ABSTRACT
Total phenolic content and antioxidant activity of hydroalcoholic extract of avocado leaves (Persea americana (PA)) were determined by α,α-diphenyl-β-picrylhydrazyl, ∙NO, O2∙–, and ∙OH radical capture and reducing power test. Liquid chromatography coupled to mass spectrometry/electron spray ionization was used to identify 19 types of polyphenols, as flavan-3-ols and flavonol glycosides, in PA. Stability assays were performed to optimize conditions for preserving antioxidant activity and polyphenols during drying, thermal incubation, and storage of the extract. Extracts, obtained from leaves dried at 70°C or higher, showed lower values for total phenolic content and antioxidant activity. Compared with the values of leaves dried at 60°C or lower, these differences were statistically significant (p ≤ 0.05). Incubating PA in solution, at 40°C–100°C for up to 8 h, did not produce significant changes in total phenolic content or antioxidant activity. No statistically significant changes in the total phenolic content or antioxidant activity (p ≤ 0.05) occurred after storage at 4°C and 25°C for up to 10 months. PA was shown to possess diverse polyphenolic compounds with robust antioxidant activity. Precise control of drying temperature was an important parameter for maintaining high levels of phenolic compounds and antioxidant activity of PA.
Introduction
The avocado tree (Persea americana (PA) Mill.), native to Central America and Mexico, and now cultivated in various parts of the world including Brazil, presents great commercial value because of the relevant nutritional utilization of its fruit.[Citation1,Citation2] The other parts of the plant, such as peel, seed, and leaves, may also contain bioactive compounds and, thus, may contribute to the development of avocado by-products for commercial purposes.[Citation3–Citation5] Avocado seeds and leaves, as aqueous and hydroalcoholic extracts, are utilized in folk medicine because of their anti-viral,[Citation6] anti-inflammatory,[Citation7] anti-diabetic,[Citation8] hypoglycemic,[Citation8] and antioxidant[Citation1] properties; these beneficial biological properties may be related to the presence of polyphenolic compounds. Studies have reported on the presence of phenolic acids, flavonoids, and procyanidins in avocado leaves.[Citation6,Citation8,Citation9]
Polyphenols have attracted attention because of their therapeutic value, which is related to their antioxidant activities, as well as anti-mutagenic, anticarcinogenic, and antimicrobial properties.[Citation10–Citation12] Specifically, the antioxidant activities of plant polyphenols have attracted the interest of the pharmaceutical and food industries. The pharmaceutical industry is interested in the ability of these compounds to inhibit lipid peroxidation[Citation13,Citation14] and capture reactive species such as hydrogen peroxide (H2O2), the hydroxyl radical (∙OH), and radical anion superoxide (O2∙-);[Citation15] the oxidative stress, caused by these reactive species, is related to various pathologies including neurodegenerative[Citation16] and cardiovascular diseases,[Citation17] cancer,[Citation18] and ageing.[Citation19] In the food industry, the antioxidant activity of polyphenols may be applicable in food preservation.[Citation4] Currently, synthetic compounds such as butyl hydroxyanisole and butylated hydroxytoluene (BHT) are used for this purpose.[Citation20] Although the amount of synthetic preservatives, ingested in the human diet, is low and likely non-toxic,[Citation20,Citation21] the safety of using these compounds, with respect to their effects on human health, remains controversial. In mice, the intake of high concentrations of these compounds induces genotoxicity[Citation22] and carcinogenesis.[Citation20,Citation21,Citation23]
The antioxidant activity of plant extracts is intimately related to the concentration of phenolic compounds.[Citation24,Citation25] However, the concentration and stability of the phenolic compounds is influenced by diverse factors, including the drying processes of plant material in natura, and the storage conditions of the resultant extracts.[Citation13,Citation14,Citation26,Citation27] High temperatures, used to dry the plant material and prepare extracts in solution, can lead to a decreased content of phenolic compounds.[Citation26,Citation27] The stability of polyphenol-rich extracts is influenced by storage conditions such as temperature, time of storage, pH, and the solvent used.[Citation13,Citation14,Citation28] Extended storage can lead to chemical modifications, such as hydrolysis or oxidation, which promote a decrease in the content of phenolic compounds such as anthocyanins and aglycone flavonoids.[Citation29] Therefore, it is important to evaluate the stability and potential applications of polyphenol-rich extracts because these compounds may be sources of natural antioxidants.
In this study, we measured the phenolic content in a hydroalcoholic extract of avocado leaves and related this content to in vitro antioxidant activity. Stability assays were performed to optimize conditions for preserving antioxidant activity and polyphenolic compounds during preparation (drying process), as well as during thermal incubation and storage, of the extract in solution under varying conditions.
Material and methods
Chemicals and reagents
BHT, α,α-diphenyl-β-picrylhydrazyl (DPPH), gallic acid (GA), phenazine methosulfate (PMS), quercetin (Que), and salicylic acid were obtained from Sigma-Aldrich (St. Louis, MO, USA). Reduced nicotinamide adenine dinucleotide (NADH) and nitroblue tetrazolium (NBT) were obtained from Amresco (Dallas, TX, USA). The Folin–Ciocalteu reagent was obtained from Chromate (São Paulo, Brazil). All other chemicals and standards were commercial products of the highest available purity.
Hydroalcoholic extract of avocado leaves
The leaves were sanitized using 70% v/v ethanol in purified water, dried in a laboratory furnace at 50°C, and ground using a food processor (Philips Walita Co, Varginha, MG, Brazil). The dried leaves were stored in the dark, in 70% ethanol (100 g/500 mL), for 4 days at 25°C, and then vacuum filtrated using Whatman™ Grade 4 Qualitative Filter Paper (Sigma-Aldrich). The extract was concentrated using a rotary evaporator at 40°C, freeze dried (FD), and designated as PA. The total phenolic content of this extract was determined using the Folin–Ciocalteu method; antioxidant activity was determined using DPPH; nitric oxide (∙NO), superoxide anion (O2∙-), and hydroxyl (∙OH) radical capture assays and the reducing power test (). Liquid chromatography coupled to mass spectrometry/electron spray ionization (LC-MS/ESI) was used to characterize the polyphenolic profile of the PA extract. The pH of the PA extract was 6.27 ± 0.09, determined using a digital pH meter (GEHAKA, São Paulo, Brazil). Additionally, the PA extract was designated as the standard extract and was used in the stability assays to evaluate the effects of incubating for 0, 3, and 8 h at 40°C–90°C. We also assessed the effects of storing the PA extract at 25°C and 40°C for 0–10 months. Because polyphenol-rich extracts are light sensitive, the extracts were protected from light in all the experiments employed in this study.
