their steady shear properties were apparently improved with increasing concentrations (0.0125–0.05%) of STMP/STPP.
Table 3. Apparent viscosity (ηa,100), consistency index (K), flow behavior index (n), and Casson yield stress (σoc) of native (NPS), and cross-linked potato starch (CLPS) pastes prepared with different STMP/STPP concentrations at 25°C.
Dynamic shear rheaological properties
The changes in storage modulus (G′), loss modulus (G″), complex viscosity (η*), and tan δ as a function of the frequency (ω) for NPS and CLPS (-0.125, -0.025, and -0.05) pastes are shown in . The G′ and G″ values of the CLPS pastes were much higher than those of NPS. The G′ values were greater than the G″ values at all frequency ranges (0.63–62.8 rad s−1). The higher G′ values for the CLPS pastes might be due to the formation of a strong gel network.
Figure 4. Plots of log G′, G″, η*, and tan δ versus log ω of native (NPS) and cross-linked potato starch (CLPS) pastes prepared with different STMP/STPP concentrations at 25°C: (○) NPS, (×) CLPS-0.0125, (◇) CLPS-0.025, and (△) CLPS-0.05.
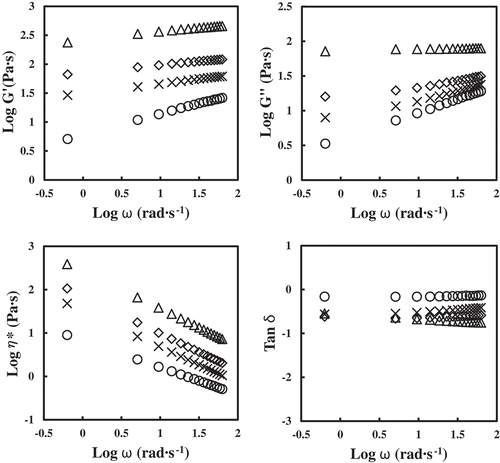
The frequency dependence of the complex viscosity (η*) for NPS and CLPS (-0.125, -0.025, and -0.05) pastes is illustrated in . All starch samples exhibited shear-thinning flow behavior in accordance with the power law model. Moreover, the complex viscosity of all CLPS pastes was significantly (p < 0.05) higher than that of NPS, and this was confirmed by the steady shear rheaological data ().
The G′, G″, η*, and tan δ at 6.28 rad s−1 for NPS and CLPS (-0.125, -0.025, and -0.05) pastes are shown in . The dynamic moduli (G′ G″, and η*) values for the CLPS pastes were significantly greater than those for NPS, and they were significantly elevated with increases in the STMP/STPP concentrations. Consistent with our results, CLPSs prepared with POCl3 up to 500 ppm concentration have been reported to have considerable increases in the dynamic values compared to native ones.[Citation38,Citation39]
Table 4. Storage modulus (G′), loss modulus (G″), complex viscosity (η*), and tan δ at 6.28 rad/s of native (NPS) and cross-linked potato starch (CLPS) pastes prepared with different STMP/STPP concentrations at 25°C.
In semisolid food, the tan δ (ratio of G″/G′) values can be used to elucidate the viscoelastic behavior of starch pastes.[Citation40] For example, the tan δ values <1 indicate predominantly elastic behavior while those >1 indicate predominantly viscous behavior. As shown in , the tan δ values at a frequency of 6.28 rad/s for the NPS and CLPS samples were in the range of 0.22–0.69 (tan < 1), indicating that all samples that were studied were more elastic than viscous.
