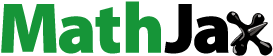
ABSTRACT
In this research, the behavior of orange fruit under impact cases was investigated by finite-element modeling in SolidWorks simulation and experimental dropping tests. The values of 0.584 MPa and 0.24 were experimentally determined for modulus of elasticity and Poisson’s ratio as a pre-requirement for simulation. In the simulation tests, orange fruits were dropped from two different heights of 2 and 3 m with a drop angle of 45° relatives to a rigid plane. Experimental tests were also conducted to validate simulation results. Maximum equivalent stress and contact force were obtained from simulation results 0.5826 MPa and 14.95 N, respectively.
Introduction
Orange fruits due to vitamin C, minerals, as well as fresh consumption, have an important position in agricultural products and producing countries gain substantial income by exporting them. Orange fruits have been cultivated in Iran from about 600 years ago, first in the southern coast and later in some other regions. Iran produces 4% of the world’s orange fruit and generates more than 2733,000 t annually.[Citation1] It is essential having sufficient knowledge of the physical and mechanical properties of orange fruit for harvesting. Mechanical damage during harvest and postharvest processing of agricultural products is causing physiological changes. These damages reduce the quality and thus the decay of the fruits. Mechanical impacts are known as a major factor of the crop’s postharvest losses.[Citation2] Although some bruising results do not appear instantly, they can contribute to decreasing quality of the fruit within a short period.[Citation3–Citation5] Predictions of the impact areas, deformation, and stress distribution in the fruit are important issues in postharvest studies. Direct measurement of the impact effects, which are caused by external forces, is very difficult. Today, the development of computer technology with the availability of the finite-element methods (FEM) which can be a useful tool to predict the mechanical behaviors of fruits is employed to solve complicated problems using computer-aided engineering technology without need to physical tests.[Citation6]
There are many researches, which have investigated the level of damage, stress distribution, and reaction of the fruits under the external forces using FEM.[Citation7–Citation9] For example, deformation behavior of the tomato fruit was investigated upon crashing.[Citation10] In this study, at the first, a 3D model of tomato was generated in CATIA software and then in order to find mechanical properties of tomato in compression tests, simulated using FEM. A comparison between experimental data and finite element simulation confirmed that the two sets of results agreed well,[Citation6] utilized a 3D scanner, high-speed camera, and FEM simulations to investigate drop case deformation of a Golden Delicious apple. They validated the results of SolidWorks simulation (drop test) by high-speed camera and observed that visual investigations and simulation results agreed well.
Bruise susceptibility of Pear under various impact cases were experimentally determined and modeled using FEM by.[Citation7] Three impact heights (0.2, 0.5, and 1 m), two impact surfaces (steel and wood), two impact orientations of the fruit (vertical and horizontal), and three ripeness level (unripe, ripe, and overripe) were considered in the impact simulation scenarios. The lowest and the highest errors between the predicted and observed bruised areas calculated by the ANSYS software were 0.00% and 60.53%, respectively. Comparing experimental and modeling data, a good agreement was observed in the case of pears dropped from 0.2 and 0.5 m heights. These studies indicate the potential of FEM as a powerful tool to investigate deformation behavior of the agricultural crops particularly fruits and vegetables.
For simulation of orange fruit using FEM, it needs to know some mechanical properties of the fruit such as modulus of elasticity and Poisson’s ratio, which are required as two fundamental properties of elastic and viscoelastic solids for calculation of stress and elastic deformation.[Citation11,Citation12] Based on previous researches, Poisson’s ratio in the organic materials is a function of moisture content, stress, and loading rate[Citation13,Citation14] and in terms of theory, it changes from zero to half. Poisson’s ratio is the ratio of transverse strain to longitudinal strain along the direction of applied force. In the contact problems, modulus of elasticity is a more effective parameter than Poisson’s ratio.[Citation11]
There are several reports about calculation of the Poisson’s ratio and modulus of elasticity of fruits and vegetables in the published literatures. Kiani deh Kiani et al.[Citation15] obtained Poisson’s ratio and modulus of elasticity of two varieties of red bean grains in different moisture contents and loading rates. They used the instrumented-bending beam as a contact sensor for transverse strain measurement and Hertz theory for determination of the modulus of elasticity. Poisson’s ratio and modulus of elasticity decreased with increasing moisture content and increased when loading rate increased. Cakir et al.[Citation16] determined the modulus of elasticity and Poisson’s ratio of two varieties of onion. In this study, determination of the transverse strain was measured by linear variable differential transformer regardless of crop geometry. Pallottino et al.[Citation17] also obtained the modulus of elasticity and Poisson’s ratio of orange fruits using universal testing machine, which equipped with upper and lower plates and three cameras along three orthogonal axis lines. Hassanpour et al.[Citation18] also used the Hertz theory and image processing tool to calculate the modulus of elasticity and Poisson’s ratio of white seedless grapes.