Figure 1. Schematic of the procedures for the stability assay. For more details on the terminology used to designate the fractions, see the Materials and Methods section. LC-MS/ESI: Liquid chromatography coupled with mass spectrometry/electron spray ionization analysis; DPPH: 1,1-diphenyl-2-picryl-hydrazyl; ·NO: nitric oxide; O2·–: anion superoxide; and ·OH: hydroxyl capture assays. Total phenolic determinations were performed at least five times, on different days, in triplicate for each sample. Determinations of antioxidant activity (evaluated using DPPH, the reducing power test, and •NO, •OH, and O2·– capture assays) were performed at least twice, in triplicate, for each concentration of each sample tested.
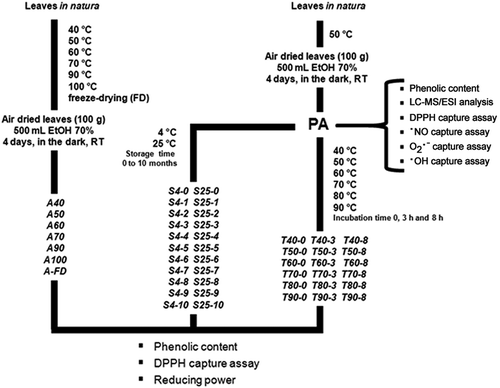
Determination of the total phenolic content
The total phenolic content was determined using the Folin–Ciocalteu method by Singleton and Rossi Jr.[Citation30] with modifications. Folin–Ciocalteu reagent (1.25 mL) was combined with the sample of the extract (250 µL) and then Na2CO3 (7.5% w/v) (1.0 mL) was added to the mixture. The mixture was incubated in the dark at 100°C for 10 min. Aliquots of the samples (200 µL) were transferred into 96-well plates, and the absorbance was measured at 760 nm using a microplate reader spectrophotometer (Bio Tek, model EPOCH, ELX800, Winooski, VT, USA). The total phenolic content was expressed as GA equivalents in micrograms per milligrams (μg/mL) of dry extract.
In vitro antioxidant activity
1,1-diphenyl-2-picryl-hydrazyl (DPPH) free radical capture
The DPPH radical capture rate (CR) of the extracts was measured using a method adapted from Blois.[Citation31] Initially, aliquots (300 µL) of samples (10–640 µg/mL) or standards (GA, Que, BHT; 1.25–640 µg/mL) were combined with 40 mM DPPH radical solution (100 µL) and incubated in the dark for 20 min, at 25°C. Aliquots of the samples (200 µL) were transferred into 96-well plates and absorbance was measured at 515 nm using a microplate reader spectrophotometer (Bio Tek). The CR was determined using the equation: CR (%) = {[A0 – (A –Ab)]/A0} × 100, where A0 indicates the absorbance of the DPPH solution alone; A is the absorbance of the sample and DPPH; and Ab is the absorbance of the sample without DPPH.
Hydroxyl free radical (•OH) capture
The capability of the extracts to capture the hydroxyl radical was determined using the method described by Halliwell and Gutteridge.[Citation32] Hydroxyl radicals, produced using a mixture of 8.8 mM hydrogen peroxide (H2O2) (200 µL) and 6 mM ferrous sulfate (FeSO4) (200 µL), were added to 200 µL of the PA extract (20–640 µg/mL) or standards (GA and ascorbic acid [AA]; 1.25–640 µg/mL). After 10 min, 6 mM salicylic acid (200 µL) was added and the solution was incubated for an additional 20 min, at 25°C. Aliquots of the samples (200 µL) were transferred into 96-well plates, and absorbance at 510 nm was measured using a spectrophotometer (Bio Tek). The CR was determined using the equation: CR (%) = {[A0 – (A –Ab)]/A0} × 100, where A0 is the absorbance of the H2O2, FeSO4, and salicylic acid solution only; A is the absorbance of the sample with H2O2, FeSO4, and salicylic acid; and Ab is the absorbance of the sample only.
Capture of the superoxide anion radical (O2•–)
The ability of extracts to capture the superoxide anion was determined using a method described by Nishikimi et al.[Citation33] Aliquots (900 µL) of PA extract (20–640 µg/mL) or standards (GA, AA, and Que; 1.25–640 µg/mL) were dissolved in 10 mM Tris–HCl (pH 8.0), added to a solution in a cuvette containing 72 µM NBT (20 µL) and 30 µM PMS (20 µL), and incubated for 5 min at 25°C. Then, aliquots (60 µL) of 338 µM NADH were added and the absorbance at 560 nm was measured using a spectrophotometer (SP 2000 UV model, Bel Photonics, Monza, ITA).
This method is based on the reduction of NBT by NADH, which consumes the superoxide anion present in the solution. Thus, a higher rate of O2– capture by samples of the extract results in lower reduction of NBT and lower absorbance at 560 nm. The CR was determined using the equation: CR (%) = {[A0 – (A –Ab)]/A0} × 100, where A0 is the absorbance of the Tris–HCl buffer, NBT, PMS, and NADH solution alone; A is the absorbance of the sample in the presence of NBT, PMS, and NADH; and Ab is the absorbance of the sample alone.
