ABSTRACT
Three palm mid-fraction (PMF) groups produced from different fractionation paths were analyzed in terms of the fat, triacylglycerol and sn-2 fatty acid compositions, thermal properties (melting and crystallization behaviors, and solid fat contents (SFCs)), micronutrient levels and oxidative stability indexes (OSIs) to achieve their sufficient utilization. PMF-A (iodine value (IV), 48.4 g/100 g) fractionated from palm olein contained 43.8% 1,3-dipalmitoyl-2-oleoyl-glycerol (POP) and 16.7% 1-palmitoyl-2,3-dioleoyl-glycerol, showing soften properties (slip melting point (SMP), 27.6°C; SFC at 30°C, 2.2%) at hot weather. PMF-B (IV, 42.3 g/100g) obtained from palm stearin showed similar fat and triacylglycerol compositions as PMF-A, but the high 1,2,3-tripalmitoyl-glycerol level (6.2%) improves its thermal behaviors (SMP, 34.9°C; SFC at 30°C, 13.8%). The SFC profiles of PMF-A and PMF-B were comparable to those found in frozen and puff margarine shortenings. Furthermore, both of the PMF groups exhibited excellent OSIs (13.2 and 11.2 h, respectively) because of their high micronutrient levels (especially γ-tocopherol and campesterol). In general, γ-tocopherol and campesterol contribute to preventing lipids from oxidation under frying conditions. Therefore, PMF-A and PMF-B are recommended for manufacturing margarine shortenings and frying fasts. PMF-C (IV, 33.0 g/100g) produced from the additional fractionation of PMF-A or PMF-B contained the highest POP percentage (67.1%) and showed heat-resistant property (SMP, 31.8°C; SFC at 30°C, 22.4%). Its steep SFC profile was superior to that of cocoa butter, suggesting the fat is preferred in producing hard chocolate fats.
Introduction
Palm mid-fractions (PMFs) are produced by multi-stage fractionation from palm oil and has been widely popular in the formulation of various food ingredients (e.g., chocolate/confectionery fats, icings, shortenings, margarines and frying fats) due to their high yields, unique physicochemical properties and nutritional values.[Citation1] The annual output of PMFs amounts to 14–17 million tons calculated based on the production quantity of palm oil and related fractionation yields[Citation2], which meets the demands for processed fat-containing food. For instance, PMFs are strongly suggested to be used as chocolate fats, and the potential demand of cocoa butter equivalents (CBEs, one of the high-quality chocolate fats) is estimated to be only 0.025–0.050 million tons annually according to the European Directive 2000/36/EC.[Citation1,Citation3] In contrast, most of other chocolate fat resources, i.e., illipe butter, sal fat, and shea butter, are usually difficult to obtain because of their intermittent availabilities and low yields.[Citation4] In this regard, PMFs are the most ideal ingredients in the confectionery manufacture.
In general, industrial PMFs are divided into three groups as shown in : PMF-A, also known as soft PMF, is generally obtained from dry fractionation of the palm olein or PMF-B as the following process: fast cooling to 16°C and keeping at this temperature for 1 h, follow by fast or slow cooling to 12–13°C and holding for 5–7 h[Citation1,Citation5,Citation6]; PMF-B, also named soft stearin or low melting stearin, is generally fractionated from the palm stearin or PMF-A using acetone in a ratio of 1:4–8 (w:v) at 17–25°C for 8–48 h[Citation5,Citation7]; and PMF-C, also called hard PMF or 1,3-dipalmitoyl-2-oleoyl-glycerol (POP)-rich fat, is produced from the additional fractionation of PMF-A or PMF-B using acetone in a ratio of 1:10 (w:v) at 4°C for 24 h.[Citation5,Citation8]
PMFs obtained from different fractionation routes have different chemical compositions (especially the triacylglycerol compositions and diacylglycerol levels) and thermal properties, which might greatly affect the physical quality (especially the thermal behaviors and sensory characteristics) of final products. As our previous study, PMF-C with 65.0% of POP and 1.3% of diacylglycerols was demonstrated to be the best compared to the PMF-A (POP, 40.4%; diacylglycerols, 4.3%) and PMF-B (POP, 44.9%; diacylglycerols, 4.0%) in preparing heat-resistant chocolate fats by blending mango kernel fat.[Citation9] For other specialty usages, PMF-A (slip melting point (SMP), 25.8°C) and PMF-B (32.3–34.9°C) were recommended as the shortening ingredients by mixing with lard in the ratios of 80%:20% (PMF:lard, %wt), while the ratio was changed to be 10%:90% (PMF:lard, %wt) when PMF-C (30.9°C) was involved.[Citation10] In addition, most of the current reports does not point out the PMF types when the fats were involved in preparing the products, which may cause problems to researchers who want to repeat the studies or to continue to further experiments. Therefore, both of PMF types and mixing ratios should be paid attention to the manufacture of different fat-containing foods. It would help to better understand how the different PMFs interact with other fats or oils in the blends in terms of their physical properties. As Bootello et al.[Citation11] mentioned that it is interesting to investigate the maximum amount of PMFs in the CBE formulas. However, there is little information about a thorough interpretation for the three group PMFs, which further limits their application.
