ABSTRACT
The delivery of tyrosol by BN-nanotube was investigated by density functional theory in the gas phase. The antioxidant activity of tyrosol was investigated in the gas phase and water. The effects of NHMe, OMe, and tert-butyl substituents on the antioxidant activity and delivery of tyrosol were investigated. Results showed that BN-nanotube interacted with tyrosols effectively and adsorption of tyrosol derivatives on BN-nanotube were exothermic and possible from the energetic viewpoint. Also, replacement of the NHMe group at the x position of tyrosol was suitable scheme the sensitivity of tyrosol toward BN-nanotube surface. The NHMe and OMe substitutions decreased the BDE and IP values and increased the antioxidant activity of tyrosol. The BDE an IP values of tyrosols have linear dependencies with Ead values of tyrosols. These linear dependencies proposed the novel tyrosol derivatives with high level of antioxidant activity.
Introduction
Peroxidation of cell membrane lipid results in membrane destabilization and change of transport characteristics, which leads to cell death, eventually. Many studies have demonstrated that lipid peroxidation by free radicals was most important factor to increase the cancer in human body. Antioxidants protect body cells against the unstable molecules known as free radicals. Tyrosol is a natural phenolic antioxidant in a variety of natural sources such as wines, green tea, and argan oil. [Citation1,Citation2]
Tyrosol is considered for its contribution to flavour, stability, and nutritional value of oil, with also demonstrated bioavailability in humans. In addition, tyrosol has strong antioxidant activity as well as the ability to inhibit pro-oxidation processes on human particles in numerous in vitro experiments.[Citation3,Citation4] Tyrosol as phenolic antioxidant can play its protective role via two mechanisms. In the first mechanism, the free radical removes a hydrogen atom from the tyrosol. In the second mechanism, the tyrosol give an electron to the free radical. The bond dissociation enthalpy (BDE) and ionization potential (IP) are important parameters for evaluation the antioxidant activity of compounds. The antioxidants with high level of activity have lower BDE and IP values and so they can deactivate free radicals by transfer the electron or hydrogen to free radicals, efficiently.[Citation5–Citation9]
Nano-medicine is the medical application of nanotechnology. Nano-medicine ranges from the medical applications of nano-materials and biological devices, to nano-electronic biosensors, and even possible future applications of molecular nanotechnology such as biological machines. The integration of nano-materials with biology has led to the development of diagnostic devices and drug delivery vehicles.[Citation10,Citation11]
Since the discovery of boron nitride (BN)-nanotubes, they have opened up new fields in science and technology because of their unique properties. BN-nanotubes have the bio-compatibility properties and they were suitable nanostructure to drug delivery and other biomedical applications. In previous studies, have been proved that BN-nanotubes were smooth nanostructures channel to transfer the important antioxidant drugs.[Citation12,Citation13]
In the present work, the antioxidant activity of tyrosol and its interaction with BN-nanotube (9, 0) were investigated by using of theoretical methods. The effects of NHMe, OMe, and tert-butyl substituents (structures were shown in ) on the antioxidant activity of tyrosol were investigated. Also the interactions of studied tyrosol derivatives with BN-nanotube (9, 0) were investigated in the gas phase and water (structures were shown in ). In this study, novel tyrosol derivatives with high level of antioxidant activity were proposed.
Figure 2. Complexes of x-substituted tyrosol derivatives (x = NHMe, OMe, and tert-butyl) with BN-nanotube (9, 0).
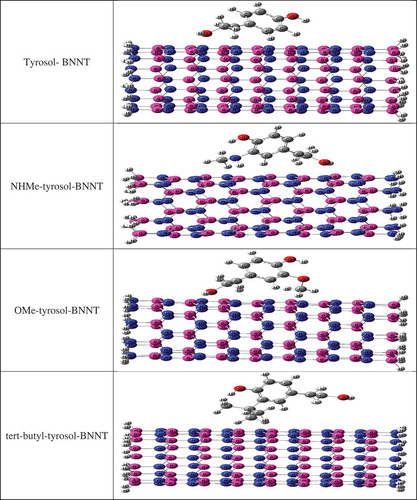
The main objectives of present study are: (1) to investigate the antioxidant activity of tyrosol; (2); to identify the effects of NHMe, OMe, and tert-butyl substituents on BDE and IP values of tyrosol; (3) to find tyrosol derivatives with high level of antioxidant activity and low BDE and IP values; (4) to investigate the ability of BN-nanotube (9, 0) to delivery of tyrosol; (5) to explore how the NHMe, OMe, and tert-butyl substituents alter the tyrosol adsorption on BN-nanotube (9, 0) surface.
