ABSTRACT
Caprine whey protein concentrates (WPC) were incorporated as emulsifiers in a salad dressing. The concentrates were manufactured by ultrafiltration-diafiltration with or without previous clarification by thermocalcic precipitation. Aggregates obtained in the clarification treatment and a commercial bovine WPC were also used as emulsifiers. Good emulsifying properties of caprine products were observed. Dressings made with caprine products showed higher firmness and stability than dressings made with bovine WPC. Thermocalcic precipitation improved the properties of caprine WPC. Aggregates modified the colour of dressings contributing to a more yellowish aspect. Microstructure of emulsions was affected by the type of protein.
Introduction
Food dressings are oil in water emulsions and are often used for seasoning food and enhancing its taste, colour, or aroma. Emulsifiers are essential for emulsion formation and stabilisation.[Citation1,Citation2] Among the emulsifiers commonly used in the industry, whey proteins play an important role as effective emulsifiers in food products.[Citation3] However, most of the whey proteins concentrates (WPC), typical dried whey products, are derived from bovine whey. In recent years, people are increasingly concerned about nutrition and look for healthy and allergen-free food products. Caprine milk proteins have been considered a potential substitute for bovine milk proteins due to their hypoallergenic and nutritional value.[Citation4] Therefore, caprine WPC could be interesting ingredients in the formulation of new food products.
The functional properties of clarified and unclarified caprine WPC and aggregates (clarification by-products) have been studied by Sanmartín et al.[Citation5] The results showed that these products could become suitable candidates for food formulations that require good emulsifying properties, although research is needed in order to evaluate them in complex mixtures with other ingredients.
Little information on the application of caprine WPC as emulsifiers in a real food product has been found. Palatnik et al.[Citation6] applied a caprine whey protein concentrate (23.7% protein) in the formulation of a dressing. The emulsion was made with a protein gel, and starch was also added as stabiliser, probably due to the low protein content of the concentrate. No studies on the application of caprine WPC of high protein content as exclusive emulsifiers in dressings have been reported.
The aim of this work was the application of three caprine products (unclarified and clarified WPC, and aggregates) as emulsifiers in the formulation of a salad dressing and to compare the rheological properties, colour, stability, and microstructure of these dressings with those of a salad dressing made with a commercial bovine WPC.
Materials and methods
Sample preparation
Sweet caprine whey was obtained from a cheese-making farm which produced rennet-coagulated cheese with pasteurised whole milk. The curd fines were separated from the whey using a filter (20 µm of pore diameter) and then, the whey was pasteurised at 63°C for 30 min (initial whey). Approximately 80 L of whey were used in each trial. 50 L were clarified by thermocalcic precipitation followed by separation of aggregates by centrifugation. The clarified whey (40 L), obtained after separation of aggregates, and the initial whey (30 L) were ultrafiltrated followed by diafiltration using a Centramate lab tangencial flow system equipped with an Omega (polyethersulfone) membrane cassette (0.09 m2 surface area, 10 kDa MW cut-off) (Pall Corporation, Ann Arbor, MI, USA). The diafiltration retentates and the aggregates were freeze dried in a Lyph-LockTM (Labconco Corporation, Kansas City, USA) freeze dryer. Three products were obtained: the aggregate powder (aggregates) (9.4% protein), the diafiltration retentate powder from the clarified whey (CWP) (74.1% protein), and the diafiltration retentate powder from the unclarified whey (UWP) (36.8% protein). More details and a diagram of the process can be found in Sanmartín et al.[Citation7] Detailed chemical, lipid, and whey protein compositions of the three types of caprine products and a commercial bovine WPC (74.7% protein) [Protarmor 800, Armor Proteins (Saint Brices en Coglés, France)] are described in Sanmartín et al.[Citation5,Citation7]
Salad dressing preparation
Salad dressings were prepared based on the method described by Turgeon et al.[Citation2] Salad dressings contained 62.0% refined sunflower oil, 5.0% vinegar, 2.5% sugar, 1.5% salt, 0.7% onion, and 0.8% garlic. Caprine or bovine products (CWP, UWP, bovine WPC and aggregates) were incorporated in order to obtain two incorporation levels [0.5 and 1.0% (w/w) protein]. The mixture was completed with water. The dressings were prepared using a Polytron PT-20 homogeneiser (Brikman Instruments, Westbury, USA) at ambient temperature. The ingredients were added into the aqueous phase (water and vinegar) and were stirred at 11,000 rpm for 30 s. Finally, oil was added into the mixture and homogenised at 11,000 rpm for 3 min. The pH of the salad dressings was determined: 3.7 for CWP, 3.8 for UWP, 5.0 for aggregates and 4.0 for bovine WPC. All dressings were made in duplicate.