Capture of the nitric oxide free radical (•NO)
The rate of nitric oxide capture by the extracts was determined using a method described by Marcocci, Maguire, Groy-Lefaix, and Packer.[Citation34] Nitric oxide radicals, produced by 10 mM sodium nitroprusside in phosphate buffer (pH 7.4) (250 µL), were incubated for 120 min with 250 µL of PA extract (20–640 µg/mL) or standards (GA, Que, and BHT; 1.25–640 µg/mL), at 25°C. Aliquots (100 µL) were transferred into 96-well plates and 100 µL of Griess reagent was added; absorbance was measured at 546 nm using a microplate reader spectrophotometer (Bio Tek). The CR was determined using the equation: CR (%) = {[A0 – (A –Ab)]/A0} × 100, where A0 is the absorbance of sodium nitroprusside and Griess reagent; A is the absorbance of sodium nitroprusside, Griess reagent, and sample; and Ab is the absorbance of the sample and sodium nitroprusside.
Reducing power test
The reducing power of the extracts was determined using the method adapted from Oyaizu.[Citation35] Samples (250 µL) of PA extract (10–640 µg/mL) or standards (GA and AA; 1.25–640 µg/mL) were mixed with 0.2 M sodium phosphate buffer (pH 6.6) (250 µL) and potassium ferricyanide (1% w/v) (250 µL). After 20 min of incubation at 50°C, trichloroacetic acid (10% w/v) (250 µL) was added, and the aliquots (200 µL) were transferred into 96-well plates; then, ferric chloride (0.1% w/v) (50 µL) was added. The absorbance of the samples was then measured at 700 nm using a microplate reader spectrophotometer (Bio Tek). This assay is based on the concept that substances with reducing power can reduce potassium ferricyanide in solution, generating potassium ferrocyanide, which reacts with ferric chloride to form a complex with maximum absorption at 700 nm. Thus, higher absorbance at 700 nm indicates higher reducing power of the sample.
LC-MS/ESI analysis
Analyses of polyphenol content, using LC-MS/ESI, were conducted using Agilent 1200 series coupled with a triple quadrupole MS series 6400 (Santa Clara, CA, USA). Separation was performed using a reverse-phase column (C18, 150 × 2.1 mm, 5 µm; Shim-Pack CLC-ODS, Shimadzu, Kyoto, Japan) at 25°C. The mobile phase consisted of 0.5% formic acid in water (solvent A) and 0.5% formic acid in acetonitrile (solvent B) using the following gradients: 0–10 min: 10% B; 10–20 min: 10–30% B; 20–30 min: 30–50% B; 30–32 min: 50% B; 32–38 min: 50–10% B; 38–48 min: 10% B. The flow rate was 1.0 mL/min and injection volume was 5 μL. To remove low-molecular-weight compounds, the samples were eluted through C18 cartridges (Varian, Palo Alto, CA, USA) before analysis with LC-MS/ESI. The parameters for ESI were as follows: nebulizer at 50 psi, 4500 V, arrest gas (N2) flow at 11 L/min, and ionization temperature at 350°C. The ion trap mass spectrometer was operated in negative ion mode with a full scanning range from m/z 150 to m/z 1000.
Stability assays
Effects of the air-drying process on avocado leaf extracts
Avocado leaves were FD or air dried at 40°C, 50°C, 60°C, 70°C, 90°C, or 100°C in a laboratory furnace. The leaves were then ground in a food processor, generating a duplicate for each condition. The leaves were FD or air dried under the six drying temperatures, until constant weight. Then, the dried leaves were subjected to a hydroalcoholic extraction (as described previously in the Hydroalcoholic extract of avocado leaves section), stored in the dark in 70% ethanol (100 g/500 mL) for 4 days at 25°C, and vacuum filtrated using filter paper (Sigma-Aldrich). The filtrated extracts were then concentrated using a rotary evaporator at 40°C, FD, and designated as A-FD, A40, A50, A60, A70, A90, and A100 according to the drying method or condition used. All the hydroalcoholic extracts were evaluated for total phenolic content and antioxidant activity using the DPPH radical capture and reducing power test ().
Effects of thermal incubation and time of storage on avocado leaf extracts in solution
The standard PA extract was solubilized (50 mg/mL in 70% ethanol) and subjected to six different drying temperatures (40°C, 50°C, 60°C, 70°C, 80°C, or 90°C) in a thermostatic bath, generating a duplicate for each temperature. The extracts were maintained at each temperature for up to 8 h with aliquots removed after 0, 3, and 8 h of incubation. The aliquots were FD, generating fractions designated as T40-0, T40-3, T40-8, T50-0, T50-3, T50-8, T60-0, T60-3, T60-8, T70-0, T70-3, T70-8, T80-0, T80-3, T80-8, and T90-0, T90-3, T90-8, according to the temperature and time of incubation used. All the samples were evaluated for the total phenolic content and antioxidant activity using the DPPH radical capture and the reducing power test ().
Effect of storage conditions on avocado leaf extracts in solution
The standard extract of PA was solubilized in 70% ethanol (50 mg/mL) and stored at 4°C and 25°C for up to 10 months. Aliquots, generated in duplicate per each storage temperature, were collected each month and FD, generating extracts designated as S4-0, S4-1, S4-2, S4-3, S4-4, S4-5, S4-6, S4-7, S4-8, S4-9, S4-10, and S25-0, S25-1, S25-2, S25-3, S25-4, S25-5, S25-6, S25-7, S25-8, S25-9, and S25-10 according to the temperature and time of storage. All samples were evaluated for the total phenolic content and antioxidant activity using DPPH radical capture and the reducing power test ().
Statistical analysis
The determination of total phenolic content was performed at least five times, on different days, in triplicate for each sample. The determination of antioxidant activity (using DPPH, the reducing power test, and •OH, O2•-, and •NO radical capture assays) was performed at least twice in triplicate for each concentration of each sample tested. All the results were analysed by one-way analysis of variance followed by Tukey’s multiple comparison test. The differences were considered statistically significant at p ≤ 0.05. The half maximal effective concentration (EC50), corresponding to the concentration able to capture 50% of free radicals, was calculated using linear equations (r2 ≥ 0.95) obtained by linearizing the activity curves. Pearson’s correlation was used to correlate the effects of air-drying temperature with the total phenolic content and antioxidant activity. Significance was tested by Student’s t test and Pearson’s correlation coefficient (r).