Computational details
In this article, geometry structure of substituted tyrosols and their radical and cation radical forms were optimized in the gas phase and water. Also the geometry structures of BN-nanotube (9, 0) and their complexes with tyrosol derivatives were optimized in the gas phase and water. In order to avoid the boundary effects, atoms at the open ends of the studied BN-nanotube (9, 0) structures were saturated with hydrogen atoms. Frequency analyses at the optimized structures were carried out at the same theoretical level which enable us to confirm real minima.[Citation14–Citation16] All the calculations were performed in the GAMESS package by using of the DFT/B3LYP method and 6–311++G (d) basis set in gas phase and polarized continuum model (PCM) in water.[Citation17–Citation19]
Results and discussion
In this study, the BDE and IP values of tyrosol were investigated in the gas phase and water. The effects of NHMe, OMe, and tert-butyl substituents on antioxidant activity of tyrosol were investigated. According to obtained results in this study, the novel tyrosol derivatives with high level of antioxidant activity were proposed. Also the adsorption energy (Ead) of studied tyrosol derivatives on BN-nanotube surface were investigated. In this study, the adsorption energy of studied complexes were defined as follow:
where the E (BN-nanotube/x-tyrosol) corresponds to the energy of x-tyrosol when adsorbed to BN-nanotube, E (BN-nanotube) is the energy of the BN-nanotube, E (x-tyrosol) is the energy of x-tyrosol, and EBSSE is the energy of the basis set superposition error, that was calculated by using the counterpoise correction method.[Citation20] By definition, a negative Ead value corresponds to an exothermic adsorption of x-tyrosol on BN-nanotube surface. In this study, the dependencies of between calculated BDE and IP values of tyrosols and Ead values of tyrosols were investigated.
Computed BDE and IP values of tyrosol derivatives
In recent years, the antioxidant activity of important antioxidant compounds have been investigated.[Citation21–Citation30] In this section, the effects of NHMe, OMe, and tert-butyl substituents on the BDE and IP values of tyrosol were investigated, in order to find the novel tyrosol derivatives with high level of antioxidant activity. This work represents the first systematic study of antioxidant activity of substituted tyrosol derivatives by using of theoretical methods.
The computed BDE and IP values of NHMe, OMe, and tert-butyl substituted tyrosols in the gas phase and water were reported in . The calculated BDE values of tyrosol were 340 and 333 kJ mol−1 in the gas phase and water, respectively. For NHMe-tyrosol the BDE values were lower than those of tyrosol ca 38 and 36 kJ mol–1 in the gas phase and water, respectively.
Table 1. The computed BDE and IP values (kJ mol−1) of tyrosol derivatives in the gas phase and water.
The OMe group in the x position of tyrosol, decrease the BDE value of tyrosol ca 21 and 18 kJ mol−1 in the gas phase and water, respectively. For tert-butyl-tyrosol, the BDE values were lower than those of tyrosol ca 328 and 324 kJ mol−1 in the gas phase and water, respectively. Finally, results show that BDE values of NHMe-tyrosol were lower than those of OMe-tyrosol ca 11 and 32 kJ mol−1 in the gas phase and water, respectively.