In addition, PMFs are also a source of valuable micronutrients, such as sterol, tocopherol and carotene.[Citation7,Citation12] Although micronutrients might not significantly change the physical characteristics of PMF-containing food, they would be the key factors that take influences on the oxidative stabilities or shelf life of the products.[Citation13,Citation14] This also improves the importance of systemically analyzing the PMFs.
Therefore, the present study focuses on the three PMF groups (i.e., PMF-A, PMF-B and PMF-C) obtained from multi-step fractionation by analyzing their fat and triacylglycerol compositions, micronutrient levels, thermal properties, and oxidative stabilities, and to further extend their usages in fat based food products.
Materials and methods
Materials
Twenty-seven different batches of PMFs, including twelve batches of PMF-A, six batches of PMF-B, and nine batches of PMF-C, were collected from industrial fractionation plants in China (Wilmar Group, China Oil & Foodstuffs Corporation, Julong Group and Cargill China) and Malaysia (IOI Group) over a period of last 2 years. Commercial CBE, cocoa butter (CB) were donated by Wilmar Group and Jinsihou Group respectively. Frozen food shortening was prepared by mixing PMFs and lard.[Citation10] Each sample was collected in duplicate and kept frozen at −20°C in glass containers until it was ready to be analyzed.
Standards of oleic acid, 2-olein, 1,3-diolein, 1,2-diolein, and 37 fatty acid methyl esters were provided by Sigma-Aldrich Chemical Co. Ltd. (Shanghai, China); and triacylglycerols, including POP, 1-palmitoyl-2,3-dioleoyl-glycerol (POO), and 1-palmitoyl-2-oleoyl-3-stearoyl-glycerol (POS), were purchased from Larodan Fine Chemicals AB (Malmö, Sweden). Pancreatic lipase was bought from Sigma-Aldrich Chemical Co. Ltd. (Shanghai, China). Other chemical reagents were obtained from Sinopharm Chemical Regent (Shanghai, China).
Fat composition determination
Fat composition, including triacylglycerol, diacylglycerol and monoacylglycerol, and free fatty acid, were detected by a high-performance liquid chromatographic system (LC-20AT, Shimadzu, Japan) consisted of a refractive index detector (RID-10A, Shimadzu, Japan) and a silica column (5 µm, 4.6 × 250 mm, Sepax, USA).[Citation15] Conditions of analysis: mobile phase, n-hexane, isopropanol, and methanolic acid (15:1:0.003, v:v:v); flow rate, 1.0 mL min−1; sample concentration, 20 mg mL−1; injection volume, 10 µL. Standards of oleic acid, 2-olein, 1,3-diolein, 1,2-diolein, and triacylglycerol, were used to identify the relative peaks, and the percentages were reported in terms of relevant proportions.
Triacylglycerol composition determination
Triacylglycerol profile was detected by a high-performance liquid chromatograph (Agilent 1200) consisted of a RID (RID-10A, Shimadzu, Japan) and two LiChroCART 18e columns (5 µm, 4.6 × 250 mm each; Merck, Germany) according to the AOCS Official Method Ce 5c-93.[Citation16] Conditions of analysis: mobile phase, acetone and acetonitrile (75:25, v:v); flow rate, 1.0 mL min−1; sample concentration, 30 mg mL−1; injection volume, 20 µL. Triacylglycerol species were identified by comparing the retention time of standards and by correlating the equivalent carbon number, and their percentages were reported in terms of the relative proportions.
Sn-2 fatty acid composition determination
Triacylglycerols were hydrolyzed by pancreatic lipase to prepare 2-monoacylglycerols based on the method described by Luddy et al.[Citation17] The monoacylglycerols were then mixed with 1.5 mL n-hexane and methyl esterified using 2 mol L−1 KOH-CH3OH to prepare fatty acid methyl esters. The analysis was performed on a gas chromatograph system (7820A, Agilent, USA) equipped with a flame ionization detector (FID) and a Trace TR-FAME capillary column (0.25 µm, 60 m × 0.25 mm, Thermo Fisher, USA). Conditions of analysis: FID and injector temperature, 250°C; oven temperature = 80 (3min) - 215°C (15°C min−1) - 215°C (20 min); injection volume, 1.0 µL; nitrogen (carrier gas), 1 mL min−1; split ratio, 1:20. Fatty acid species were identified by comparing the retention time of standards and their percentages were reported in terms of the relative proportions.