Results and discussion
Chemical characterization of polyphenol compounds and antioxidant activity of avocado leaf extract
The leaves, dried at 50°C, were subjected to a hydroalcoholic extraction and FD, generating PA extract with a yield of 22.9% (w/w) and total phenolic content of 237.1 ± 25.1 µg per GA equivalents/mg of dry extract. The LC-MS/ESI analysis was performed to identify the polyphenol constituents of PA extract. LC-MS/ESI provides information on polyphenol structures in plant extracts without the need to isolate the compounds beforehand.[Citation36–Citation38] The chromatogram of PA extract is presented in . Baseline separation of 26 compounds was achieved within a 30-min run. Nineteen of these compounds were identified based on mass spectra and by comparing the results with reference compounds from the literature[Citation3,Citation6,Citation9,Citation39,Citation40] ().
Table 1. Polyphenol compounds of avocado leaf extract identified by LC-MS/ESI.
Figure 2. LC-MS/ESI chromatogram of avocado leaf extract. Numbers in the chromatogram refer to the compounds described in . For information of the MS spectra, see and Fig. S1.
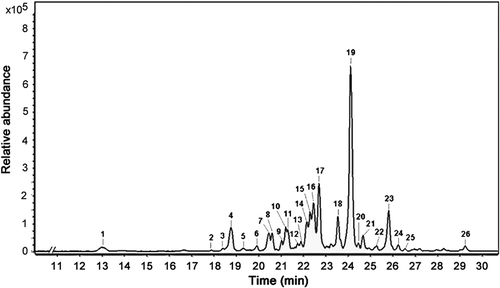
Peaks 1, 4, and 6 presented [M-H]– ions at the m/z values of 577.1, 865.1, and 865.1, respectively, which are characteristic of typical flavan-3-ol compounds. Peak 1 was, therefore, designated as a procyanidin dimer, likely procyanidin B1. Peaks 4 and 6 were identified as procyanidin C1 and another procyanidin trimer, respectively. For peaks 7–25, all identified constituents were classified as flavonol (kaempferol/quercetin) glycosides. Peaks 7, 10, and 11 suggested the presence of tri-glycosylated kaempferol, considering the [M-H]– ions observed at the m/z values of 739.2, 741.1, and 741.2, respectively. Mono-glycosylated flavonols, such as kaempferol-3-O-arabinoside (peak 21, [M-H]– ion at m/z 417.1), kaempferol-3-O-glucoside (astragalin; peaks 20 and 22; [M-H]– ion at m/z 447.1), and kaempferol-3-O-rhamnoside (afzelin; peaks 23 and 24; [M-H]– ion at m/z 431.1), were also identified.
For quercetin-O-glycosides, we identified the presence of quercetin-3-O-[rhamnosyl-glucoside]-7-rhamnoside (peak 8; [M-H]– ion at m/z 755.2), quercetin-3-O-arabinoglucoside (peltatoside; peak 12; [M-H]– ion at m/z 595.0), quercetin-3-O-rhamnoglucoside (rutin; peak 14; [M-H]– ion at m/z 609.2), quercetin-3-O-galactoside (hyperoside; peak 16; [M-H]– ion at m/z 463.1), and quercetin-3-O-glucoside (isoquercitrin; peak 17; [M-H]– ion at m/z 463.1).[Citation6] Peak 18 presented two main [M-H]– ions at m/z 433.1 and 505.1, which may be avicularin (quercetin-3-O-arabinoside) and quercetin-3-O-glucose-6′′-acetate,[Citation6] respectively. Peak 19 ([M-H]– ion at m/z 447.1) was identified as quercitrin (quercetin-3-O-rhamnoside).[Citation6,Citation39] Last, peak 26 was designated as flavone luteolin-8-C-glucoside (orientin; [M-H]– ion at m/z 447.0). The mass spectra are shown in Supplementary Fig. S1.
The content of phenolic compounds is closely correlated with the total antioxidant activity of plant extracts.[Citation24,Citation25] The antioxidant activities of PA extract were evaluated using DPPH, capture of ∙NO, O2∙–, and ∙OH radicals, and reducing power assays in vitro. The results are shown in Supplementary Fig. S2. Except for the results of the reducing power assay, all the values of EC50 are presented in . The DPPH EC50, calculated for PA extract, was 57.8 ± 5.4 µg/mL. For the standards GA, Que, and BHT, the DPPH EC50 values were 9.2 ± 1.3, 9.4 ± 1.4, and 21.0 ± 1.4 µg/mL, respectively (). The antioxidant activity of PA extract, at 320 µg/mL, was approximately 70% of an equivalent concentration of BHT or GA, as determined by the DPPH assay (Supplementary Fig. S2). The EC50 values for PA extract, determined using the ∙OH, ∙NO, and O2∙– radical capture assays, were 171.6 ± 5.4, 149.0 ± 9.3, and 99.3 ± 4.7 µg/mL, respectively (). PA extract showed antioxidant activity that was equivalent to 52% of BHT activity at the same concentration, as determined by the reducing power assay (Supplementary Fig. S2).
Table 2. EC50 values for the PA extract and standards calculated for DPPH, •NO, •OH, and O2•– capture assays.