According to the literature review, the object of this study was to simulate the free fall motion of orange fruit using finite element analysis (FEA) in SolidWorks simulation and validate the software outputs by experimental laboratory tests. Modulus of elasticity and Poisson’s ratio of orange fruits were also determined as a requirement inputs for drop test simulation.
Material and methods
A 3D solid model
In this study, an orange fruit was used as a biological material to study deformation behavior under drop case. A 3D solid model of the orange was created by SolidWorks software on the basis of dimensions which determined from actual samples. Required samples for the experimental tests were collected in early October 2016. Equatorial diameter (De), polar diameter (Dp), and thickness (T) of samples were measured by a digital caliper with an accuracy of 0.05 mm. Polar diameter is the distance between the fruit base and the point of stem attachment to the fruit. Equatorial diameter is the maximum width of the orange fruit in a plane perpendicular to the polar diameter. Some physical and mechanical properties of the samples are shown in . Shape index is used to evaluate the shape of oranges, and it is determined according to the Equation 1. If the shape index is larger than 1.5, the sample is considered as an oval and if the shape index is smaller than 1.5, it is considered as spherical.[Citation18]
Table 1. Some average value of physical and mechanical properties of orange samples.
Modulus of elasticity
A universal testing machine was employed in order to determine the modulus of elasticity of orange fruits in quasi-static compression tests. Samples were loaded between two parallel plates of machine (Fig. 1a) by the rate of 10 mm min−1.[Citation19] During the experiments, the universal testing machine generated force graph as a function of deformation. Force exertion stopped when initial failure detected as a sharp reduction in the value of force (Fig. 1b). Modulus of elasticity for a whole fruit, when it is compressed through parallel plates, was determined based on ASAE standards for compression test of food materials of convex shape[Citation19]:
where is the modulus of elasticity (MPa),
is the deformation (mm),
is the Poisson’s ratio (dimensionless),
is the exerted force (N), the constants
and
dimensionless parameters related to geometrical characteristics of the bodies in contact with each other.
,
are radii of curvature of the convex surface of the sample at the point of contact with the upper plate (m),
,
are radii of curvature of the convex surface of the sample at the point of contact with the lower plate (m). According to the sample dimensions and the shape index value (), the orange fruit assumed as a spherical object. So, upper and lower radii of curvature are equal to constant K (
)[Citation20]
So, Equation 2 converted to Equation 4 for a spherical object.
Volume measurement
Orange volume determined using the fluid displacement technique. Water was used as a fluid due to the lack of water absorption by orange peel. According to water density,[Citation1] the sample volume is equal to the mass of water which is moved by the immersion of sample in water.[Citation12] Water was used at environment temperature (about 20°C), at which the density is near to .
Poisson’s ratio determination
Poisson’s ratio was determined by loading samples between parallel plates along the polar diameter in the universal testing machine, which was equipped with a 50 N load cell. At the first, the upper plate was placed in contact with the fruit without applying any pressure, and a digital image was captured using Sony DSC-W200 camera at this situation. Then, after loading the sample with a standard rate of 10 mm min−1, another image was captured and saved with jpg format. A program code was written in MATLAB (R2015b) for image processing and the initial image (before loading) uploaded in order to determine the longitudinal and transverse distance. Each of the images was calibrated with a ruler in the background () and then, the margin of image was removed for the rising of the points selection accuracy (). After that, the highest and lowest points of sample in tangency with parallel plates were selected (). So, the program measured and indicated the longitudinal distance between these two points as output (). The transverse distance for the sample also obtained in a similar way. Similar method was applied on the final image to calculate longitudinal and transverse deflection after loading and then, Poisson’s ratio (μ) was obtained by Equation (5).
Figure 1. Quasi-static compression test of orange fruit using parallel plates: (a) before loading, (b) after loading.