Slip melting point and iodine value determination
SMP and iodine value (IV) were quantified using the AOCS Official Method Cc 1–25 and Cd 1–25, respectively.[Citation16]
Micronutrient determination
Tocopherols were detected by a high-performance liquid chromatographic system (LC-20AT, Shimadzu, Japan) consisted of an ultraviolet detector (SPD-20A, Shimadzu, Japan) and a silica column (5 µm, 4.6 × 250 mm, Hanbon, China) based on our pervious method.[Citation18] Conditions of analysis: mobile phase, hexane and isopropanol (98.5:1.5, v:v); flow rate, 1.0 mL min−1; column temperature, 30°C; detection wavelength, 295 nm; sample concentration, 100 mg mL−1; injection volume, 20 µL. Tocopherol isomers, including α-, β-, γ-, and δ-tocopherols, were identified and quantified by comparing the standards, and their levels were reported in mg kg−1.
Sterols were analyzed using a gas chromatograph-mass spectrum system (Thermo Fisher, USA) consisted of a FID and a DB-5 capillary column (0.25 µm, 30 m × 0.25 mm, Agilent, USA) as described by Li et al.[Citation19] Two hundred milligram of the fat and 0.2 mL of 0.825 mg mL−1 5α-cholestane (the internal standard) were saponified by 2 mL of 2 mol L−1 KOH-CH3CH2OH at 85°C for 1 h, and then 5 mL of hexane and 2 mL of distilled water were added to extract the unsaponifiables three times. The extractive was then dried by nitrogen and silylated with 400 µL BSTFA + TMCS at 70°C for 30 min, and finally was dissolved using 1 mL hexane. Conditions of gas chromatograph analysis: FID and injector temperature, 290°C; oven temperature = 200 (1 min) - 300°C (1°C min−1, 18 min); helium (carrier gas), 1 mL min−1; injection volume, 1.0 µL; split ratio, 1:100. Conditions of mass spectrum analysis: ionization mode, EI; mass range (m/z), 50–500; transmission line temperature, 250°C; source temperature, 280°C. 5α-cholestane was used to quantify the contents of sterols, and the levels were reported in mg/kg
Melting and crystallization analyses
Melting and crystallization behavior analyses were conducted on a DSC instrument (DSC Q200, TA, USA) based on the AOCS Official Method Cj 1–94.[Citation16] The melted sample without thermal memory (8.0 ± 0.5 mg) was cooled from 80 to −40°C at 10°C min−1 and maintained 5 min to obtain the crystallization curve, and was then heated to 80°C at 5°C min−1 to obtain the melting curve. The enthalpy, and onset, maximum and offset temperatures of each peak were calculated from the curves using TA Universal Analysis 2000.
Solid fat content determination
Solid fat content (SFC) of each fat was measured according to the AOCS Official Method Cd 16b-93[Citation16] by a pulse nuclear magnetic resonance (AM4000, Oxford, UK). The melted sample (3.0–3.2 g) was poured into a NMR tube and melted at 80°C for 60 min followed by tempering at 0°C for 60 min. The value was detected from 0 to 40°C (with 5°C intervals) and equilibrated at each setting temperature for 30 min before determination.
Oxidative stability index determination
Oxidative stability index (OSI) of each fat was detected according to the AOCS Cd 12b-92 method[Citation16] using a Metrohm Rancimat model 743 (Herisau, Switzerland). Four grams of the fat was heated at 120°C with 20 L h−1 of the cleaned and dried air, and then the effluent air containing volatile organic acids from the fat was collected in a measuring vessel with 60 mL of distilled water. The conductivity of the water was determinated automatically as oxidation proceeded, and the result was recorded as an hour (h).
Statistical analysis
All the determinations mentioned above were carried out in triplicates and were reported as mean ± standard deviation. Analysis of variance (ANOVA) and a LSD test were used to test the differences using SPSS program version 19.0, a p < 0.05 was considered to be significant difference. The correlations between OSI and tocopherols/sterols were calculated by multiple linear regression analysis using a stepwise method. The use probability of F values p-to-enter and p-to-remove new variables into statistical model were p < 0.05 and p > 0.10, respectively.
Results and discussion
Chemical compositions
IV reflects the unsaturation of fats (the chemical compositions) and is the important analytical parameter, which is related strongly to the SFC (the thermal properties).[Citation20] The values are presented in and were reported with the values of 48.4, 42.3, and 33.0 g/100g for PMF-A, PMF-B and PMF-C, respectively. They fell within the ranges of 42.0–48.0 g 100g−1 for soft PMF, 40.0–42.0 g 100 g−1 for soft stearin and 32.0–36.0 g 100g−1 for hard PMF respectively[Citation5], indicating the studied PMF samples are representative. However, few information about their chemical compositions and thermal properties are systematically reported, which are the impetus for the present study.
