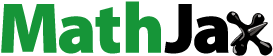
ABSTRACT
In this study, the relationship between protein hydrolysis and oil release was studied by fluorescence microscopy and Fourier Transform Infrared (FTIR) spectroscopy, respectively. Five commercial proteases (Flavourzyme 1000L, Neutrase 1.5MG, Protamex, Alcalase 2.4L, and Thermolysin) were evaluated for oil extraction from rapeseed, and Alcalase 2.4L was found to be the best enzyme for highest free oil yield (81.81%). When use this enzyme, the results showed that parts of proteins restricted the release of oil, and the oil released almost completely was achieved after hydrolysis for 3.0 h. To further reveal the relationship between oil release and protein hydrolysis, the effects of hydrolysis time on the degree of hydrolysis of protein, and free oil yield were studied. The results showed that the release of oil was only related to the hydrolysis of parts of proteins, and when the degree of hydrolysis of protein was more than 17.05%, the effect of protein hydrolysis on oil release was greatly reduced. The intrinsic relationship between the structural changes of proteins and the release of oil in the hydrolysis process was analyzed by comparing protein secondary structure of different hydrolysis time, and the results showed that the transition of protein structure from order to disorder was an important factor for oil release.
Introduction
Currently, solvent extraction is the most economical, efficient, and widely used in rapeseed oil-processing industry due to the high oil recovery. Although the solvent extraction has highly increased the oil-extraction rate of rapeseed compared to the conventional mechanical pressing process[Citation1]; however, it still cannot overcome the problems of the poor quality of oil,[Citation2,Citation3] and serious emission of organic compounds. With the increasing emphasis on oil nutrition and environmental pollution, the demand for rapeseed oil green extraction processing is becoming increasingly urgent.
Aqueous enzymatic extraction process, being applied to the oil extraction in the 1980s,[Citation4] is considered to be the most appreciate process for replacing solvent extraction, which is safety, regulatory, environment friendly,[Citation5–Citation8] and provides oils with favorable characteristics. Despite the advantages, the development of such process was once limited by the unavailability of enzymes. With the emergence of new types of enzyme, and enzyme prices going down, the interest in this technology has increased in recent years.
For rapeseed, the important issue facing aqueous enzymatic extraction process is the low oil extraction yield, that is, incompletely release of oil.[Citation9–Citation12] In this regard, many scholars have also carried out related researches. Guillaume et al.[Citation13] studied the relationship between cell wall digestion and oil release, and pointed that due to the presence of rapeseed oil emulsion, even if the cell wall was highly digested, the oil still could not fully released. For the effective release of the oil, Shao et al.[Citation14] effectively disrupted the rapeseed cell wall by combining with mechanical grinding and compound polysaccharide enzyme, which led to the full release of oil. Fullbrook[Citation15] used the crude enzyme preparations from Aspergillus sp, and achieved the highest oil recovery was 72%. The above studies have made the useful contributions to the exploration of the release of oil in the aqueous enzymatic oil extraction of rapeseed; however, little studies reported the relationship between protein hydrolysis and oil release.
To perfect the mechanism of aqueous enzymatic extraction of rapeseed oil, the present study observed the changes of protein and oil states during hydrolysis, and analyzed the relationship between protein hydrolysis and oil release through inspection the degree of hydrolysis and secondary structure of protein.
Materials and methods
Materials
Dehulled rapeseeds of Brassica napus (46.67% oil, and 23.71% protein, respectively) were obtained from the Institute of Oil Crops Research of the Chinese Academy of Agriculture Sciences (Wuhan, China). Flavourzyme 1000L (Aspergillus oryzae, 1000L APU/g), Neutrase (EC 3.2.1.8, Bacillus subtilis, 1.5 AU-N/g), Protamex (EC 3.4.21.62, and 3.4.24.28, a Bacillus protease complex, 1.5 AU-N/g), Alcalase 2.4L (EC 3.4.21.62, Bacillus licheniformis, 2.4 AU-A/g), and Thermolysin (EC thermoproteolyticus rokko, 80 U/mg) were provided by Novozymes (China) Biotechnology Co. Ltd. Nile Red and Fluorescein isothiocyanate (FITC) were purchased from Sigma-Aldrich China (Shanghai, China).