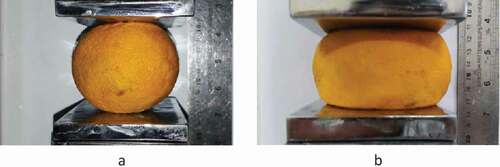
Figure 2. Measurement steps of Poisson’s ratio in MATLAB: (a) the length calibration by ruler, (b) image margin elimination, (c) point selection, and (d) longitudinal and transverse distance measurement.
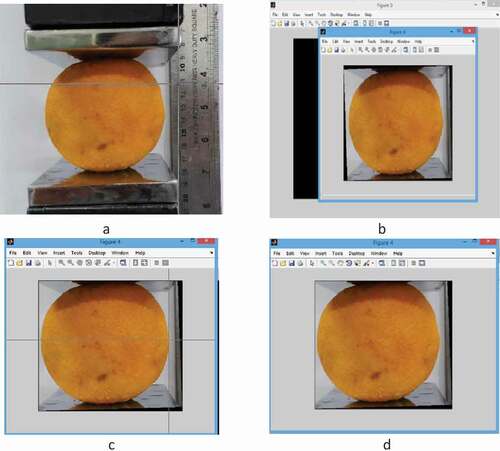
where is the lateral strain and
is the longitudinal strain.
Finite element principles and mesh model
FEA is an advanced engineering tool, which can use in simulation and analyzing agricultural products under a variety of conditions and replace experimental testing. In this process after the creation of a geometric model, the program subdivides the model into small pieces of simple shapes (elements) which connected at common points (nodes). FEA programs dealing with the model as a network of discrete interconnected elements.
In this study, investigation of free fall motion of the orange fruit as a nonrigid object was done using drop test Analysis (available in Solidworks Simulation). Nonlinear vibration analysis intended for drop test simulation. After defining the necessary material properties of orange fruit (density, elasticity modulus, and Poisson’s ratio), mesh generation (break the model into small elements) was performed. Meshing is a very crucial step in design analysis. For the analysis of orange sample, 3D parabolic tetrahedral solid elements were used (). Solid elements are naturally suitable for bulky models. Parabolic tetrahedral elements (also called second-order or higher order elements) which yield better results were selected due to more accurate representation of curved boundaries and better mathematical approximations than linear elements. Mesh construction of the model by using solid mesh (element size: 6.7 mm and mesh quality: high) was containing 10,393 elements and 12,050 nodes ().
Figure 3. (a) Schematic drawings of parabolic tetrahedral solid elements, (b) mesh construction of the orange sample in SolidWorks.
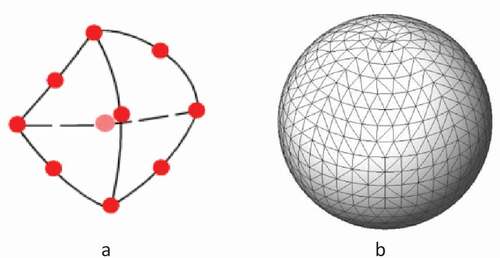
The next step after meshing and applying material to the model is specifying the drop test settings. The drop height (h), the acceleration of gravity (g), and the orientation of the impact plane must be defined for analyzing the free fall motion of orange fruit. In this study, sample was dropped on the ground from the heights of 2 and 3 m under the earth’s gravitational effect with an angle of 45° relatives to the rigid surface (the worst drop cases)(). Therefore, the program calculates the velocity (V) at the moment of collision with the ground surface from the following equation:
where g is the acceleration of gravity (m s−2) and h is the drop height (m). Finally, simulation scenarios were run after completion of the preprocessor steps, and the results were recorded. SolidWorks solves the dynamics issues as a function of time. The general equation of motion is as follows:
where ,
,
, and
, which include gravitational forces and impact forces. All of these forces are time dependent.[Citation21] The program uses an explicit time integration method to solve drop test studies. It automatically estimates the critical time step based on the smallest element size and uses a smaller value to prevent divergence. The program calculates the response starting from the moment of the first impact. It estimates a value for the solution time based on the model geometry and material properties. The default solution time is based on the traveling time of elastic wave through the model which generated by the impact. The velocity (
) of the elastic wave can be estimated from the following equation:
where and ρ = density (kg cm−3). The wave starts propagating in the model at the moment of impact. In order to estimate reasonable period, assuming that the length of the model is L, the wave takes a time period approximately equal to
to travel to the farthest boundary, get reflected, and travel back to the originating area. Due to existence of the opposite forces to gravity which start acting on the model during this period, the program sets the default solution time to
.