Table 1. Iodine values, and fat, triacylglycerol and sn-2 fatty acid compositions of PMFs.a
The chemical characteristics of three PMF groups, mainly including fat, triacylglycerol and sn-2 fatty acid compositions are given in . Significant differences (p < 0.05) of triacylglycerol and diacylglycerol percentages were found between PMF-C and PMF-A/PMF-B, while the monoacylglycerol and free fatty acid showed similar levels among the three group samples. PMF-A and PMF-B used here contained higher diacylglycerol percentages (4.2% and 4.7%, respectively) compared to PMF-C (0.3%). Accordingly, their triacylglycerol levels (93.8% and 93.1%, respectively) were significantly lower than that of PMF-C (99.1%). Fractionation is the preferred technique for obtaining triacylglycerols as diacylglycerols are removed as filtrates[Citation8,Citation21], which could explain the low diacylglycerol levels in PMF-C. High amounts of diacylglycerols would greatly delay the transition of symmetrical monounsaturated triacylglycerols into more stable polymorph and further give the chocolate products undesirable physical properties.[Citation22–Citation24] Rather, as Ray et al.[Citation22] reported, low diacylglycerol levels (0.7%) had less influence on the transformation from γ into β form. In this regard, PMF-C is more suitable for producing chocolate fats compared to PMF-A and PMF-B.
further shows the triacylglycerol and sn-2 fatty acid compositions of three PMF groups. sn-2 Fatty acid composition analyses revealed that oleic acid represented the greatest percentages among the studied samples ranging from 61.8% to 76.8%, which demonstrated that symmetrical monounsaturated triacylglycerols (e.g., POP, 43.8–67.1%; and POS, 8.1–13.1%) were the predominant triacylglycerols in all the studied samples. POP and POS, the high-melting triacylglycerols, are the main triacylglycerols in CB, suggesting that some PMFs are the preferred POP source to mix with 1,3-distearoyl-2-oleoyl-glycerol (SOS)-rich fats (e.g., mango kernel fat) to produce heat-resistant CBEs.[Citation9,Citation25–Citation27] In general, PMF-C is recommended to be used in the chocolate fat formulations because of its significantly higher POP content (>60.0%) and low diacylglycerol level (<1.5%).[Citation8,Citation28] For PMF-A and PMF-B, significant higher POO percentages were observed reaching 16.7% and 14.1%, respectively. POO could provide a fat system with fluidity that would be discussed in the next section, and such PMFs (POP, 29.6–45.2%; POO, 14.1–20.4%) are suitable in the shortening preparation.[Citation10] In addition, PMF-B obtained from the palm stearin generally contained high amounts of 1,2,3-tripalmitoyl-glycerol (PPP, 6.2%), which contributes to form hard texture in blending formula and is widely used in preparing frying fats.[Citation29]
Thermal properties
The melting and crystallization behaviors of PMFs are in relation to industrial applications. Their curves are shown in , and the parameters together with SMPs are presented in . The melting profile generally gives the information about the amounts of high-melting triacylglycerols and the occurrence of polymorphic transformation.[Citation29] A distinct melting peak was started at 12.0°C and ended at 37.0°C in PMF-C ()), whereas subtle peaks were observed in PMF-A (10.4–32.1°C) and PMF-B (4.6–37.7°C). The melting profile of PMF-C was similar to that of CB and commercial CBE, which also indicated that the fat contained more unique fat and triacylglycerol compositions.[Citation9] The significant lower onset temperatures in PMF-A and PMF-B suggested that they had higher levels of di- or tri-unsaturated triacylglycerols (mainly including POO, OOO and PLO, ) compared to PMF-C, but the higher PPP level in PMF-B still made the fat exhibited high SMP as PMF-C. In this regard, PMF-B and PMF-C are more popular in summertime and in most tropical countries.
Table 2. Slip melting points, melting, and crystallization characteristics of PMFs.a
Figure 2. Melting (a) and crystallization (b) profiles of palm mid-fractions, cocoa butter and commercial cocoa butter equivalent.
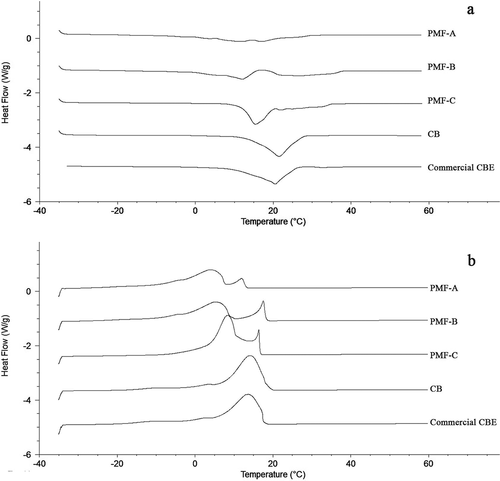
Similar heat-resistant properties of PMF-B and PMF-C were found in the crystallization properties as shown in . However, crystallization curves showed that all the three PMF groups had two exothermic peaks ()), which were quite different from those of CB and commercial CBE.[Citation9] High-melting PPP was considered to be responsible for the small shoulder between 15.0 and 20.0°C. The findings were in accordance with the previous study described by Sonwai et al.[Citation26] Therefore, too high percentages of PMFs in the chocolate fat mixtures might lead the blends undesirable in terms of compatibilities and thermal properties. As our previous research, 10% PMF-C was the ideal addition amount in preparing CBEs combined with CB and SOS-rich fat (data were not published). Smith et al. and Beckett[Citation4,Citation30] also concluded that the addition of suitable amounts of POP-rich fats could improve the compatibilities for hard chocolate fat formulas.