The calculated IP values of tyrosol were 670 and 315 kJ mol−1 in the gas phase and water, respectively. The OMe group in x position of tyrosol decrease the IP values of tyrosol 38 and 31 kJ mol−1, in gas phase and water, respectively. The NHMe group in x position of tyrosol decrease the IP values of tyrosol ca 64 and 61 kJ mol−1, in the gas phase and water, respectively. Results show that IP values of NHMe-tyrosol was lower than those of OMe tyrosol ca 28 and 40 kJ mol−1 in gas phase and water, respectively. Results show that BDE and IP values of tyrosol derivatives in water were lower than those of values in gas phase ca 6 and 350 kJ mol−1, respectively. Therefore, replacing of hydrogen atom in x position of tyrosol by NHMe group can suitable scheme to improve the antioxidant activity of tyrosol.
In this article, the BDE and IP values of tyrosol and NHMe, OMe, and tert-butyl substituted tyrosols were calculated in benzene, methanol, and ethanol phases and calculated results were reported in . Results show that BDE values of tyrosol were 343, 338, and 339 kJ mol−1 in benzene, methanol, and ethanol phases, respectively. The IP values of tyrosol were 677, 680, and 681 kJ mol−1 in benzene, methanol, and ethanol phases, respectively.
Table 2. The computed BDE and IP values (kJ mol−1) of tyrosol derivatives in the benzene, ethanol, and methanol phase.
The BDE and IP values of substituted tyrosol derivatives in benzene were higher than values of substituted tyrosol derivatives in water ca 8 and 360 kJ mol−1, respectively. The BDE and IP values of substituted tyrosol derivatives in methanol and ethanol were higher than corresponding values of substituted tyrosol derivatives in water ca 6 and 365 kJ mol−1, respectively. Therefore results show that, calculated BDE and IP values of substituted tyrosol derivatives in water were lower than corresponding values of substituted tyrosol derivatives in benzene, methanol, and ethanol phases.
Calculated and experimental ∆BDE and ∆IP values of substituted phenols were compiled in . The experimental BDE values were obtained by using of the electro chemical (EC) method. The experimental IP values were obtained by using of the electron impact technique (EIT) method. [Citation5,Citation6,Citation21,Citation28] The average absolute deviations of the ∆BDE values of tyrosols and those of phenols in were 2 and 6 kJ mol−1, respectively.
Table 3. The obtained ∆BDE and ∆IP values of substituted phenols (kJ mol–1) via experimental and theoretical methods.
The average absolute deviations of the ∆IP values of substituted tyrosols and those of phenols in were 3 and 5 kJ mol−1, respectively. Therefore, results show that, published ∆BDE and ∆IP values of phenols [Citation5,Citation6,Citation21,Citation28] and ∆BDE and ∆IP values of tyrosols in present article have same trends.
Adsorption of tyrosol derivatives on BN-nanotube surface
The calculated Ead values of x-substituted tyrosol derivatives on BN-nanotube surface in the gas phase and water were reported in the . Results reveal that Ead values of x-tyrosol derivatives on BN-nanotube surface were negative. Results show that, the Ead values of tyrosol on BN-nanotube in the gas phase and water were −6.18 and −5.96 kJ mol−1, respectively. The Ead values of tert-butyl-tyrosol on BN-nanotube in the gas phase and water were −6.38 and −6.12 kJ mol−1, respectively. Results indicated that, NHMe and OMe substituents increase the absolute values of Ead of tyrosol ca 0.55 and 0.38 kJ mol−1 in gas phase, respectively. Also, the NHMe and OMe substituents increase the absolute values of Ead of tyrosol ca 0.61 and 0.37 kJ mol−1 in water, respectively. The absolute values of Ead of tyrosol on BN-nanotube in water were lower than corresponding values in gas phase ca 0.24 kJ mol−1.
Table 4. The calculated Ead values (kJ mol−1) of tyrosol derivatives on BN-nanotube (9, 0) surface, and the charge transferred (QT (e) in eV) from the tyrosol derivatives to BN-nanotube (9, 0).
The NHMe and OMe substituents have the high delocalization energy or high resonance energy and these can stabilize the tyrosol derivatives and their complexes with BN-nanotube remarkable, in the gas phase and water. Therefore, the NHMe and OMe tyrosol derivatives have more negative Ead values and higher ability to adsorption on surface of BN-nanotube in comparison to tyrosol. In according to obtained Ead values, it can be concluded that NHMe-tyrosol derivative has highest ability to adsorption on surface of BN-nanotube.