Based on the findings of the pasting properties, steady shear rheaological properties, and dynamic shear rheaological properties, the following three mechanisms might explain the improvement in the pasting and rheaological characteristics of the CLPS samples. First, such an increase in the values of the pasting, steady shear, and dynamic shear rheaological parameters for the CLPS samples might be due to the introduction of new intermolecular bridges or cross-links between the hydroxyl groups on the starch molecules of cross-linked starch.[Citation5] Cross-linking can occur when a starch is reacted with multifunctional reagents such as phosphorus oxychloride (POCl3), STMP, STPP, a mixture of adipic acid and acetic anhydride, and EPI.[Citation5] Native starch granules are only held together by hydrogen bonds. Therefore, the granules can be easily weakened by the high temperature and shear. In the present study, the cross-linking reagent STMP/STPP might have provided new cross-links at random locations in the starch granule, which might have stabilized and strengthened the granule, leading to improved rheaological properties but less sensitivity to processing conditions by shear, high temperature, or low pH than native ones.[Citation3]
Second, the improved viscosities of the CLPS samples can be explained by the strengthened rigidity and surface friction.[Citation27] During gelatinization and/or shearing, the starch granules can exude water, which can act as a lubricant and can make granules slide more easily and past adjacent granules. The viscosity is well known to be integral for all frictional resistances to flow and deformation. Therefore, more rigid granules were produced by cross-linking with STMP/STPP, and it may exude less fluid than the more deformable native starch granules, thereby producing less lubricity with greater frictional resistance and resulting in greater viscosity.
Finally, the rheaological properties of the starch pastes might be associated with the size of the swollen and hydrated starch granules. As was already mentioned, the NPS granules were completely ruptured while the CLPS granules (CLPS-0.0125, -0.025, and -0.05) were scarcely ruptured, which retarded the rate of granule swelling and maintained swollen granules after gelatinization at 95°C for 30 min (microscopic observations, ). Furthermore, SFs significantly decreased after cross-linking with STMP/STPP. Similar trends were observed in cross-linked taro starch modified by POCl3 (0.05% and 0.10%)[Citation28] and cross-linked cassava starch prepared by EPI (0.25% and 0.50%).[Citation26] Cross-linked starch granules can prevent granules from sufficient swelling and disintegrating, leading to a higher size of swollen and hydrated starch granules and consequently generating a higher viscosity.[Citation5,Citation27] Therefore, higher pasting and rheaological properties of the CLPS pastes could be associated with new cross-links between the starch granules, increased rigidity of the starch granules, and intact swollen granules of starch due to cross-linking with STMP/STPP.
Conclusion
Cross-linking with STMP/STPP resulted in remarkable changes in the physicochemical, structural, rheaological, and pasting properties of potato starch. The 31P NMR spectra confirmed successful cross-linking (DSMP, St–P–St) within starch granules when a reaction was carried out with STMP/STPP. Cross-linking with STMP/STPP (0.0125–0.05%) significantly increased the peak and final viscosity but decreased the breakdown viscosity. The apparent viscosity (ηa,100), consistency index (K), and Casson yield stress (σoc) for potato starch obtained from the power law and Casson models increased significantly by cross-linking with STMP/STPP (0.125–0.05%). The dynamic moduli (G′ and G″) values of the potato starch cross-linked by STMP/STPP (0.125–0.05%) were significantly higher than those of NPS. Therefore, cross-linking with STMP/STPP (0.125–0.05%) might be suitable for industrial applications as thickeners, stabilizers, and binders.
Additional information
Funding
References
- Pedreschi, F.;. Fried and Dehydrated Potato Products. In Singh, J., Kaur, L., Ed., Advances in Potato Chemistry and Technology; Academic Press: Elsevier, 2009.
- Kawai, K.; Takato, S.; Ueda, M.; Ohnishi, N.; Viriyarattanasak, C.; Kajiwara, K. Effects of Fatty Acid and Emulsifier on the Complex Formation and in Vitro Digestibility of Gelatinized Potato Starch. International Journal of Food Properties 2017, 20, 1500–1510.
- Maningat, C. C.; Seib, P. A.; Bassi, S. D.; Woo, K. S. Wheat Starch: Production, Properties, Modifications and Uses. In Bemiller, J. N., Whistler, R. L., Ed., Starch: Chemistry and Technology; Academic Press: Elsevier, 2009.
- Wang, L.; Liu, X.; Wang, J. Structural Properties of Chemically Modified Chinese Yam Starches and Their Films. International Journal of Food Properties 2017, 20, 1239–1250.
- Rutenberg, M.; Solarek, D. Starch Derivatives: Production and Uses. Starch: Chemistry and Technology 1984, 2, 324–328.
- Bhandari, P. N.; Singhal, R.; Kale, D. Effect of Succinylation on the Rheological Profile of Starch Pastes. Carbohydrate Polymers 2002, 47, 365–371.