SFC is connected with the textural (softness, hardness, and melting behavior) and sensorial properties of fat-containing food.[Citation31,Citation32] The values of three PMF groups, CB and typical pastry shortening are shown in . In general, PMF-C had the highest solid contents at all the studied temperatures, followed by PMF-B and PMF-A. PMF-A (68.9%→2.2%) and PMF-B (79.9%→13.4%) showed steep profiles from 10 to 30°C. The gradual decrement trends were comparable to those of reference shortenings, i.e., frozen food shortening and typical puff pastry margarine.[Citation10,Citation33] However, the reference shortenings cannot melt completely at 40% (SFCs = 10.0–13.0%), which will induce a waxy mouth feel. By contrast, the studied PMF-A and PMF-B had short plastic ranges with high SFCs at low temperature (<20°C) and approximately zero SFCs at mouth temperature, which were recommended as good-quality shortenings.[Citation34] In addition, the profile of PMF-C was similar to that of CB, but shifted to higher temperatures, showing that PMF-C is the fat that would remain solid at high temperatures and contribute to preparing heat-resistant chocolate fats. For instance, both of PMF-C and CB had high SFCs (92.9% and 85.9%, respectively) at 15°C, and PMF-C still showed high values of 84.7% and 70.4% at 20 and 25°C respectively, while dramatic decreases from 69.6% to 33.1% were observed in CB in the same temperature range.
Micronutrient levels
Micronutrients, especially tocopherols and sterols, are usually considered as nutritional supplements and antioxidants for lipids, and their levels in PMFs are presented in . Significant differences of tocopherol contents were found in the three PMF groups. PMF-A contained the highest value reaching 300 mg kg−1, followed by PMF-B (158 mg kg−1) and PMF-C (only 7 mg/kg−1). Micronutrients tend to be concentrated into the liquids during the fractionation, which could be responsible for the significant changes.[Citation1] Kumar et al.[Citation7] also concluded that only 38 mg kg−1 was found in the soft stearin (i.e., PMF-B in present study) after two-stage fractionation of crude red palm oil. In addition, the tocopherols in studied PMFs were mostly dominated by γ-tocopherol (53%-71%), which was in accordance with the results reported by Hamm et al.[Citation35] They concluded that γ-tocopherol accounted for about 46% of the total tocopherols in palm oil. In general, γ-tocopherol exhibits excellent antioxidant capacity at high temperature (> 50°C) compared to that of α-tocopherol and β-tocopherol, especially under the condition that the total tocopherol concentration is more than 100 mg/kg.[Citation36,Citation37] Therefore, it suggests that such PMFs (i.e., PMF-A and PMF-B) are suitable in using frying fats.
Table 3. Tocopherols, sterols, and oxidative stability indexes of PMFs.a
Sterols are the major unsaponifiables in almost all the vegetable fats and oils. As shown in , the highest sterol content was found in PMF-A (535 mg kg−1), followed by 370 mg kg−1 in PMF-B, but there were no sterols detected in PMF-C. The trends were similar to those found in tocopherols and reported by Kumar et al.[Citation7] Sterol levels in fractionated PMFs were comparable to that in reported palm oils (300–700 mg/kg), but were significantly lower than those in common oils, e.g., rape oil (4500–11300 mg kg−1), soybean oil (1800–4500 mg kg−1), and sunflower oil (2400–5000 mg kg−1).[Citation35] As previous reports, β-sitosterol is the most abundant specie among the sterols in palm oils, followed by campesterol and stigmasterol[Citation35], which was also found in present study.
Characteristics of oxidative stabilities
OSI is the most widely applied index to evaluate the shelf life of edible fats and oils. also shows the results for OSIs of the three PMF groups ranging from 8.8 to 13.2 h. All the values were significantly higher than those found in common edible oils (3.1–5.0 h), e.g., sunflower, canola, soybean, peanut, and corn oils[Citation38], indicating that PMFs could improve the oxidation stabilities of the related fat-containing food. Among the three PMF groups, PMF-A (13.2 h) and PMF-B (11.2 h) exhibited higher OSIs compared to that of PMF-C (8.8 h). The results are in agreement with those of micronutrient levels mentioned above.