Pretreatment and aqueous enzymatic extraction
The flow chart for aqueous enzymatic extraction of rapeseed oil is shown in . As such, the dehulled rapeseed were first treated by an electric near-infrared oven at 120°C for 30 min to inactive the native myrosinase and enhance rapeseed oil flavor, followed by dry grinding for 5 min in a DFY-400 grinder to ruptured the cell wall. Then, the seeds-to-water ratio was adjusted to 1:3 to obtain a uniform slurry, and boiled the slurry for 15 min to denature the protein related to emulsification. A fixed amount of slurry (containing 50 g original dry rapeseeds) was transferred to a 500-mL jacketed glass reactor equipped with a thermostatically controlled water heater. The seeds to water ratio was fixed at 1:5 (w/v), and the slurry pH was adjusted to 9.0 to embark on the alkaline extraction at 60°C for 1 h to promote the action of enzyme and the accumulation of oil.
At the end of alkaline extraction condition, the pH was readjusted to desired value to suite different enzyme. After that, Flavourzyme 1000L, Neutrase 1.5MG, Protamex, Alcalase 2.4L, and Thermolysin were then added to the slurry at 1.5% (v/w) of the rapeseed protein to commence the hydrolysis at the desired temperature for 3.5 h with an agitation rate at 200 rpm, respectively. The suspension was heated at 90°C for 10 min to inactive the enzyme activity, followed by cooling to room temperature. Following the incubation, the suspension was centrifuged at 4500 g for 15 min. The layers of free oil and emulsion phase were collected separately, while the precipitate was freeze dried. And this freeze dried precipitate was pulverized and analyzed for residual oil. A suitable enzyme was selected based on high free oil recovery.
Extraction yield
The yields of total oil extraction, free oil, and emulsified oil were expressed using Eqs (1)–(3), respectively.
Fluorescence microscopy
To better reveal the relationship between protein hydrolysis and oil release, the free oil released during the process of smashing removed by centrifuging at 4500 r/min for 10 min. Then the precipitate was collected for the next hydrolysis operation. Samples obtained from different phases were fixed with 2% glutaraldehyde in 0.1M phosphate buffer, pH 7.0 for 24 h before dehydration with an ethanol series (50–100%), infiltrated and embedded in glycol methacrylate resin (Historesin, Leica).[Citation16] The sectioned samples were analyzed by fluorescence microscopy with fluorochromes for each specific component: FITC for protein at an excitation wavelength (λ) of 488 nm,[Citation17] and Nile red for lipids at (λ) 514 nm.[Citation18]
Protein degree of hydrolysis
The degree of protein hydrolysis (DH), defined as the percentage of peptide bonds cleaved, was determined by using the pH-Stat method. Hydrolysis experiments were carried out in a 500-mL jacketed glass reactor at the same time with aqueous enzymatic extraction of rapeseed oil. 2N NaOH was added by the titration to maintain constant pH at the desired value (pH 8.5). Under alkaline conditions, the DH is proportional to the base consumption of NaOH[Citation19] required to maintain constant pH, and was calculated according to Eq. (4) described by Adler-Nissen.[Citation20]
where h is the hydrolysis equivalent or the amount of peptide cleaved during the hydrolysis (in meqv peptide bonds per gram protein), htot is the total amount of peptide bonds determined from an amino acid assay, and assumed to be 8.35 meqv/g for rapeseed protein, V is the volume of NaOH consumed during hydrolysis (mL), Nb is the normality of the base, α is the average degree of dissociation of the terminal amino peptides (at pH 8.5 and 60°C, the calibration factor 1/α can be estimated to be 1.03),[Citation20] and Mp is the mass (g) of protein in the dehulled rapeseed sample.
FTIR spectroscopy spectra
The secondary structure conformation of the freeze-dried proteins from different phases was investigated by using a Thermo Nicolet Nexus 470 FTIR spectrometer (Thermo Nicolet Analytical Instruments, MA, USA) in a range of 4000–400 cm−1.[Citation21] The freeze-dried proteins were prepared with potassium bromide (KBr) pellet method. Each interferogram was an average of 64 scans at 4 cm−1 resolution, and a 2 cm−1 interval.[Citation22] After deconvolution and secondary-derivation, the amide I band in the original spectra was analyzed by using Omnic (version V6.2, Thermo Nicolet, USA), and Peak Fit 4.12 software, respectively.