Rheological properties
Oscillatory rheological measurements of dressings were determined using a stress-controlled rheometer (Haake RS100, Thermo Electron, Karlsruhe, Germany) fitted with a cone-and-plate geometry (C35/4). Prior to frequency sweeps, the linear viscoelastic region was determined performing stress sweeps at a frequency of 0.1 Hz. Dressings were subjected to a frequency sweep from 0.147 to 14.7 Hz at 0.5 Pa in the linear viscoelastic range. Complex (G*), elastic (Gʹ) and viscous (Gʺ) moduli were recorded. The rheological evaluation was carried out after overnight cold storage at 4°C. All measurements were made in triplicate for each dressing at ambient temperature.
Dressing stability
Short and long-term dressing stabilities were evaluated by two different methods. Short-term stability was studied according to Christiansen et al.[Citation3] After preparation of dressings, five aliquots were separated: three aliquots of 25 g were used to evaluate the stability after elaboration (day 0), and on the next day after holding overnight at 4°C and at ambient temperature (day 1). The emulsions were centrifuged at 2000 g for 15 min at 20°C.
Long-term stability was studied in accordance with Turgeon et al.[Citation2] Two aliquots of 10 ml were stored for 6 months at 4°C and at ambient temperature. Emulsion stability was determined by weighing the dressing before and after the separation of the aqueous and oil phase from the emulsion, using the equation: Storage stability (%) = [weight (g) of dressing after liquid separation/weight (g) of initial dressing] * 100. All measurements were carried out in duplicate for each dressing.
Colour
Colour of the dressings was measured using a chromameter X-Rite model SP60 (X-Rite, Grand Rapids, MI, USA). All determinations were made in the CIE L*a*b* colour space using the D65 illuminant and 10º standard observer. The instrument was standardised with the white and black tiles provided by the manufacturer before sample measurements. The colour values were expressed as L* (lightness), a* (red/greenness) and b* (yellow/blueness). Colour was evaluated after an overnight cold storage at 4°C. The measurements were made in duplicate at ambient temperature.
Scanning electron microscopy
Microstructure of salad dressings was studied by scanning electron microscopy according to the method described by Egelandsdal et al.[Citation8] with some modifications. Dressing samples were encapsulated in 2% agar and fixed in 2.5% glutaraldehyde in 0.1M cacodylate buffer. Afterwards, they were postfixed overnight in 1% OsO4 solution, dehydrated in a graded series of ethanol and dried at critical point with CO2 in a critical point drier (Baltec, model CDP 030, Balzers, Liechtenstein). The agar capsules were opened and the samples were covered with Au–Pd alloy with a Sputter Coater (Baltec, model SCD 005, Balzers, Liechtenstein). The microstructure of salad dressings was observed using a JEOL-JSM 6360LV scanning electron microscope (Jeol, Ltd., Tokyo, Japan) operated at an accelerating voltage of 15 kV.
Statistical analysis
The data were analysed by one-way ANOVA, and the means were compared using the least significant difference test with significance at p < 0.05 (IBM SPSS Statistics for Windows version 20.0, 2011, IBM Corp., Armonk, NY, USA).
Results and discussion
Rheological properties
shows the variation of Gʹ and Gʺ according to the frequency of the salad dressings made with 1.0% (w/w) protein incorporation of CWP, UWP, bovine WPC and aggregates. Gʹ was always higher than Gʺ over the entire frequency range. Salad dressings also showed a moderate influence of the frequency on the values of Gʹ and Gʺ. These results were also found in bovine WPC dressings.[Citation2,Citation3] This pattern is a typical behaviour in most dressings and sauces.[Citation1]
Figure 1. Elastic modulus (Gʹ) and viscous modulus (Gʺ) as a function of frequency of salad dressings made with 1.0% (w/w) protein incorporation.