Experimental method
To verify the accuracy and correctness of the simulation results obtained from SolidWorks software, the experimental dropping tests were conducted. For this purpose, 20 ripe and fresh oranges with almost the same size and weight were randomly bought from the market. For measurement of the maximum force of impact, an axial load cell (Sokki Kenkyujo, Japan) under the rigid plate was used which transmitted (TM-1020 transmitter, Tika, Iran) data to the computer with connecting cable () and then recorded them by the software. Samples dropped from two heights of 2 and 3 m with 45° drop angle on the rigid plate. In order to determine the stress value, it is needed to know the sample contact area with the rigid plate, which was stamped after impact by fresh painting color on the rigid plate (). The painted area was measured using an image-processing algorithm in MATLAB software and then, stress was obtained by the following equation:
Figure 5. (a) Experimental dropping test setup: (1) axial load cell, (2) rigid plate connected to the load cell, and (3) and transmitter. (b) Determination of contact area for stress calculation after impact.
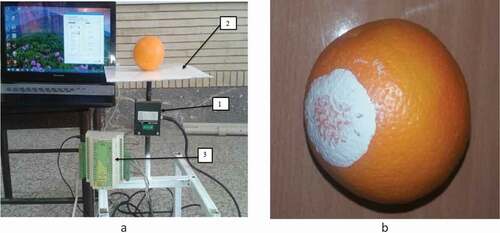
where is the stress (kPa),
is the contact force (N), and
is the contact area (cm2).
Results and discussion
Some of the physical and mechanical properties of the orange fruit under abovementioned condition indicate in to use in the FEM simulation. As the previous studies emphasized, the simulation results and bruise susceptibility directly depend on some mechanical properties of sample such as elastic modulus and Poisson ratio.[Citation6,Citation22] The Modulus of elasticity and Poisson’s ratio obtained in this study have good compatibility with the results reported by Ref. [Citation23] but observed some difference with Pallottino et al. results which can be interpreted by different variety and therefore different texture of fruits.[Citation17]
The orange impact was considered and it was simulated through nonlinear FEM-based explicit dynamics simulation approach. In the scenarios, the orange was allowed to make free-fall impact on a rigid plate from predefined heights under the acceleration of gravity. After completion of the simulation preprocessor steps, simulation scenarios were run and useful numerical and visual results include equivalent stresses and contact forces. In total, 22 steps after impact were set after the first impact moment in the simulation for the drop case. Time-dependent stress progression and distribution on the orange body during predefined impact events were demonstrated using stress plot.
Contact force and stress magnitudes for the 2-m dropping height
indicates contact force and equivalent stress (Von Mises) diagram for the orange sample under drop case from height of 2 m. Numerical results revealed that the maximum impact force and equivalent stress (Von Mises) exerted to the simulated sample when dropped from height of 2 m were equal to 11.1 N and 47.719 kPa, which occurred in the step 4 of the analysis steps.
The value of contact force at the moment of impact was equal to 2.1 N (Fig. 6). This value keeps its increasing trend until the sample separates from the rigid plate and after that, contact force was reduced to zero at step 9 as there is no contact with the rigid plate at this step. Also at the moment of sample collision with the flat platform, the value of equivalent stress was 21.84 kPa. Then, this value increased until it reaches its maximum value (47.719 kPa) in the step 4. After that, when the orange starts to bounce as a reaction to the rigid plate, according to the elastic force, the stress reduced until it gets to its minimum value. Although there is no contact force existing after step 9, there are instances of stress observable, ranging from the value 0.29 to 0.67 kPa. In this study, the regions where stress were beyond the bio-yield stress can be described as bruised regions of the orange fruit and can be used for estimation of bruised volume under impact loads.