Previous studies, using DPPH, have described the high antioxidant activity of methanolic extracts, obtained from PA leaves, with respect to free radical scavenging and capture.[Citation1,Citation41] However, those studies did not assess the values of EC50. The antioxidative capacity of our extract, calculated as the percentage of DPPH activity, was similar to that described in those studies.[Citation1,Citation41] Except for the assays determining the capture of the ∙NO radical, and the reducing power test,[Citation1] we did not find any studies assessing the ∙OH and O2∙– radical scavenging activities of the PA leaf extracts. Our study shows that avocado leaves are a good source of antioxidants; the levels of polyphenol compounds and antioxidant activity of PA extract were comparable to those of other leaf extracts.[Citation5,Citation14,Citation42,Citation43] For example, the concentration of phenolic compounds in PA extract (237.1 ± 25.1 µg/mg) was higher than those in the extracts of Camelia sinensis (58.7 ± 2.9 µg/mg),[Citation43] mulberry (Morus indica L.) (71.0 ± 2.5–93.2 ± 1.0 µg/mg),[Citation14] blackberry (Rubus sp.) (48.5 ± 5.1 µg/mg), raspberry (Rubus idaeus L.) (54.7 ± 3.8 µg/mg), or strawberry (Fragaria × ananassa D.) (30.9 ± 3.1 µg/mg).[Citation42] In terms of antioxidant activity, determined using DPPH under the conditions described previously, the EC50 (µg/mL) values of extracted avocado leaves were similar to those of Camelia sinensis (37.0 ± 0.2 µg/mL)[Citation43] and mulberry (Morus indica L.) (79.5 ± 0.8 µg/mL).[Citation14]
Effect of air-drying temperature on the concentration of phenolic content and antioxidant activity of PA extracts
After being dried using one of the seven different conditions (40°C, 50°C, 60°C, 70°C, 90°C, or 100°C or FD), the avocado leaves showed an average moisture content of 61.4% ± 2.2%. The rate of dehydration increased with the increasing air-drying temperature; the times of air drying were approximately 43.5 h (40°C), 19.2 h (50°C), 7.1 h (60°C), 6.2 h (70°C), 3.2 h (90°C), and 1.0 h (100°C). The dried leaves were subjected to hydroalcoholic extractions and FD, generating the fractions A40, A50, A60, A70, A90, A100, and A-FD, with an average yield of 22.6 ± 4.4% (w/w). The content of phenolic compounds varied between 130.0 ± 7.6 and 233.3 ± 36.8 µg of GA equivalents/mg of dry extract; the differences in the values were statistically significant (p ≤ 0.05) (). The air-drying temperatures were negatively correlated with the concentrations of phenolic compounds (Pearson’s correlation: r = −0.81; p = 0.026), suggesting that high temperatures generate extracts with lower concentrations of phenolic compounds ().
Figure 3. Characteristics of avocado leaf extract derived from leaves subjected to different drying conditions. Avocado leaf extracts were analysed by measuring the total phenolic content (A), DPPH radical capture test (B), and reducing power test (C). The resulting values are expressed as a mean ± standard deviation calculated according to each method. In (A), different letters mean significant differences as determined using Tukey’s test (*p ≤ 0.05). Results from Pearson correlation analyses (D and E) are also provided.
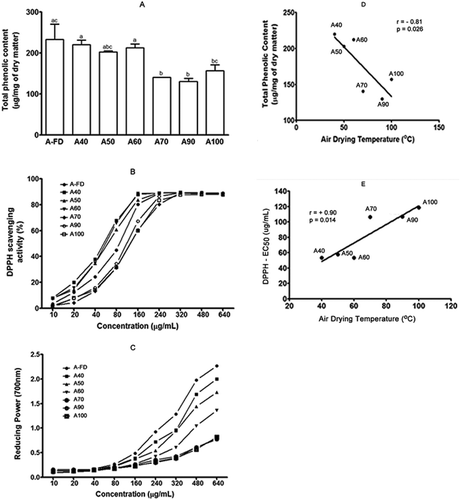
Compared with the total phenolic content and antioxidant activity values, samples air dried at 70°C, 90°C, and 100°C showed a lower total phenolic content and antioxidant activity than samples that had been air drying at 40°C, 50°C, and 60°C (a and b). The EC50 values for all fractions are described in . A linear correlation, between the air-drying temperature and antioxidant activity, showed that higher drying temperatures contributed significantly to decreased antioxidant activity. This was determined by DPPH and calculated by the increase in the values of EC50 (Pearson’s correlation: r = +0.90; p = 0.014; ) and by the reducing power test (Pearson’s correlation: r = −0.89; p = 0.016; n = 6, calculated at the concentration of 640 μg/mL; data not shown). We also verified a linear correlation between the total phenolic content and antioxidant activity (DPPH: Pearson’s correlation: r = −0.93; p = 0.003; n = 6) and the reducing power test (Pearson’s correlation: r = +0.92; p = 0.005; n = 6, calculated at the concentration of 640 µg/mL; data not shown). This suggests that the high drying temperatures decreased the concentrations of phenolic compounds in the leaves, and consequently decreased the antioxidant activity of the resulting extracts.
Table 3. DPPH EC50 values of the fractions obtaining from stability assays of avocado leaf extract.
According to Maillard and Berset,[Citation44] the variation in the phenolic content of the samples, subjected to high temperatures, may occur via three possible mechanisms: by the release of phenolic acid derivatives bound to macromolecules such as cumaric acid linked to lignin or ferulic acid linked to arabinoxylans; by degradation of a part of lignin and release of phenolic acids such as hydroxycinnamic acid; or by thermal degradation. Several studies have reported that air-drying temperatures, from 90°C to 160°C, can produce extracts containing melanoidins, which have robust antioxidant activity.[Citation45,Citation46] Nevertheless, the majority of reports have suggested that high drying temperatures can decrease the concentration of phenolic compounds in plant extracts via thermal degradation.[Citation26,Citation27] The effect of air-drying temperature on the stability of polyphenols and antioxidant activity in mulberry leaves was described by Katsube et al.,[Citation26] indicating that temperature under 60°C did not affect the stability of phenolic content. However, there was a significant and progressive reduction in antioxidant activity when temperature over 70°C was employed, suggesting that thermal degradation was the main mechanism, which supports our findings. Thus, precise control of the drying temperature is important for maintaining high levels of phenolic compounds and antioxidant properties of avocado leaf extracts.
Effect of temperature and time of incubation on the concentration of phenolic content and antioxidant activity of avocado leaf extracts in solution
Incubating the PA extract at various temperatures (40°C, 50°C, 60°C, 70°C, 80°C, and 90°C) for 0, 3, and 8 h did not show a significant effect of temperature on either the total phenolic content or antioxidant activity (Supplementary Fig. S3). These results indicate that the phenolic compounds in PA are stable at the tested temperatures. The phenolic content in all the samples ranged between 197.4 ± 9.5 and 259.3 ± 28.4 µg of GA equivalents/mg of dry extract, and no values differed significantly from that of the PA control extract (237.1 ± 25.1 µg of GA equivalents/mg of dry extract) (Fig. S3, Supplementary Material). The DPPH EC50 values of samples, subjected to different drying temperatures for 8 h, varied between 61.5 ± 1.7 and 77.8 ± 8.1 µg/mL; however, even after exposure to the highest drying temperatures, antioxidant activity was not significantly different from that of the PA control extract (DPPH EC50 = 57.8 ± 5.4 µg/mL) (). A similar result was obtained when antioxidant activity was determined by the reducing power assay (Fig. S3, Supplementary Material).