In this paper, Also the transferred charge (QT (e)) from the tyrosol derivatives to BN-nanotube (9, 0) were calculated and obtained results were reported in . Results show that, value of transferred charge from tyrosol to BN-nanotube (9, 0) was 0.132 eV. Also, the values of transferred charge from NHMe-tyrosol and OMe-tyrosol to BN-nanotube (9, 0) were 0.178 and 0.143 eV, respectively. The scheme of density of state of complexes of tyrosol derivatives with BN-nanotube (9, 0) were reported in . Results in indicated that NHMe-tyrosol has significant adsorption energies and charge transfer and therefore, NHMe-tyrosol can be chemisorbed on the surface of the BN-nanotube (9, 0).
In present paper, the dependencies between Ead, BDE, and IP values of tyrosol derivatives were investigated. The absolute values of Ead of tyrosol derivatives were corrected against calculated BDE and IP values. The Equations 2–5 were obtained from linear regressions as follow:
The correlation coefficients of Equations 2–5 were 0.993, 0.994, 0.989, and 0.985, respectively. These results show that, there were linear dependencies between Ead, BDE, and IP values of studied tyrosol derivatives. Obtained equations enable us to estimate the Ead values of studied tyrosol derivatives from corresponding BDE and IP values. According to obtained results, novel tyrosol derivatives with high level of antioxidant activity were proposed.
In this article, the effects of size of BN-nanotubes on adsorption potential of tyrosol derivatives were investigated. The calculated Ead values of tyrosol derivatives on various BN-nanotube surfaces were reported in the . Results show that, BN-nanotube (10, 0) has higher potential to tyrosol adsorption rather than BN-nanotube (9, 0) ca 0.12 kJ mol−1. Also, results show that BN-nanotube (8, 0) and BN-nanotube (7, 0) have lower potential to tyrosol adsorption rather than BN-nanotube (9, 0) ca 0.04 and 0.06 kJ mol−1, respectively.
Table 5. The calculated Ead values (kJ mol−1) of tyrosol derivatives on BN-nanotube (10, 0), BN-nanotube (8, 0), and BN-nanotube (7, 0) surfaces.
Conclusion
In this study, the BDE and IP values of tyrosol were calculated in gas phase and water. The effects of NHMe, OMe, and tert-butyl substituents on the antioxidant activity of tyrosol were investigated. According to obtained results, novel tyrosol derivatives with high level of antioxidant activity were proposed. Also the adsorption energy (Ead) of studied tyrosol derivatives on BN-nanotube surface were investigated. Results show that, BN-nanotube interacted with substituted tyrosols effectively and so their adsorptions were exothermic and possible from the energetic viewpoint. Results show that, replacement of the NHMe group at the x position of tyrosol was suitable scheme to improve the sensitivity of tyrosol toward BN-nanotube surface. The NHMe and OMe substitutions increase antioxidant activity of tyrosol. The calculated BDE an IP values of the substituted tyrosols have linear dependencies with Ead values of tyrosols. These linear dependencies, selected the novel tyrosol derivatives with high level of antioxidant activity.
Acknowledgment
I would like to thank my colleagues for their valuable discussion on the computational affairs.
References
- Bolton, J. L.; Trush, M. A.; Penning, T. M.; Dryhurst, G.; Monks, T. J. Role of Quinones in Toxicology. Chem. Res. Toxicol. 2000, 13, 135–160. DOI: 10.1021/tx9902082.
- Buege, J. A.; Aust, S. D. Microsomal Lipid Peroxidation. Meth. Enzymol. 1978, 52, 302–310.
- Cao, G.; Alessio, H. M.; Culter, R. G. Oxygen Radical Absorbance Capacity Assay for Antioxidant Free Radicals. Biol. Med. 1993, 14, 303–311.
- Chanda, S.; Dave, R. In Vitro Models for Antioxidant Activity Evaluation and Some Medicinal Plants Possessing Antioxidant Properties: An Overview. Afr. J. Microbiol. Res. 2009, 3, 981–996.