indicates the equivalent stress (Von Mises) distribution for six selected steps from solving steps after impact. At step 1 (75 µs after impact), the first deformation and stress values occurred. This stress value increased until it reaches its maximum value (at step 4 as shown in , and then due to elastic force, sample gradually starts to bounce and the stress value in the steps c, d, e, and f reduced. The maximum equivalent stress value obtained at the time of 300 µs (step 4). The results of this research were similar to previous research in that FEM simulations was utilized to investigate drop case deformation events of a Golden Delicious apple as the sample organic material.[Citation6]
Contact force and stress magnitudes for the 3-m dropping height
shows contact force and equivalent stress diagram for the sample under drop case (drop height of 3 m). In comparison with , contact force and equivalent stress for height of 3 m have higher magnitude than that of 2 m height (higher height, larger magnitude of contact force, and equivalent stress). At the first step after impact, the value of contact force was 3.15 N, which was increased until it reached its maximum value at step 4 after impact (14.14 N). After that, force value decreased until contact between the fruit and the platform was broken (step 9) and this value goes back to zero. The value of equivalent stress at this height was obtained 26.34 kPa at step 1 after impact and reached its maximum value at step 4 (55.29 kPa). After rebound, due to plastic work produced by deformation, the stress values did not go back to zero values and there are instances of stress observable, ranging from the value 0.39 to 0.74 kPa.
indicates the equivalent stress (Von Mises) distribution in the 6 nonconsecutive steps from 20 solving steps after impact. At the first step (75 µs after impact), due to the impact forces, the first values of deformations appeared at the collision point and consequently stresses observed in the sample (). The stress values had an increasing trend until it got its maximum magnitude as shown in , and after that when sample was getting away from the rigid plate, the stress magnitudes was decreased in the next steps (–). The maximum value of stress occurred at step 4, 300 µs after impact. It can be observed that the higher the height of the fall of the fruit, the more contact force and stress are exerted to the fruit. Dropping from these heights causes bruise susceptibility and time-dependent nonlinear deformation at the impact point. Therefore, it is recommended that during harvesting, handling, transportation, and storage, try to minimize the external force on the product due to its soft texture in order to deliver a healthier product to consumers. The results of this study were consistent with the research on apples and pears.[Citation6,Citation9]
Experimental measurement of contact force and normal stress
In order to validate the results of FEM simulation data, experimental dropping tests were performed from the heights of 2 and 3 m with 10 replication and then, measured contact force and normal stress values were recorded (). Contact force was directly measured by the load cell and normal stress was calculated by the ratio of contact force to the contact surface with the plate attached to the load cell. The average value of contact force and normal stress was obtained 11.32 N and 46.53 kPa for the height of 2 m and 14.17 N and 55.08 kPa for the height of 3 m. In all tests, contact force and normal stress values were increased with increasing dropping height, which was consistent with the results reported for drop test simulation of a tomato.[Citation21]
Table 2. The values of contact force, normal stress, height, and contact surface in the experimental tests.
The values of contact force and equivalent stress (Von Mises) exerted to the sample in simulation versus experimental test are presented in that shows the two sets of results agree well. Evaluation of the difference between experimental data and finite element simulation was performed using a one-sample t-test in Minitab 16 software. However, a statistically significant difference was not observed between the means of FEM simulation and experimental tests (P < 0.05). For dropping heights of 2 and 3 m, there are 1.93% and 0.24% difference between contact forces and 4% and 0.38% difference between normal stresses, respectively. Therefore, it can be concluded that the simulation results have good agreement with experimental test results.
Table 3. A comparison between experimental data and finite element simulation.
The values of contact force and equivalent stress obtained in the study were lower than the critical values for these parameters. Therefore, the sample is far away from rupture conditions and is in the safety zone, but due to sensitive texture of agricultural crops, specially fruits and vegetables, as affected by mechanical impact, over the time some bruise damages may be appeared. So, it is necessary to try that the least amounts of external forces applied on crops during different agricultural processes such as harvest, transportation, and postharvest operations to avoid the occurrence of the bruise damages which force economic losses to the fruit industry.
Conclusion
Improvement of the ability of hardware and software allows engineers to solve complex problems using computer-aided design technology and numerical methods without making physical model or experimental tests. This study presents a practical and efficient way for modeling and analyzing the behavior of samples when dropping on a rigid plate in SolidWorks software and evaluates it with experimental tests. Using a rigid flat plate mounted on the load cell, and a transmitter, the magnitude of contact force and normal stress after impact was measured. For two dropping heights of 2 and 3 m, the maximum value of contact force and equivalent stress (Von Mises) exerted to the orange sample were obtained 11.1 and 14.14 N and 47.73 and 55.29 kPa, respectively, for the step 4 in the FEM simulation. Also, the value of contact force and normal stress obtained in experimental tests for similar dropping heights were 46.53 and 55.08 kPa and 11.32 and 14.17 N, respectively. When comparing the results of simulation with experimental test, good agreement was observed and simulation results were in an acceptable range. Experimental tests approved that the SolidWorks simulation can be used to predict the behavior of agricultural crops under drop case. The results of this study can be used as a foundation for further investigations of the mechanical parameters of agricultural crops by using FEM simulations. In addition, some other parameters such as bruise susceptibility can be determined by this practical, simple, and cost-effective method.