CWP (♦); UWP (■); Aggregates (○); Bovine WPC (▲).
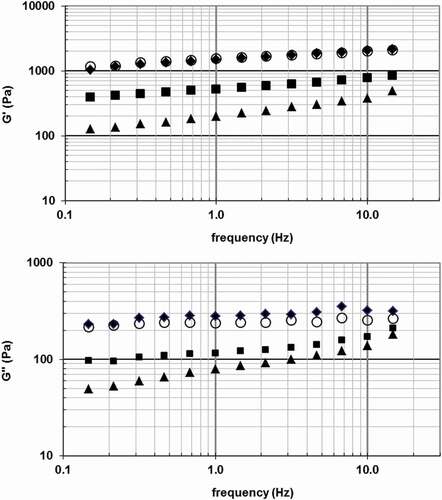
Figure 2. Microstructure of salad dressings added of whey protein products. 1: bovine whey protein concentrate; 2: aggregates powder; 3: unclarified caprine whey powder; 4: clarified caprine whey powder. Images a: product at 0.5% protein; images b: product at 1% protein. Images a and b: magnification of 1000x; image 4c: magnification of 4000x.
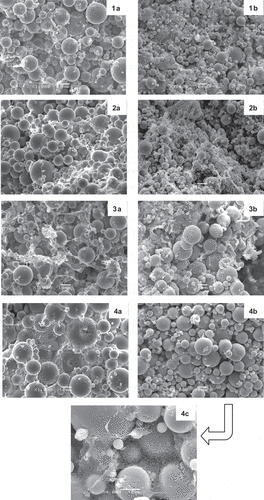
The values of G*, Gʹ, and Gʺ of all salad dressings made with CWP, UWP, aggregates, and bovine WPC at the frequency of 1Hz are shown in . Rheological moduli values of caprine samples were similar to those described by Turgeon et al.[Citation2] in heat treated or hydrolysed bovine WPC dressings and higher than those observed by Christiansen et al.[Citation3] in WPC emulsions. The results showed that values of G*, Gʹ, and Gʺ were influenced by the type of caprine WPC applied to emulsify the dressing. CWP provided significantly firmer salad dressings (higher values of G*, Gʹ, and Gʺ) than UWP at the two levels of protein incorporation.
Table 1. Rheological properties [G*, Gʹ, and Gʺ] of salad dressings made with caprine cheese whey powders and bovine whey protein concentrate.
The strength of caprine dressings is significantly higher than those observed in bovine WPC products at the two levels of protein concentration. The interaction between proteins could be more intense in caprine salad dressings as it has been reported in heat-induced gels at pH 4 by Sanmartín et al.[Citation9]
A good emulsifier effect was also showed by aggregates, providing salad dressings with higher values of G*, Gʹ, and Gʺ than bovine WPC. The results of this study confirmed the good emulsifying properties of clarified by-products in model emulsions at pH 4.[Citation5] The fat globule membrane proteins obtained by precipitation during thermocalcic aggregation could be the compounds responsible of the good emulsifying properties of aggregates.[Citation5,Citation10] High Gʹ values of salad dressings made with aggregates are especially remarkable at 1.0% protein incorporation level. At this level, salad dressings showed significantly higher values of G*, Gʹ, and Gʺ than those made with UWP.
Salad dressings with 1.0% protein showed higher values of G*, Gʹ, and Gʺ than dressings with 0.5% protein. Similar protein concentration effects were observed in egg yolk dressings.[Citation11] This increase could be related to the fact that the emulsifying capacity is improved by protein concentration.[Citation12] Yamauchi et al.[Citation13] reported that the compact globular structure of whey proteins prevents their effective adsorption, and so, higher concentration would be necessary to obtain better emulsifying properties. The presence of more proteins could have helped to create more proteins interactions and could have contributed to increase the values of Gʹ and G”.