- Wright, J. S.; Johnson, E. R.; Dilabio, G. A. Predicting the Activity of Phenolic Antioxidants: Theoretical Method, Analysis of Substituent Effects, and Application to Major Families of Antioxidants. J. Am. Chem. Soc. 2001, 123, 1173–1183. DOI: 10.1021/ja002455u.
- Zhang, H. Y.; Ji, H. F. S–H Proton Dissociation Enthalpies of Thiophenolic Cation Radicals: A DFT Study. J. Mol. Structure: THEOCHEM. 2003, 663, 167–174. DOI: 10.1016/j.theochem.2003.08.124.
- Foti, M. C.; Daquino, C.; Geraci, C. Electron–Transfer Reaction of Cinnamic Acids and Their Methyl Esters with the DPPH• Radical in Alcoholic Solutions. J. Org. Chem. 2004, 69, 2309–2314. DOI: 10.1021/jo035758q.
- Litwinienko, G.; Ingold, K. U. Abnormal Solvent Effects on Hydrogen Atom Abstraction. 3. Novel Kinetics in Sequential Proton Loss Electron Transfer Chemistry. J. Org. Chem. 2005, 70, 8982–8990. DOI: 10.1021/jo051474p.
- Wang, L. F.; Zhang, H. Y. A Theoretical Study of the Different Radical–Scavenging Activities of Catechin, Quercetin, and A Rationally Designed Planar Catechin. Bioorg. Chem. 2005, 33, 108–115. DOI: 10.1016/j.bioorg.2005.01.002.
- Machado, M.; Mota, R.; Piquini, P. Electronic Properties of BN Nanocones under Electric fields. Microelectron J. 2003, 34, 545–547. DOI: 10.1016/S0026-2692(03)00044-2.
- Halpern, J. B.; Bello, A.; Gilcrease, J.; Harris, G. L.; He, M. Biphasic GaN Nanowires: Growth Mechanism and Properties. Microelectron J. 2009, 40, 316–318. DOI: 10.1016/j.mejo.2008.07.022.
- Beheshtian, J.; Kamfiroozi, M.; Bagheri, Z.; Ahmadi, A. B12N12 Nanocage as Potential Sensor for NO2 Detection. Chin. J. Chem. Phys. 2012, 25, 60–64. DOI: 10.1088/1674-0068/25/01/60-64.
- Ahmadi, A.; Beheshtian, J.; Kamfiroozi, M. Benchmarking of ONIOM Method for the Study of NH3 Dissociation at Open Ends of BNNTs. J. Mol. Model. 2012, 18, 1729–1734. DOI: 10.1007/s00894-011-1202-5.
- Dinadayalane, T. C.; Murray, J. S.; Concha, M. C.; Politzer, P.; Leszczynski, J. Reactivities of Sites on (5,5) Single-Walled Carbon Nanotubes with and without a Stone–Wales Defect. J. Chem. Theory Computational. 2010, 6, 1351–1357. DOI: 10.1021/ct900669t.
- Schmidt, M.; Baldridge, K.; Boatz, J.; Elbert, S.; Gordon, M.; Jensen, J.; Koseki, S.; Matsunaga, N.; Nguyen, K.; Su, S.; Windus, T.; Dupuis, M. General Atomic and Molecular Electronic Structure System. J. Comput. Chem. 1993, 14, 1347–1363. DOI: 10.1002/jcc.540141112.
- Grimme, S. Accurate Description of Van Der Waals Complexes by Density Functional Theory Including Empirical Corrections. J. Comput. Chem. 2004, 25, 1463–1471. DOI: 10.1002/jcc.20078.
- Andzelm, J.; Kolmel, C. Incorporation of Solvent Effects into Density Functional Calculations of Molecular Energies and Geometries. J. Chem. Phys. 1995, 103, 9312–9320. DOI: 10.1063/1.469990.
- Gan, L. H.; Zhao, J. Q. Theoretical Investigation of [5, 5, 9, 0] and [10, 10] Closed WCNTs. Physica E (Low-Dimensional Systems and Nanostructures). 2009, 41, 1249–1252. DOI: 10.1016/j.physe.2009.02.014.