Statistical analysis
All tests were performed using triplicate samples. The values were reported as the mean and standard deviations for at least three trials. One-way SPSS program (SPSS Statistical Software, Inc., IL, USA) was used to process the data. The significant differences (p < 0.05) were evaluated with SPSS software (version 13.0, SPSS Inc., Chicago, IL, USA).
Results and discussion
Effect of protease type on oil release
Five commercial proteases (Flavourzyme 1000L, Neutrase 1.5MG, Protamex, Alcalase 2.4L, and Thermolysin) were selected, and their effects on free oil and total oil yield were compared, respectively. Different optimal enzymatic hydrolysis conditions and results are shown in and , respectively. On the basis of breaking rapeseeds cell completely, proteases had little effect on total oil yield, but had great effects on free oil yield. The control group without any enzyme had the lowest free oil yield at 70.02%. With the addition of different proteases, free oil yield increased in different degrees. Among these five enzymes, Alcalase 2.4L was found to be the most desirable enzyme, which offered the highest free oil yield (81.81%), while Flavourzyme 1000L offered the lowest free oil yield as 70.54%. The studied enzymes exhibited efficacy on oil release in the order: Alcalase 2.4L > Thermolysin > Protamex > Neutrase 1.5MG > Flavourzyme 1000L. As a result, Alcalase 2.4L was chosen as the optimum enzyme for enzymatic treatment, which was in agreement with the results of aqueous enzymatic sesame oil reported by Latif et al.[Citation23]
Table 1. Optimal enzymatic hydrolysis conditions of proteases.
Effect of proteolysis state on oil release
The technique of fluorescence microscopy was used to observe changes of oil and protein during hydrolysis process. As can be seen from , in the control group, the protein and oil combined tightly, and there were no free oils being released, which indicating proteins restricted the oil release. While at 1.0 h (), a small portion of proteins were hydrolyzed, some oil was released and gathered into small droplets as well. After hydrolysis for 2.0 h (), most proteins were hydrolyzed, and more oil was released, as the result, the size of oil droplets became larger than that of before. In the , there were a large amount of oils but little proteins, which indicating after hydrolysis for 2.5 h, proteins were hydrolyzed to soluble state as FITC had not been unable to dye the protein. Oils were released almost completely after hydrolyzed 3.0 h (), and extending the hydrolysis time, there were no noticeable changes on the amount and particle size of oil droplets (). In summary, it was the protein that restricted the release of oil, and after hydrolysis for 3.0 h, the oil was released completely.
Effect of degree of hydrolysis on oil release
To further reveal the relationship between oil release and protein hydrolysis, the effects of hydrolysis time on protein degree of hydrolysis and free oil yield were studied, respectively, and results were showed in . As the increasing of hydrolysis time, the protein degree of hydrolysis and free oil yield were increased gradually, and free oil yield was reached peak at the hydrolysis time of 3.0 h, which was consistent with the results of . The cause may be that protein peptide bonds were exposed gradually in the hydrolysis treatment, which was beneficial for protein hydrolysis. The release of oil increased with the increase of protein hydrolysis degree when the degree of protein hydrolysis was less than 17.05%. While when the degree of protein hydrolysis increased continually, oil release maintained at the same level. Thus, it could be concluded that the process of protein hydrolysis and oil release was inhomogeneous. The results showed that the release of oil was only related to the hydrolysis of parts of proteins, and when the protein degree of hydrolysis was more than 17.05%, the effect of protein hydrolysis on oil release was greatly reduced.
Effect of protein secondary structure on oil release
FTIR spectroscopy was used to detect the changes of protein secondary structure during the hydrolysis process. The intrinsic relationship between the structural changes of proteins and the release of oil in the hydrolysis process was analyzed by comparing the changes of protein secondary structure with the hydrolysis time, and the results were showed in and , respectively. showed that protein secondary structure was changed in the hydrolysis process. Compared with the control group, the content of α-helix of protein at hydrolysis time 4.0 h reduced 12.1%, while the content of β-sheet, β-turn, and random coil increased 6.0%, 4.6%, and 1.5%, respectively. Thus, it can be concluded that the transition of protein structure from order to disorder was an important factor for the oil release. That is to say, the disorder of protein structure was favorable for the protein enzymatic hydrolysis and oil release.