When individual micronutrients were fitted into the OSI model, the best highly significant variable were γ-tocopherol and campesterol (P < 0.05) as shown in . In this regard, they are the main factors that affected the oxidative stabilities of PMFs. Similar to γ-tocopherol, campesterol also shows high oxidative activities at industrial frying operations. Dutta et al.[Citation39] found that epoxides of both sitosterol and campesterol were the major contributors in terms of total sterol oxides in the fries prepared in rapeseed oil and palm oil blend. Similarly, although β-sitosterol was the most abundant sterol in PMFs, Salta concluded[Citation40] that its survived percentages after deep or pan frying were higher than those of campesterol, also suggesting the higher activities of campesterol during frying. The result indicates that PMF-A and PMF-B containing 109–158 mg/kg of campesterol are more suitable for the manufacture of frying fats compared to PMF-C, whose campesterol level was not detected by the current method.
Table 4. Multiple linear regression analyses between oxidative stability indexes and micronutrient levels (tocopherol and sterol).
Conclusion
Significant differences in fat, triacylglycerol compositions and micronutrient levels were found in PMF-A, PMF-B and PMF-C, resulting their different thermal behaviors and oxidative stabilities. PMF-A showed soften properties in terms of SMP, DSC and SFC analyses, while PMF-B was characterized as hard fat. Their SFC profiles were comparable to those of frozen and puff margarine shortenings. In addition, both of PMF-A and PMF-B contained higher micronutrient levels (mainly including γ-tocopherol, α-tocopherol, β-sitosterol, and campesterol) and OSI values than those in PMF-C. γ-Tocopherol and campesterol are the most significant factors to prevent PMFs from oxidation according to the multiple linear regression analyses between OSIs and micronutrients compositions, and they generally exhibit excellent antioxidant capacities at frying conditions. Therefore, PMF-A and PMF-B are suggested to be used in manufacturing of margarine shortenings and frying fasts. In particular, PMF-B is more popular in hot weather. PMF-C is the further fraction of PMF-A or PMF-B. It contained the highest symmetrical monounsaturated triacylglycerol level (especially POP and POS) and showed steep SFC curve, which are preferred in producing heat-resistant chocolate fats.
Nomenclature
CB | = | cocoa butter |
CBE | = | cocoa butter equivalent |
IV | = | iodine value |
OSI | = | oxidative stability index |
PMF | = | palm mid-fraction |
POO | = | 1-palmitoyl-2,3-dioleoyl-glycerol |
POP | = | 1,3-dipalmitoyl-2-oleoyl-glycerol |
POS | = | 1-palmitoyl-2-oleoyl-3-stearoyl-glycerol |
PPP | = | 1,2,3-tripalmitoyl-glycerol |
SFC | = | solid fat content |
SMP | = | slip melting point |
SOS | = | 1,3-distearoyl-2-oleoyl-glycerol |
FID | = | flame ionization detector |
Additional information
Funding
References
- Marc, K.; Véronique, G.; Marc, H.; Wim, D. G. Palm Oil Fractionation. European Journal of Lipid Science & Technology. 2010, 109, 336–349.
- Food and Agriculture Organization of the United Nations (FAO) Statistics Division. 2014. http://www.fao.org/faostat/en/#data/QD/visualize (accessed July 28, 2017).
- Official Journal of the European Communities. Directive of 23 June 2000 Relating to Cocoa and Chocolate Products Intended for Human Consumption; Directive 2000/36/EC, 19, L197, 2000.
- Beckett, S. T.; The Science of Chocolate; Royal Society of Chemistry: York, 2000, 102–170.
- Danthine, S.; Lefébure, E.; Blecker, C.; Dijckmans, P.; Gibon, V. Correlations between Cloud Point and Compositional Properties of Palm Oil and Liquid Fractions from Dry Fractionation. Journal of the American Oil Chemists Society. 2017, 94, 841–853. DOI: 10.1007/s11746-017-2990-2.
- Gijs, C.; Véronique, G.; Wim, D. G.; Laurence, P.; Imogen, F.; Koen, D. Phase Composition during Palm Olein Fractionation and Its Effect on Soft PMF and Superolein Quality. Journal of the American Oil Chemists Society. 2007, 84, 885–891. DOI: 10.1007/s11746-007-1118-5.
- Kumar, P. K. P.; Krishna, A. G. G. Physico-Chemical Characteristics and Nutraceutical Distribution of Crude Palm Oil and Its Fractions. Grasas Y Aceites. 2014, 65, e018. DOI: 10.3989/gya.2014.v65.i2.
- Kang, K. K.; Kim, S.; Kim, I. H.; Lee, C.; Kim, B. H. Selective Enrichment of Symmetric Monounsaturated Triacylglycerols from Palm Stearin by Double Solvent Fractionation. LWT-Food Science and Technology. 2013, 51, 242–252. DOI: 10.1016/j.lwt.2012.08.009.