- Agboola, S.; Akingbala, J.; Oguntimein, G. Physicochemical and Functional Properties of Low DS Cassava Starch Acetates and Citrates. Starch-Stärke 1991, 43, 62–66.
- Park, S.; Chung, M.; Yoo, B. Effect of Octenylsuccinylation on Rheological Properties of Corn Starch Pastes. Starch-Stärke 2004, 56, 399–406.
- Adebowale, K. O.; Lawal, O. S. Functional Properties and Retrogradation Behaviour of Native and Chemically Modified Starch of Mucuna Bean (Mucuna Pruriens). Journal of the Science of Food and Agriculture 2003, 83, 1541–1546.
- Koo, S. H.; Lee, K. Y.; Lee, H. G. Effect of Cross-Linking on the Physicochemical and Physiological Properties of Corn Starch. Food Hydrocolloids 2010, 24, 619–625.
- Lim, S.; Seib, P. Location of Phosphate Esters in a Wheat Starch Phosphate by 31p-Nuclear Magnetic Resonance Spectroscopy. Cereal Chemistry Journal 1993, 70, 145–146.
- Sang, Y.; Prakash, O.; Seib, P. A. Characterization of Phosphorylated Cross-Linked Resistant Starch by 31P Nuclear Magnetic Resonance (31P NMR) Spectroscopy. Carbohydrate Polymers 2007, 67, 201–212.
- Kahraman, K.; Koksel, H.; Ng, P. K. Optimisation of the Reaction Conditions for the Production of Cross-Linked Starch with High Resistant Starch Content. Food Chemistry 2015, 174, 173–179.
- Chung, H.; Woo, K.; Lim, S. Glass Transition and Enthalpy Relaxation of Cross-Linked Corn Starches. Carbohydrate Polymers 2004, 55, 9–15.
- Van Hung, P.; Morita, N. Physicochemical Properties of Hydroxypropylated and Cross-Linked Starches from A-Type and B-Type Wheat Starch Granules. Carbohydrate Polymers 2005, 59, 239–246.
- Wongsagonsup, R.; Pujchakarn, T.; Jitrakbumrung, S.; Chaiwat, W.; Fuongfuchat, A.; Varavinit, S.; Suphantharika, M. Effect of Cross-Linking on Physicochemical Properties of Tapioca Starch and Its Application in Soup Product. Carbohydrate Polymers 2014, 101, 656–665.
- Huang, T.; Zhou, D.; Jin, Z.; Xu, X.; Chen, H. Effect of Debranching and Heat-Moisture Treatments on Structural Characteristics and Digestibility of Sweet Potato Starch. Food Chemistry 2015, 187, 218–224.
- Woo, K.; Seib, P. Cross-Linked Resistant Starch: Preparation and Properties 1. Cereal Chemistry Journal 2002, 79, 819–825.
- American Association of Cereal Chemists (AACC). Approved Method of the AACC, Methods 08-01, 30-10, 44-15A, 46-13, 76-13.01, AACC International, 10th.; St. Paul: MN, USA, 2000.
- Williams, P.; Kuzina, F.; Hlynka, I. Rapid Colorimetric Procedure for Estimating the Amylose Content of Starches and Flours. Cereal Chemistry Journal 1970, 47, 411–421.
- Wongsagonsup, R.; Shobsngob, S.; Oonkhanond, B.; Varavinit, S. Zeta Potential (ζ) and Pasting Properties of Phosphorylated or Crosslinked Rice Starches. Starch-Stärke 2005, 57, 32–37.
- Tester, R. F.; Morrison, W. R. Swelling and Gelatinization of Cereal Starches. I. Effects of Amylopectin, Amylose, and Lipids. Cereal Chemistry Journal 1990, 67, 551–557.
- Sang, Y.; Seib, P. A.; Herrera, A. I.; Prakash, O.; Shi, Y. Effects of Alkaline Treatment on the Structure of Phosphorylated Wheat Starch and Its Digestibility. Food Chemistry 2010, 118, 323–327.