Thermal processing can influence the stability of polyphenol-rich extracts in solution.[Citation13,Citation14,Citation26] High temperatures can decrease the levels of total phenolic content and affect the profile of these extracts.[Citation13,Citation14,Citation26] The different structures of phenolic components impart these components with different levels of heat sensitivity; temperatures, above 70°C, may affect the chemical structure of these components according to their level of heat sensitivity.[Citation13,Citation14,Citation26] For example studies reports that glycosylated flavonoids are more resistant to heat treatment than aglycone flavonoids and other types of polyphenols such as procyanidins.[Citation13] Under the conditions tested in our study, the polyphenolic content of PA in solution was stable and maintained its antioxidant activity at all the tested temperatures; this can be related to the presence of the various types of glycosylated flavonoids in this extract.
Effect of storage conditions on total phenolic content and antioxidant activity of avocado leaf extracts in solution
During assessments of storage temperature (at 4°C and 25°C), the total phenolic content of the avocado leaf extract remained statistically similar for nearly a year; the values varied from 201.6 ± 8.0 to 250.8 ± 6.6 and from 225.4 ± 20.4 to 271.5 ± 33.8 µg of GA equivalents/mg of dry extract for 4°C and 25°C, respectively. Details are presented in Figs. S4 and S5 (Supplementary Material). The DPPH EC50 values were similar to that of PA control extract (57.8 ± 5.4 µg/mL), varying by 57.9 ± 14.0 to 91.1 ± 7.1 and 48.5 ± 14.4 to 81.7 ± 3.8 µg/mL at 4°C and 25°C, respectively (, Figs. S4 and S5, Supplementary Material). The antioxidant activity of PA and other extracts, subjected to different times of storage, did not change significantly at either 4°C or 25°C (Figs. S4 and S5, Supplementary Material).
We assessed the effects of temperature (at 4°C and 25°C) and duration of storage on the stability of phenolic compounds.[Citation13,Citation14,Citation28] Extended storage can promote reactions that may affect the phenolic content and, thus, cause alterations in organoleptic characteristics including colour and odour; this is especially pertinent with respect to beverages such as wine and juices.[Citation13,Citation28,Citation29,Citation47] Marques et al.[Citation28] used three different varieties of wine to show that temperature and extended storage can affect the levels of anthocyanins and flavonoids without altering their antioxidant properties. In apple juice, several phenolic compounds were stable during storage at the conditions tested.[Citation13] The total phenolic content of wine did not show significant changes after 12 months of storage at 25°C.[Citation47] In this study, we showed that the polyphenolic content in a hydroalcoholic extract of avocado leaves did not vary for nearly a year when stored at 4°C and 25°C.
Conclusion
This study indicates that the hydroalcoholic extract of avocado leaves (designated as PA) is a good source of diverse polyphenol compounds, identified as flavan-3-ols and flavonol glycosides, with a robust antioxidant activity comparable to those of other plant species possessing known antioxidant properties. In solution, the PA extract was stable from 40°C to 90°C for up to 8 h. The PA extract was also stable when stored from 0 to 10 months at 4°C and 25°C. However, the drying process of the plant material requires precise temperature control; this is the most important parameter for maintaining high levels of phenolic compounds and antioxidant properties of avocado leaf extract.
LJFP_A_1369105_Supplementary_Material.docx
Download MS Word (1 MB)Acknowledgments
The authors thank CAPES for the fellowship granted to F. T. Yamassaki. The authors declare that there are no conflicts of interest.
Supplementary material
Supplemental data for this article can be accessed on the publisher’s website.
Additional information
Funding
References
- Asaolu, M.F.; Asaolu, S.S.; Fakunle, J.B.; Emman-Okon, B.O.; Ajayi, E.O.; Togun, R.A. Evaluation of In-Vitro Antioxidant Activities of Methanol Extracts of Persea americana and Cnidosculus aconitifolius. Pakistan Journal of Nutrition 2010, 9, 1074–1077.
- Di Stefano, V.; Avellone, G.; Bongiorno, D.; Indelicato, S.; Massenti, R.; Lo Bianco, R. Quantitative Evaluation of the Phenolic Profile in Fruits of Six Avocado (Persea americana) Cultivars by Ultra-High-Performance Liquid Chromatography Heated Electrospray-Mass Spectrometry. International Journal of Food Properties 2017, 20, 1302–1312.
- Kosińska, A.; Karamać, M.; Estrella, I.; Hernández, T.; Bartolomé, B.; Dykes, G.A. Phenolic Compound Profiles and Antioxidant Capacity of Persea americana Mill. Peels and Seeds of Two Varieties. Journal of Agricultural and Food Chemistry 2012, 60, 4613–4619.
- Yamassaki, F.T.; Bovo, F.; Campestrini, L.H.; Zawadzki-Baggio, S.F.; Maurer, J.B.B. Use and Obtaining Extracts of Leaves of Persea americana Mill. As Antioxidants and Preservatives. Patent Registered in “Instituto Nacional Da Propriedade Industrial” (INPI) by Federal University of Paraná, Process number: BR1020130287431, deposit date: 11/07/2013, Paraná, Brazil.
- Chen, G.-L.; Zhang, X.; Chen, S.-G.; Han, M.-D.; Gao, Y.-Q. Antioxidant Activities and Contents of Free, Esterified and Insoluble-Bound Phenolics in 14 Subtropical Fruit Leaves Collected from the South of China. Journal of Functional Foods 2017, 30, 290–302.