Stability
shows the short- (day 0 and day 1) and long-term stability (after 6 months) at ambient and refrigerated storage of the salad dressings prepared with CWP, UWP, aggregates, and bovine WPC. The short-term stability was higher than to 80% for all dressings. Christiansen et al.[Citation3] observed similar values of stability for bovine WPC salad dressings. The dressings also showed very good long-term stability: 90–96% and 88–100% for 0.5 and 1.0% protein, respectively. So, salad dressings were quite stable and showed excellent short and long-term stability with only 0.5 and 1.0% of protein concentration. These results are in agreement with those of Foley and O´Connell,[Citation14] which reported that even small amounts of whey protein are efficient enough to create stable emulsions. Similar results were observed in the stability of model emulsions with caprine products.[Citation5] No other previous studies have been reported on dressing stability of caprine WPC. Turgeon et al.[Citation2] observed that salad dressing made with bovine WPC (35% protein) were unstable (<4 days) regardless of incorporation level (0.5–1.5%) and storage temperature (4 or 25°C) and required higher concentration (1.5%) of heat-treated WPC to improve their stability.
Table 2. Storage stability (%) of salad dressings made with caprine cheese whey powders and bovine whey protein concentrate after different periods and temperature of storage.
No significant differences in short-term stability (days 0 and 1) between UWP and CWP dressings at 0.5 and 1.0% of protein were observed. This is in agreement with the results observed for emulsifying stability of caprine WPC in model emulsions at pH 4.[Citation5] Neither the emulsifying stability rating (ESR) at pH 4 in model emulsions nor the salad dressings short-term stability were improved by the clarification treatment. However, CWP dressings showed better long-term stability than UWP dressings. These results could be related to the viscoelastic properties of the samples; high viscosity of salad dressings improves their storage stability.[Citation2] As it has been reported before, CWP provided significantly firmer salad dressings (higher values of G*, Gʹ, and Gʺ) than UWP, and so, CWP samples were more stable after 6 months of storage. The increase of the interaction among protein molecules in CWP, which contained lower amounts of other compounds, might also be responsible for this improve in long-term stability.
Dressings with caprine aggregates also showed good stability. In model emulsions at pH 4, Sanmartín et al.[Citation5] reported that aggregates showed significant higher ESR values than CWP and UWP at pH 4. The stability of salad dressings made with aggregates was superior to caprine WPC at 1.0% of protein incorporation (except at 6 months compared to CWP which were 100% in both products).
The stability values of dressings obtained by adding caprine WPC or aggregates were higher than those obtained in dressings made with bovine WPC. These results confirmed the good emulsifying properties of caprine products previously reported by Sanmartín et al.,[Citation5] where caprine WPC and aggregates emulsions showed better stability values (ESR) than commercial bovine WPC emulsions at pH 4 and 7. These results for bovine WPC were even worse at pH 4, which corresponds with the pH of the dressings of this work. Sanmartín et al.[Citation5] indicated that the lower solubility of bovine WPC could explain the differences in emulsifying stability between caprine and bovine WPC due to emulsion stability has been positively correlated with protein solubility.[Citation15]
Salad dressings made with 1.0% (w/w) protein incorporation showed higher stability (short- and long-term) than dressings prepared with 0.5% protein, and this fact is especially remarkable for the long-term stability, where 100% values were found for CWP and aggregates dressings. Other authors[Citation12,Citation13,Citation15] have also reported that enhanced stability is expected with higher protein content. Higher protein content could be beneficial to create more proteins interactions and form a protective and resistant layer around oil droplets against coalescence and aggregation.
Salad dressings showed similar storage stability behaviour at 4°C and 25°C with higher values in those samples maintained under refrigeration. Turgeon et al.[Citation2] also observed similar stability behaviour at both temperatures for bovine WPC salad dressings with higher values for the products storage at 4°C. They indicated that refrigerated storage improved the stability due to the increasing of the viscosity as a consequence of stronger solvent-protein interactions and protein-protein interactions between adjacent globules.
Colour
The values of colour parameters (L*, a*, and b*) of the salad dressings made with caprine WPC, aggregates, and bovine WPC are shown in . No information about colour of salad dressings made with WPC has been found. L* and b* values of caprine salad dressings were lower than those described by Palatnik et al.[Citation6] in caprine WPC dressings. These dressings were not a salad dressing and so, the manufacturing process and the composition were different.
Table 3. Colour parameters (CIE L*a*b*) of salad dressings made with caprine cheese whey powders and bovine whey protein concentrate.