- Jin, J.; Pembe, W.; Qi, C.; Sun, C.; Jie, L.; Xie, D.; Huang, J. H.; Jin, Q. Z.; Wang, X. G. Mango Kernel Fat Based Chocolate Fat with Heat Resistance Triacylglycerols: Production via Blending Using Mango Kernel Fat Mid-Fraction and Palm Mid-Fractions Produced in Different Fractionation Paths. Rsc Advances. 2016, 6, 108981–108988. DOI: 10.1039/C6RA19438A.
- Xia, Y.; Study on the compability of lard and palm oil and its application. Master thesis, Jiangnan University, 2014. (In Chinese).
- Bootello, M. A.; Hartel, R. W.; Garcés, R.; Martínez-Force, E.; Salas, J. J. Evaluation of High Oleic-High Stearic Sunflower Hard Stearins for Cocoa Butter Equivalent Formulation. Food Chemistry. 2012, 134, 1409–1417. DOI: 10.1016/j.foodchem.2012.03.040.
- Gibon, V.; Tirtiaux, A. Latest Trends in Dry Fractionation. Lipid Technology. 2002, 3, 33–36.
- Jin, J.; Wang, Y.; Su, H.; Pembe, W.; Xie, D.; Liu, Y. J.; Wang, X. S.; Huang, J. H.; Jin, Q. Z.; Wang, X. G. Oxidative Stabilities of Mango Kernel Fat Fractions Produced by Three-Stage Fractionation. International Journal of Food Properties. 2016. DOI: 10.1080/10942912.2016.1253096.
- Gunstone, F. D.;. Vegetable Oils in Food Technology Composition, Properties and Uses, 2nd ed; Wiley: New York, 2011, 25–58.
- Zeng, C. X.; Qi, S. J.; Xin, R. P.; Yang, B.; Wang, Y. H. Enzymatic Selective Synthesis of 1,3-DAG Based on Deep Eutectic Solvent Acting as Substrate and Solvent. Bioprocess and Biosystems Engineering. 2015, 38, 2053–2061. DOI: 10.1007/s00449-015-1445-0.
- AOCS. Official Method and Recommended Practices of the American Oil Chemists’ Society, 5th ed; AOCS Press: Champaign, IL, 1998.
- Luddy, F. E.; Barford, R. A.; Herb, S. F.; Magidman, P.; Riemenschneider, R. W. Pancreatic Lipase Hydrolysis of Triglycerides by a Semimicro Technique. Journal of the American Oil Chemists Society. 1964, 41, 693–696. DOI: 10.1007/BF02661412.
- Shi, C.; Chang, M.; Liu, R. J.; Jin, Q. Z.; Wang, X. G. Trans-Free Shortenings through the Interesterification of Rice Bran Stearin, Fully Hydrogenated Soybean Oil and Coconut Oil. International. Journal of Food Engineering. 2015, 11, 467–477.
- Li, C. M.; Yao, Y. P.; Zhao, G. Z.; Cheng, W.; Liu, H. L.; Liu, C. Y.; Shi, Z.; Chen, Y.; Wang, S. Comparison and Analysis of Fatty Acids, Sterols, and Tocopherols in Eight Vegetable Oils. Journal of Agricultural and Food Chemistry. 2011, 59, 12493–12498. DOI: 10.1021/jf203760k.
- Traitler, H.; Dieffenbacher, A. Palm Oil and Palm Kernel Oil in Food Products. Journal of the American Oil Chemists’ Society. 1985, 62, 417–421. DOI: 10.1007/BF02541414.
- Timms, R. E.; Choice of Solvent for Fractional Crystallisation of Palm Oil. International Conference on Palm Oil Product Technology in the eighties. Proceedings June 22-24 Kula Lumpur (No. L-0016), ISP, 1983.
- Ray, J.; Smith, K. W.; Bhaggan, K.; Nagy, Z. K.; Stapley, A. G. Crystallization and Polymorphic Behavior of Shea Stearin and the Effect of Removal of Polar Components. European Journal of Lipid Science & Technology. 2013, 115, 1094–1106.
- Wahnelt, S.; Meusel, D.; Tulsner, M. Influence of Isomeric Diglycerides on Phase Transition of Cocoa Butter-Investigation by Isothermal DSC. Fett Wiss Technol-Fat Science Technology. 1991, 93, 174–178.
- Ray, J.; Smith, K. W.; Bhaggan, K.; Nagy, Z. K.; Stapley, A. G. F. Characterisation of High 1,3-Distearoyl-2-Oleoyl-Sn-Glycerol Content Stearins Produced by Acidolysis of High Oleic Sunflower Oil with Stearic and Palmitic Acids. European Journal of Lipid Science & Technology. 2014, 116, 532–547. DOI: 10.1002/ejlt.201300282.