- De Almeida, A.P.; Miranda, M.M.F.S.; Simoni, I.C.; Wigg, M.D.; Lagrota, M.H.C.; Costa, S.S. Flavonol Monoglycosides Isolated from the Antiviral Fractions of Persea americana (Lauraceae) Leaf Infusion. Phytotherapy Research 1998, 12, 562–567.
- Adeyemi, O.O.; Okpo, S.O.; Ogunti, O.O. Analgesic and Anti-Inflammatory Effects of the Aqueous Extracts of Leaves of Persea americana Mill (Lauraceae). Fitoterapia 2002, 73, 375–380.
- Lima, C.R.; Vasconcelos, C.F.B.; Costa-Silva, J.H.; Maranhao, C.A.; Costa, J.; Batista, T.M.; Carneiro, E.M.; Soares, L.A.L.; Ferreira, F.; Wanderley, A.G. Anti-Diabetic Activity of Extract from Persea americana Mill. Leaf via the Activation of Protein Kinase B (Pkb/Akt) in Streptozotocin-Induced Diabetic Rats. Journal of Ethnopharmacology 2012, 141, 517–525.
- Jiménez, P.; García, P.; Bustamante, A.; Barriga, A.; Robert, P. Thermal Stability of Oils Added with Avocado (Persea americana Cv. Hass) or Olive (Olea europaea Cv. Arbequina) Leaf Extracts during the French Potatoes Frying. Food Chemistry 2017, 221, 123–129.
- Rice-Evans, C.A.; Miller, N.J.; Paganga, G. Antioxidant Properties of Phenolic Compounds. Trends in Plant Science Reviews 1997, 2, 152–159.
- Reddy, L.; Odhav, B.; Bhoola, K.D. Natural Products for Cancer Prevention: A Global Perspective. Pharmacology & Therapeutics 2003, 99, 1–13.
- Almajano, M.P.; Carbó, R.; Jiménez, J.A.L.; Gordon, M.H. Antioxidant and Antimicrobial Activities of Tea Infusions. Food Chemistry 2008, 108, 55–63.
- Van Der Sluis, A.A.; Dekker, M.; Boekel, M.A.J.S. Activity and Concentration of Polyphenolic Antioxidants in Apple Juice. 3. Stability during Storage. Journal of Agricultural and Food Chemistry 2005, 53, 1073–1080.
- Arabshahi-Delouee, S.; Urooj, A. Antioxidant Properties of Various Solvent Extracts of Mulberry (Morus indica L.) Leaves. Food Chemistry 2007, 102, 1233–1240.
- Siddhuraju, P.; Becker, K. Antioxidant Properties of Various Solvent Extracts of Total Phenolic Constituents from Three Different Agroclimatic Origins of Drumstick Tree (Moringa oleifera Lam.) Leaves. Journal of Agricultural and Food Chemistry 2003, 51, 2144–2155.
- Scheibmeir, H.D.; Christensen, K.; Whitaker, S.H.; Jegaethesan, J.; Clancy, R.; Pierce, J.D. A Review of Free Radicals and Antioxidants for Critical Care Nurses. Intensive and Critical Care Nursing 2005, 21, 24–28.
- Heitzer, T.; Schlinzig, T.; Krohn, K.; Meinertz, T.; Münzel, T. Endothelial Dysfunction, Oxidative Stress, and Risk of Cardiovascular Events in Patients with Coronary Artery Disease. Circulation 2001, 104, 2673–2678.
- Valko, M.; Rhodes, C.J.; Izakovic, M.; Mazu, M. Free Radicals, Metals and Antioxidants in Oxidative Stress-Induced Cancer. Chemico-Biological Interactions 2006, 160, 1–40.
- Del Valle, L.G. Oxidative Stress in Aging: Theoretical Outcomes and Clinical Evidences in Humans. Biomedicine & Aging Pathology 2011, 1, 1–7.
- Williams, G.M.; Iatropoulos, M.J.; Whysner, J. Safety Assessment of Butylated Hydroxyanisole and Butylated Hydroxytoluene as Antioxidant Food Additives. Food and Chemical Toxicology 1999, 37, 1027–1038.
- Botterweck, A.A.M.; Verhagen, H.; Goldbohm, R.A.; Kleinjans, J.; Van Den Brandt, P.A. Intake of Butylated Hydroxyanisole and Butylated Hydroxytoluene and Stomach Cancer Risk: Results from Analyses in the Netherlands Cohort Study. Food and Chemical Toxicology 2000, 38, 599–605.
- Sasaki, Y.F.; Kawaguchi, S.; Kamaya, A.; Ohshita, M.; Kabasawa, K.; Iwama, K.; Taniguchi, K.; Tsuda, S. The Comet Assay with 8 Mouse Organs: Results with 39 Currently Used Food Additives. Mutation Research 2002, 519, 103–119.
- Whysner, J.; Wang, C.X.; Zang, E.; Iatropoulos, M.J.; Williams, G.M. Dose Response of Promotion by Butylated Hydroxyanisole in Chemically Initiated Tumours of the Rat Forestomach. Food and Chemical Toxicology 1994, 32, 215–222.
- Djeridane, A.; Yousfi, M.; Nadjemi, B.; Boutassouna, D.; Stocker, P.; Vidal, N. Antioxidant Activity of Some Algerian Medicinal Plant Extracts Containing Phenolic Compounds. Food Chemistry 2006, 97, 654–660.
- Turkmen, N.; Sari, F.; Velioglu, Y.S. Effects of Extraction Solvents on Concentration and Antioxidant Activity of Black and Black Mate Tea Polyphenols Determined by Ferrous Tartrate and Folin–Ciocalteu Methods. Food Chemistry 2006, 99, 835–841.
- Katsube, T.; Tsurunaga, Y.; Sugiyama, M.; Furuno, T.; Yamasaki, Y. Effect of Air-Drying Temperature on Antioxidant Capacity and Stability of Polyphenolic Compounds in Mulberry (Morus alba L.) Leaves. Food Chemistry 2009, 113, 964–969.