- Won, C.; Jin, Y. I.; Kim, M.; Lee, Y.; Chang, Y. H. Structural and Rheological Properties of Potato Starch Affected by Degree of Substitution by Octenyl Succinic Anhydride. International Journal of Food Properties 2017. doi:10.1080/10942912.2016.1272610.
- Code of Federal Regulations (CFR). Food Starch-Modified. Title 21, Chapter1, Part 172, Sec. 172.892. In Food Additives Permitted for Direct Addition to Food for Human Consumption; U.S. Government Printing Office: Washington, DC, 2013.
- Jyothi, A. N.; Moorthy, S. N.; Rajasekharan, K. N. Effect of Cross-Linking with Epichlorohydrin on the Properties of Cassava (Manihot Esculenta Crantz) Starch. Starch-Stärke 2006, 58, 292–299.
- Hirsch, J. B.; Kokini, J. L. Understanding the Mechanism of Cross-Linking Agents (Pocl3, STMP, and EPI) through Swelling Behavior and Pasting Properties of Cross-Linked Waxy Maize Starches 1. Cereal Chemistry Journal 2002, 79, 102–107.
- Hazarika, B. J.; Sit, N. Effect of Dual Modification with Hydroxypropylation and Cross-Linking on Physicochemical Properties of Taro Starch. Carbohydrate Polymers 2016, 140, 269–278.
- Morikawa, K.; Nishinari, K. Rheological and DSC Studies of Gelatinization of Chemically Modified Starch Heated at Various Temperatures. Carbohydrate Polymers 2000, 43, 241–247.
- Cooreman, F.; Van Rensburg, H.; Delcour, J. Pasting Profiles and Solubility of Native and Cross-Linked Corn Starch in Dimethylsulfoxide-Water Mixtures. Journal of Cereal Science 1995, 22, 251–257.
- Zhao, J.; Chen, Z.; Jin, Z.; De Waard, P.; Buwalda, P.; Gruppen, H.; Schols, H. A. Level and Position of Substituents in Cross-Linked and Hydroxypropylated Sweet Potato Starches Using Nuclear Magnetic Resonance Spectroscopy. Carbohydrate Polymers 2015, 131, 424–431.
- Manoi, K.; Rizvi, S. S. Physicochemical Characteristics of Phosphorylated Cross-Linked Starch Produced by Reactive Supercritical Fluid Extrusion. Carbohydrate Polymers 2010, 81, 687–694.
- Cho, S. A.; Kim, B. Y.; Yoo, B. Flow Behavior of Sweet Potato Starch in Mixed Sugar System. Journal of the Korean Society of Food Science and Nutrition 2008, 13, 249–252.
- Zięba, T.; Gryszkin, A.; Kapelko, M. Selected Properties of Acetylated Adipate of Retrograded Starch. Carbohydrate Polymers 2014, 99, 687–691.
- Shon, K.; Yoo, B. Effect of Acetylation on Rheological Properties of Rice Starch. Starch-Stärke 2006, 58, 177–185.
- López, O. V.; Zaritzky, N. E.; García, M. A. Physicochemical Characterization of Chemically Modified Corn Starches Related to Rheological Behavior, Retrogradation and Film Forming Capacity. Journal of Food Engineering 2010, 100, 160–168.
- Włodarczyk-Stasiak, M.; Mazurek, A.; Kowalski, R.; Pankiewicz, U.; Jamroz, J. Physicochemical Properties of Waxy Corn Starch after Three-Stage Modification. Food Hydrocolloids 2017, 62, 182–190.
- Yoneya, T.; Ishibashi, K.; Hironaka, K.; Yamamoto, K. Influence of Cross-Linked Potato Starch Treated with POCl 3 on DSC, Rheological Properties and Granule Size. Carbohydrate Polymers 2003, 53, 447–457.
- Berglund, P. T.; Shelton, D. R.; Freeman, T. P. Frozen Bread Dough Ultrastructure as Affected by Duration of Frozen Storage and Freeze–Thaw Cycles. Cereal Chemistry Journal 1991, 68, 105–107.
- Oh, J.; Kim, M.; Yoo, B. Dynamic Rheological Properties of Rice Flour-Starch Blends. Starch-Stärke 2010, 62, 321–325.