Table 2. The secondary structure content (%) of proteins in different hydrolysis time.
Conclusions
The effects of hydrolysis state and secondary structure of protein on oil release in aqueous enzymatic extraction of rapeseed oil was studied. The results showed that Alcalase 2.4L was the best enzyme for highest free oil yield (81.81%) in enzymatic treatment. And the observation of the changes of protein and oil states during hydrolysis showed that parts of protein restricted the release of oil, and after hydrolysis for 3.0 h, the oil was released almost completely. The relationship between secondary structure of the protein and oil release was revealed by FTIR, and the results showed that oil release was affected by the secondary structure of the protein, and in the aqueous enzymatic extraction process, the structure of protein changed from order to disorder, which was beneficial to oil release. Thus, it could be concluded that the transition of protein structure from order to disorder was an important factor for oil release.
Additional information
Funding
References
- Hoed, V. V.; Ben Ali, C.; Slah, M.; Verhé:, R. Quality Differences between Pre-Pressed and Solvent Extracted Rapeseed Oil. European Journal of Lipid Science & Technology 2010, 112, 1241–1247. DOI: 10.1002/ejlt.v112.11.
- Rombaut, N.; Savoire, R.; Thomasset, B.; Castello, J.; Hecke, E. V.; Lanoisellé:, J. L. Optimization of Oil Yield and Oil Total Phenolic Content during Grape Seed Cold Screw Pressing. Industrial Crops & Products. 2015, 63, 26–33. DOI: 10.1016/j.indcrop.2014.10.001.
- Ahlin, K. A.; Emanuelson, M.; Wiktorsson, H. Rapeseed Products from Double-Low Cultivars as Feed for Dairy Cows: Effects of Long-Term Feeding on Thyroid Function, Fertility and Animal Health. Acta Vet. Scand. 1994, 35, 37–53.
- Olsen:, H. S.;. [Aqueous Enzymatic Extraction of Rape Oil]; [Danish]. Bagsvaerd, Denmark, Novo Industri, 1988.
- Moreau, R. A.; Dickey, L. C.; Johnston, D. B.; Hicks:, K. B. A Process for the Aqueous Enzymatic Extraction of Corn Oil from Dry Milled Corn Germ and Enzymatic Wet Milled Corn Germ (E-Germ). JAOCS, Journal of the American Oil Chemists' Society 2009, 86, 469–474. DOI: 10.1007/s11746-009-1363-x.
- Li, Y.; Zhang, Y.; Wang, M.; Jiang, L.; Sui:, X. Simplex-Centroid Mixture Design Applied to the Aqueous Enzymatic Extraction of Fatty Acid-Balanced Oil from Mixed Seeds. Journal of the American Oil Chemists' Society 2013, 90, 349–357. DOI: 10.1007/s11746-012-2180-1.
- Hou, L. X.; Shang, X. L.; Wang, X.; Liu:, J. Application of Enzyme in Aqueous Extraction of Sesame Oil. European Food Research and Technology 2013, 236, 1027–1030. DOI: 10.1007/s00217-013-1955-4.
- Ramadan, M. F.; Sitohy, M. Z.; Moersel:, J.-T. Solvent and Enzyme-Aided Aqueous Extraction of Goldenberry (Physalis Peruviana L.) Pomace Oil: Impact of Processing on Composition and Quality of Oil and Meal. European Food Research and Technology. 2008, 226, 1445–1458. DOI: 10.1007/s00217-007-0676-y.
- Ohlson:, R.;. Modern Processing of Rapeseed. Journal of the American Oil Chemists' Society. 1992, 69, 195–198. DOI: 10.1007/BF02635885.