- Mrad, N.D.; Boudhrioua, N.; Kechaou, N.; Courtois, F.; Bonazzi, C. Influence of Air-Drying Temperature on Kinetics, Physicochemical Properties, Total Phenolic Content and Ascorbic Acid of Pears. Food and Bioproducts Processing 2012, 90, 433–441.
- Marquez, A.; Serratosa, M.P.; Merida, J. Influence of Bottle Storage Time on Colour, Phenolic Composition and Sensory Properties of Sweet Red Wines. Food Chemistry 2014, 146, 507–514.
- Zafrilla, P.; Morillas, J.; Mulero, J.; Cayuela, J.M.; Marténez-Cachá, A.; Pardo, F.; Nicolás, J.M.L. Changes during Storage in Conventional and Ecological Wine: Phenolic Content and Antioxidant Activity. Journal of Agricultural and Food Chemistry 2003, 51, 4694–4700.
- Singleton, V.L.; Rossi, J.J.A. Colorimetry of Total Phenolics with Phosphomolybdic-Phosphotungstic Acid Reagents. American Journal of Enology and Viticulture 1965, 16, 144–158.
- Blois, M.S. Antioxidant Determinations by the Use of a Stable Free Radical. Nature 1958, 181, 1199–1200.
- Halliwell, B.; Gutteridge, J.M.C. Role of Iron in Oxygen Radical Reactions. Methods in Enzymology 1984, 105, 47–59.
- Nishikimi, M.; Rao, N.A.; Yagi, K. The Occurrence of Superoxide Anion in the Reaction of Reduced Phenazine Methosulfate and Molecular Oxygen. Biochemical and Biophysical Research Communications 1972, 46, 849–854.
- Marcocci, L.; Maguire, J.J.; Groy-Lefaix, M.T.; Packer, L. The Nitric Oxide-Scavenging Properties of Ginko Biloba Extract EGb 761. Biochemical and Biophysical Research Communications 1994, 201, 748–755.
- Oyaizu, M. Studies on Products of Browning Reactions: Antioxidative Activities of Products of Browning Reaction Prepared from Glucosamine. Japanese of Journal of Nutrition and Dietetics 1986, 44, 307.
- Alonso-Salces, R.M.; Korta, E.; Barranco, A.; Berrueta, A.; Gallo, B.; Vicente, F. Determination of Polyphenolic Profiles of Basque Cider Apple Varieties Using Accelerated Solvent Extraction. Journal of Agricultural and Food Chemistry 2001, 49, 3761–3767.
- Escarpa, A.; González, M.C. High-Performance Liquid Chromatography with Diode-Array Detection for the Determination of Phenolic Compounds in Peel and Pulp from Different Apple Varieties. Journal of Chromatography 1998, 823, 331–337.
- Sun, J.; Liang, F.; Bin, Y.; Li, P.; Duan, C. Screening Non-Colored Phenolics in Red Wines Using Liquid Chromatography/Ultraviolet and Mass Spectrometry/Mass Spectrometry Libraries. Molecules 2007, 12, 679–693.
- Alvárez, J.M.; Cuca, L.E.; Carrasco-Pancorbo, A.; Ruiz-Muelle, A.B.; Fernández, I.; Fernández-Gutiérrez, A. Phenolic Constituents of Leaves from Persea caerulea Ruiz & Pav; Mez (Lauraceae). Biochemical Systematics and Ecology 2016, 67, 53–57.
- Horai, H.; Arita, M.; Kanaya, S.; Nihei, Y.; Ikeda, T.; Suwa, K.; Ojima, Y.; Tanaka, K.; Tanaka, S.; Aoshima, K.; Oda, Y.; Kakazu, Y.; Kusano, M.; Tohge, T.; Matsuda, F.; Sawada, Y.; Yokota Hirai, M.; Nakanishi, H.; Ikeda, K.; Akimoto, N.; Maoka, T.; Takahashi, H.; Ara, T.; Sakurai, N.; Suzuki, H.; Shibata, D.; Neumann, S.; Iida, T.; Tanaka, K.; Funatsu, K.; Matsuura, F.; Soga, T.; Taguchi, R.; Saito, K.; Nishioka, T. MassBank: A Public Repository for Sharing Mass Spectral Data for Life Sciences. Journal of Mass Spectrometry 2010, 45, 703–714.
- Uysal, S.; Zengin, G.; Aktumsek, A.; Karatas, S. Chemical and Biological Approaches on Nine Fruit Tree Leaves Collected from the Mediterranean Region of Turkey. Journal of Functional Foods 2016, 22, 518–532.
- Wang, S.Y.; Lin, H.S. Antioxidant Activity in Fruits and Leaves of Blackberry, Raspberry, and Strawberry Varies with Cultivar and Developmental Stage. Journal of Agricultural and Food Chemistry 2000, 48, 140–146.
- Chan, E.W.C.; Lim, Y.Y.; Chew, Y.L. Antioxidant Activity of Camellia sinensis Leaves and Tea from a Lowland Plantation in Malaysia. Food Chemistry 2007, 102, 1214–1222.
- Maillard, M.N.; Berset, C. Evolution of Antioxidant Activity during Kilning: Role of Insoluble Bound Phenolic Acids of Barley and Malt. Journal of Agricultural and Food Chemistry 1995, 43, 1789–1793.
- Que, F.; Mao, L.; Fang, X.; Wu. Comparison of Hot Air-Drying and Freeze-Drying on the Physicochemical Properties and Antioxidant Activities of Pumpkin (Cucurbita moschata Duch.) Flours. International Journal of Food Science and Technology 2008, 43, 1195–1201.
- Vega-Gálvez, A.; Di Scala, K.; Rodríguez, K.; Lemus-Mondaca, R.; Miranda, M.; López, J.; Perez-Won, M. Effect of Air-Drying Temperature on Physico-Chemical Properties, Antioxidant Capacity, Colour and Total Phenolic Content of Red Pepper (Capsicum annuum, L. Var. Hungarian). Food Chemistry 2009, 117, 647–653.
- Recamales, Á.F.; Sayago, A.; González-Miret, M.L.; Hernanz, D. The Effect of Time and Storage Conditions on the Phenolic Composition and Colour of White Wine. Food Research International 2006, 39, 220–229.