- Jahurul, M. H. A.; Zaidul, I. S. M.; Norulaini, N. A. N.; Sahena, F.; Abedin, M. Z.; Ghafoor, K.; Omar, A. M. Characterization of Crystallization and Melting Profiles of Blends of Mango Seed Fat and Palm Oil Mid-Fraction as Cocoa Butter Replacers Using Differential Scanning Calorimetry and Pulse Nuclear Magnetic Resonance. Food Research International. 2014, 55, 103–109. DOI: 10.1016/j.foodres.2013.10.050.
- Sonwai, S.; Kaphueakngam, P.; Flood, A. Blending of Mango Kernel Fat and Palm Oil Mid-Fraction to Obtain Cocoa Butter Equivalent. Journal of Food Science and Technology. 2014, 51, 2357–2369. DOI: 10.1007/s13197-012-0808-7.
- Arishima, T.; Sagi, N.; Mori, H.; Sato, K. Polymorphism of POS. I. Occurrence and Polymorphic Transformation. Journal of the American Oil Chemists Society. 1991, 68, 710–715. DOI: 10.1007/BF02662157.
- Jeoungmae, S.; Kiteak, L.; Casimirc, A.; Meeree, K.; Mijung, K.; Jeunghee, L. Optimisation of Tripalmitin-Rich Fractionation from Palm Stearin by Response Surface Methodology. Journal of the Science of Food & Agriculture. 2010, 90, 1520–1526. DOI: 10.1002/jsfa.3978.
- Saadi, S.; Ariffin, A. A.; Ghazali, H. M.; Miskandar, M. S.; Boo, H. C.; Abdulkarim, S. M. Application of Differential Scanning Calorimetry (DSC), HPLC and pNMR for Interpretation Primary Crystallisation Caused by Combined Low and High Melting TAGs. Food Chemistry. 2012, 132, 603–612. DOI: 10.1016/j.foodchem.2011.10.095.
- Smith, K. W.; Bhaggan, K.; Talbot, G. Phase Behavior of Symmetrical Monounsaturated Triacylglycerols. European Journal of Lipid Science & Technology. 2013, 115, 838–846. DOI: 10.1002/ejlt.v115.8.
- Ribeiro, A. P. B.; Basso, R. C.; Grimaldi, R.; Gioielli, L. A.; Gonçalves, L. A. G. Effect of Chemical Interesterification on Physicochemical Properties and Industrial Applications of Canola Oil and Fully Hydrogenated Cottonseed Oil Blends. Journal of Food Lipids. 2009, 16, 362–381. DOI: 10.1111/j.1745-4522.2009.01152.x.
- Jahurul, M. H. A.; Zaidul, I. S. M.; Norulaini, N. A. N.; Sahena, F.; Abedin, M. Z.; Mohamed, A.; Omar, A. M. Hard Cocoa Butter Replacers from Mango Seed Fat and Palm Stearin. Food Chemistry. 2014, 154, 323–329. DOI: 10.1016/j.foodchem.2013.11.098.
- Sue, T. T.;. Pocketbook of Palm Oil Uses, 6th ed; Malaysian Palm Oil Board: Malaysian, 2009, 45–109.
- Ghotra, B. S.; Dyal, S. D.; Narine, S. S. Lipid Shortenings: A Review. Food Research International. 2002, 35, 1015–1048. DOI: 10.1016/S0963-9969(02)00163-1.
- Hamm, W.; Hamilton, R. J.; Calliauw, G. Edible Oil Processing, 2nd ed; John Wiley & Sons: West Sussex, 2013, 3–16.
- Wang, X. G.; Jin, Q. Z. Oleo Chemistry. Science Press: Beijing, 2012, 125–135. In Chinese.
- Kanno, C.; Hayashi, M.; Yamauchi, K.; Tsugo. T. Antioxidant Effect of Tocopherols on Autoxidation of Milk Fat. Agricultural and Biological Chemistry. 1970, 34, 878–890.
- Ayyildiz, H. F.; Topkafa, M.; Kara, H.; Sherazi, S. T. H. Evaluation of Fatty Acid Composition, Tocols Profile, and Oxidative Stability of Some Fully Refined Edible Oils. International Journal of Food Properties. 2015, 18, 2064–2076. DOI: 10.1080/10942912.2014.962657.
- Dutta, P. C.; Appelqvist, L. A. Sterols and Sterol Oxides in the Potato Products, and Sterols in the Vegetable Oils Used for Industrial Frying Operations. Grasas Y Aceites. 1996, 47, 38–47. DOI: 10.3989/gya.1996.v47.i1-2.841.
- Salta, F. N.; Kalogeropoulos, N.; Karavanou, N.; Andrikopoulos, N. K. Distribution and Retention of Phytosterols in Frying Oils and Fried Potatoes during Repeated Deep and Pan Frying. European Food Research and Technology. 2008, 227, 391–400. DOI: 10.1007/s00217-007-0733-6.