- Bagger, C. L.; Olsen, H. S.; Steiger, W.; Persson, L. I.; Ingvardsen, L.; Soerensen:, H. The Whole Crop Biorefinery Project: Task 5: Final Report: Aqueous Enzymatic Extraction of Rapeseed Oil, Protein, Starch and Syrup. Denmark, Bioraf Denmark Foundation, 1995.
- Yang, B.; Wang, Y. H.; Yang:, J. G. Optimization of Enzymatic Degumming Process for Rapeseed Oil. Journal of the American Oil Chemists' Society. 2006, 83, 653–658. DOI: 10.1007/s11746-006-1253-4.
- Zhang, S. B.; Zhang, W.; Xu:, S. Y. Downstream Processes for Aqueous Enzymatic Extraction of Rapeseed Oil and Protein Hydrolysates. Journal of the American Oil Chemists' Society. 2007, 84, 693–700. DOI: 10.1007/s11746-007-1080-2.
- Ricochon, G.; Elfassy, A.; Pages, X.; Piffaut, B.; Girardin, M.; Muniglia:, L. Correlation between the Release of Sugars and Uronic Acid and Free Oil Recovery following Enzymatic Digestion of Oil Seed Cell Walls. Bioresource Technology 2011, 102, 9599–9604. DOI: 10.1016/j.biortech.2011.06.097.
- Shao, B. Z.; Zhang, W.; Shi:, Y. X. Optimization of the Aqueous Enzymatic Extraction of Rapeseed Oil and Protein Hydrolysates. Journal of the American Oil Chemists' Society. 2007, 84, 97–105. DOI: 10.1007/s11746-006-1004-6.
- Fullbrook:, P. D.;. The Use of Enzymes in the Processing of Oilseeds. Journal of the American Oil Chemists' Society. 1983, 60, 476–478. DOI: 10.1007/BF02543552.
- Mat Yusoff, M.; Gordon, M. H.; Niranjan:, K. Aqueous Enzyme Assisted Oil Extraction from Oilseeds Andemulsion De-Emulsifying Methods: A Review. Trends in Food Science and Technology 2015, 41, 60–82. DOI: 10.1016/j.tifs.2014.09.003.
- Muramoto, K.; Meguro, H.; Tuzimura:, K. Application of Fluoresceinisothiocyanate Labeling of Protein to SDS-Electrophoresis and Subsequent Sequence Analysis. Agricultural & Biological Chemistry. 2014, 41, 2059–2062.
- Aguedo, M.; Waché, †, Y.; Virginie Mazoyer, S. L. G.; Belin†, ‡., . J. M. Increased Electron Donor and Electron Acceptor Characters Enhance the Adhesion between Oil Droplets and Cells of Yarrowia Lipolytica as Evaluated by a New Cytometric Assay. Journal of Agricultural & Food Chemistry 2003, 51, 3007–3011.
- Assanvo, E. F.; Kalita, M.; Saikia, M.; Baruah:, S. D. Aqueous Extraction of Ricinodendron Heudelotii Oil and Direct Miniemulsion Polymerization of the Oil-In-Water Fraction. Industrial Crops & Products 2016, 81, 30–37. DOI: 10.1016/j.indcrop.2015.11.048.
- Adlernissen, J.; Enzymic Hydrolysis Of Food Proteins. Canadian Medical Association Journal 1986, 172, 1783–1785.
- Peiqiang, Y. †.; C. R. C., M. K. †.; Christensen†, §., . D. A. Using Synchrotron-Based FTIR Microspectroscopy to Reveal Chemical Features of Feather Protein Secondary Structure: Comparison with Other Feed Protein Sources. Journal of Agricultural & Food Chemistry. 2004, 52, 7353–7361. DOI: 10.1021/jf0490955.
- Wang, C.; Jiang, L.; Wei, D.; Li, Y.; Sui, X.; Wang, Z.; Li:, D. Effect of Secondary Structure Determined by FTIR Spectra on Surface Hydrophobicity of Soybean Protein Isolate. Procedia Engineering 2011, 15, 4819–4827. DOI: 10.1016/j.proeng.2011.08.900.
- Latif, S.; Anwar:, F. Aqueous Enzymatic Sesame Oil and Protein Extraction. Food Chemistry 2011, 125, 679–684. DOI: 10.1016/j.foodchem.2010.09.064.