- Beheshtian, J.; Peyghan, A. A.; Bagheri, Z. Adsorption and Dissociation of Cl2 Molecule on ZnO Nanocluster. Appl. Surface. Sci. 2012, 258, 8171–8176. DOI: 10.1016/j.apsusc.2012.05.016.
- Boys, S. F.; Bernardi, F. The Calculation of Small Molecular Interactions by the differences of Separate Total Energies. Some Procedures with Reduced Errors. Mol. Phys. 1970, 19, 553–566. DOI: 10.1080/00268977000101561.
- Zagmutt, S.; Guzmán, L.; Orrego, R.; Wehinger, S.; Leiva, E. Phenolic Compound Identification and Antioxidant Capacity of Alperujo Extracts from Region del Maule, Chile. Int. J. Food Properties. 2016, 19, 2016–2025. DOI: 10.1080/10942912.2015.1092162.
- Qin, Y.; Sun, Y.; Li, J.; Xie, R.; Deng, Z.; Chen, H.; Li, H. Characterization and Antioxidant Activities of Procyanidins from Lotus Seedpod, Mangosteen Pericarp, and Camellia Flower. Int. J. Food Properties. 2017, 20, 1621–1632. DOI: 10.1080/10942912.2016.1215997.
- Rezaei, F.; Jamei, R.; Heidari, R.; Maleki, R. Chemical Composition and Antioxidant Activity of Oil from Wild Achillea Setacea and A. Vermicularis. Int. J. Food Properties. 2017, 20, 1522–1531. DOI: 10.1080/10942912.2016.1213281.
- Vieira, E. F.; Ferreira, I. O. Antioxidant and Antihypertensive Hydrolysates Obtained from By-Products of Cannery Sardine and Brewing Industries. Int. J. Food Properties. 2017, 20, 662–673. DOI: 10.1080/10942912.2016.1176036.
- López-Vidaña, E. C.; Figueroa, I. P.; Cortés, F. B.; Rojano, B. A. Effect of Temperature on Antioxidant Capacity during Drying Process of Mortiño (Vaccinium Meridionale Swartz). Int. J. Food Properties. 2017, 20, 294–305. DOI: 10.1080/10942912.2016.1155601.
- Zhang, Y.; Lu, R.; Song, H.; Zheng, S.; Chen, Y.; Zhou, N. Effect of Stir Frying on the Antioxidant Capacity and Aroma Components of Bok Choy (Chinese Cabbage). Int. J. Food Properties. 2016, 19, 2536–2549. DOI: 10.1080/10942912.2015.1118385.
- Djahaniani, H.; Nasrabadi, M.; Saiedpour, M.; Nazarian, S.; Ganjali, M.; Batooli, H. Facile Synthesis of Silver Nanoparticles Using Tribulus Longipetalus Extract and Their Antioxidant and Antibacterial Activities. Int. J. Food Properties. 2016, 19, 922–930.
- Ran, J.; Sun, H.; Xu, Y.; Wang, T.; Zhao, R. Comparison of Antioxidant Activities and High-Performance Liquid Chromatography Analysis of Polyphenol from Different Apple Varieties. Int. J. Food Properties. 2016, 19, 2396–2407. DOI: 10.1080/10942912.2015.1037958.
- Song, W.; Zhu, X. F.; Ding, X. D.; Yang, H. B.; Qin, S.; Chen, H.; Wei, S. Structural Features, Antioxidant and Tyrosinase Inhibitory Activities of Proanthocyanidins in Leaves of Two Tea Cultivars. Int. J. Food Properties. 2017, 20, 1348–1358. DOI: 10.1080/10942912.2016.1209682.
- Wu, L.; Jiang, A.; Jing, Y.; Zheng, Y.; Yan, Y. Antioxidant Properties of Protein Hydrolysate from Douchi by Membrane Ultrafiltration. Int. J. Food Properties. 2017, 20, 997–1006. DOI: 10.1080/10942912.2016.1192644.