Some differences in colour parameters among the salad dressings were found. Samples with caprine products showed higher values of L* than those observed in samples with bovine WPC at both protein contents. These differences in L* values could be related to the strength of salad dressings. Caprine dressings also showed higher values of values of G*, Gʹ, and Gʺ than bovine dressings. Ju and Kilara[Citation16] suggested that L* values are proportional to the light reflected by the product; so, the differences in L* between dressings could be attributed to the structure. High values are correlated with a better structured network in the dressing. Salad dressings made with 1.0% protein also showed higher values of L* than 0.5% salad dressings, and this can also be related with the higher strength of 1% salad dressings.
The colour parameters of aggregates dressings were different than those observed in caprine and bovine WPC dressings. Aggregates salad dressings showed significant higher values of b* than those observed in the other salad dressings. This fact corresponded with a yellowish aspect, whereas the other dressings showed a white appearance. The higher values of b* observed in aggregates could be due to the highest lipid content of the salad dressings made with these clarified by-products. It was necessary to add more proportion of aggregates (with high lipid content) in order to obtain dressings with the same protein concentration (0.5 or 1.0% protein).
Microstructure
SEM micrographs of salad dressings made with caprine WPC, aggregates, and bovine WPC are shown in . A typical emulsion microstructure appeared in all cases, with oil droplets covered by a rough protein layer that acted as emulsifier; similar structures were found by Tippetts et al.[Citation17] in single-layer emulsions at acid pH. However, some differences related to oil droplet size and protein aggregation can be observed among samples. Droplet size was higher when the lowest protein concentration was used in salad dressing formulation (, images 1a-4a), and this observation can be correlated to a lower emulsion stability (). In the samples with 1% protein, oil droplets were smaller and appeared embedded in denser protein networks (, images 1b-4b), due to the increase of protein molecules available not only for interface orientation as emulsifiers but also for the formation of protein-protein interactions in the water phase. Nevertheless, the protein structures showed important differences depending on the protein product. In samples with bovine WPC (, ), proteins that did not act as emulsifiers formed thin strands and small aggregates. These structures were thicker and bigger when caprine aggregates and UWP (, and ) were used in dressing preparation, showing a disordered aspect probably due to the presence of high quantities of other whey compounds such as caseinomacropeptide (in aggregates) or lipids (in both products) that could interfere protein network structure. When CWP was used, oil droplets appeared connected by a thin, well-organised protein network, and protein aggregates were small. A more detailed image (4000x magnifications) of this network can be observed in , .
Although droplet size has been inversely related to firmness and stability of emulsions,[Citation18] CWP salad dressings showed high rheological values and good stability that could be attributed to the structure of the network and the strong interactions among the caprine protein molecules that acted as emulsifiers and the proteins of the continuous phase.
Conclusion
The salad dressing made with the addition of caprine whey protein concentrates or aggregates shows higher firmness and better short- and long-term stability than the dressings manufactured with the addition of bovine whey protein concentrate. Clarification by thermocalcic precipitation increases the firmness of salad dressings made with caprine whey protein concentrates. These results suggest that caprine whey protein and clarified by-products could be considered very useful emulsifiers for food products and could be converted into high added value ingredients for food industry.
Additional information
Funding
References
- Ford, L. D.; Borwankar, R. P.; Pechak, D.; Schwimmer, B. Dressings and Sauces. In Food Emulsions, Friberg, S. E., Larsson, K., Sjoblom, J., Eds.; Marcel Dekker Inc.: New York, 2004; pp 525–572.
- Turgeon, S. L.; Sanchez, C.; Gauthier, S. F.; Paquin, P. Stability and Rheological Properties of Salad Dressing Containing Peptidic Fractions of Whey Proteins. International Dairy Journal. 1996, 6, 645–658. DOI: 10.1016/0958-6946(95)00054-2.
- Christiansen, K. F.; Vegarud, G.; Langsrud, T.; Ellekjaer, M. R.; Egelandsdal, B. Hydrolyzed Whey Proteins as Emulsifiers and Stabilizers in High-Pressure Processed Dressings. Food Hydrocolloids. 2004, 18, 757–767. DOI: 10.1016/j.foodhyd.2003.12.002.
- Haenlein, G. F. W. Goat Milk in Human Nutrition. Small Ruminant Research. 2004, 51, 155–163. DOI: 10.1016/j.smallrumres.2003.08.010.
- Sanmartín, B.; Díaz, O.; Rodríguez-Turienzo, L.; Cobos, A. Functional Properties of Caprine Whey Protein Concentrates Obtained from Clarified Cheese Whey. Small Ruminant Research. 2013, 110, 52–56. DOI: 10.1016/j.smallrumres.2012.11.029.
- Palatnik, D. R.; Porcel, M. V. O.; González, U.; Zaritzky, N.; Campderrós, M. E. Recovery of Caprine Whey Protein and Its Application in a Food Protein Formulation. LWT-Food Science and Technology. 2015, 63, 331–338. DOI: 10.1016/j.lwt.2015.03.027.
- Sanmartín, B.; Díaz, O.; Rodríguez-Turienzo, L.; Cobos, A. Composition of Caprine Whey Protein Concentrates Produced by Membrane Technology after Clarification of Cheese Whey. Small Ruminant Research. 2012, 105, 186–192. DOI: 10.1016/j.smallrumres.2011.11.020.
- Egelandsdal, B.; Christiansen, K. F.; Host, V.; Lundby, F.; Wold, J. P.; Kvaal, K. Evaluation of Scanning Electron Microscopy Images of a Model Dressing Using Image Feature Extraction Techniques and Principal Component Analysis. Scanning. 1999, 21, 316–325. DOI: 10.1002/sca.4950210505.
- Sanmartín, B.; Díaz, O.; Rodríguez-Turienzo, L.; Cobos, A. Properties of Heat-Induced Gels of Caprine Whey Protein Concentrates Obtained from Clarified Cheese Whey. Small Ruminant Research. 2015, 123, 142–148. DOI: 10.1016/j.smallrumres.2014.10.014.
- Díaz, O.; Pereira, C. D.; Cobos, A. Functional Properties of Ovine Whey Protein Concentrates Produced by Membrane Technology after Clarification of Cheese Manufacture By-Products. Food Hydrocolloids. 2004, 18, 601–610. DOI: 10.1016/j.foodhyd.2003.10.003.
- Moros, J. E.; Franco, J. M.; Gallegos, C. Rheology of Spray-Dried Egg Yolk-Stabilized Emulsions. International Journal of Food Science & Technology. 2002, 37, 297–307. DOI: 10.1046/j.1365-2621.2002.00570.x.
- Zayas, J. F. Functionality of Proteins in Food; Springer-Verlag: Berlin, Germany, 1997; 373 pp.
- Yamauchi, K.; Shimizu, M.; Kamiya, T. Emulsifying Properties of Whey Proteins. Journal of Food Science. 1980, 45, 1237–1242. DOI: 10.1111/jfds.1980.45.issue-5.
- Foley, J.; O´Connell, C. Comparative Emulsifying Properties of Sodium Caseinate and Whey Protein Isolate in 18% Oil in Aqueous Systems. Journal of Dairy Research. 1990, 53, 377–391. DOI: 10.1017/S0022029900027035.
- Patel, M. T.; Kilara, A. Studies on Whey Protein Concentrates. 2. Foaming and Emulsifying Properties and Their Relationships with Physicochemical Properties. Journal of Dairy Science. 1990, 73, 2731–2740. DOI: 10.3168/jds.S0022-0302(90)78958-8.
- Ju, Z. Y.; Kilara, A. Textural Properties of Cold-Set Gels Induced from Heat-Denatured Whey Protein Isolates. Journal of Food Science. 1998, 63, 288–292. DOI: 10.1111/j.1365-2621.1998.tb15728.x.
- Tippetts, M.; Shen, F. K.; Martini, S. Oil Globule Microstructure of Protein/Polysaccharide or Protein/Protein Bilayer Emulsions at Various pH. Food Hydrocolloids. 2013, 30, 559–566. DOI: 10.1016/j.foodhyd.2012.07.012.
- Kiokas, S.; Reiffers-Magnani, C. K.; Bot, A. Stability of Whey-Protein-Stabilized Oil-In-Water Emulsions during Chilled Storage and Temperature Cycling. Journal of Agricultural and Food Chemistry. 2004, 52, 3823–3830. DOI: 10.1021/jf035441r.