References
- FAO. Citrus Fruits Statistics Food and Agriculture Organization of the United Nations, Rome, 2016.
- Sitkei, G. Mechanics of Agricultural Materials; Elsevier: Sopran, Hungary, 1987.
- Li, Z.; Li, P.; Liu, J. Effect of Mechanical Damage on Mass Loss and Water Content in Tomato Fruits. Int Agrophys 2011, 25, 77–83.
- Topakci, M.; Celik, H. K.; Canakci, M.; Rennie, A.; Akinci, I.; Karayel, D. Deep Tillage Tool Optimization by Means of Finite Element Method: Case Study for a Subsoiler Tine. Journal of Food, Agriculture and Environment 2010, 8(2), 531–536.
- Du, C.-J.; Sun, D.-W. Learning Techniques Used in Computer Vision for Food Quality Evaluation: A Review. Journal of food engineering 2006, 72(1), 39–55. DOI: 10.1016/j.jfoodeng.2004.11.017.
- Celik, H. K.; Rennie, A. E.; Akinci, I. Deformation Behaviour Simulation of an Apple under Drop Case by Finite Element Method. Journal of Food Engineering 2011, 104(2), 293–298. DOI: 10.1016/j.jfoodeng.2010.12.020.
- Yousefi, S.; Farsi, H.; Kheiralipour, K. Drop Test of Pear Fruit: Experimental Measurement and Finite Element Modelling. Biosystems Engineering 2016, 147, 17–25. DOI: 10.1016/j.biosystemseng.2016.03.004.
- Salarikia, A.; Ashtiani, S.-H. M.; Golzarian, M. R.; Mohammadinezhad, H. Finite Element Analysis of the Dynamic Behavior of Pear under Impact Loading. Information Processing in Agriculture 2017, 4(1), 64–77. DOI: 10.1016/j.inpa.2016.12.003.
- Celik, H. K. Determination of Bruise Susceptibility of Pears (Ankara Variety) to Impact Load by Means of FEM-based Explicit Dynamics Simulation. Postharvest Biology and Technology 2017, 128, 83–97. DOI: 10.1016/j.postharvbio.2017.01.015.
- Mahmoodi-Eshkaftaki, M.; Maleki, A. Simulation of a Tomato Sample Using Finite Element Method. International Journal of Agriculture and Crop Sciences 2013, 5(16), 1739.
- Gentle, C.; Halsall, M. Measurement of Poisson’s Ratio Using Newton’s Rings. Optics and Lasers in Engineering 1982, 3(2), 111–118. DOI: 10.1016/0143-8166(82)90004-5.
- Mohsenin, N. N. Physical Properties of Plant and Animal Materials; Gordon and Breach, New York, 1986.
- Khazaei, J.; Mann, D. Effects of Temperature and Loading Characteristics on Mechanical and Stress-Relaxation Properties of Sea Buckthorn Berries. Part 2. Puncture Tests, Agriculture Engineering International: CIGR Journal, 2004.
- Finney, E. E. The Viscoelastic Behavior of the Potato, Solanum Tuberosum, under Quasi-Static Loading; Thesis-Agriculture Engineering Department, Michigan State University, 1963.
- Kiani Deh Kiani, M.; Maghsoudi, H.; Minaei, S. Determination of Poisson’s Ratio and Young’s Modulus of Red Bean Grains. Journal of Food Process Engineering 2011, 34(5), 1573–1583. DOI: 10.1111/jfpe.2011.34.issue-5.
- Cakir, E.; Alayunt, F.; Ozden, K. A Study on the Determination of Poisson’s Ratio and Modulus of Elasticity of Some Onion Varieties. Asian Journal of Plant Sciences 2002, 1(4), 376–378. DOI: 10.3923/ajps.2002.376.378.
- Pallottino, F.; Costa, C.; Menesatti, P.; Moresi, M. Assessment of the Mechanical Properties of Tarocco Orange Fruit under Parallel Plate Compression. Journal of Food Engineering 2011, 103(3), 308–316. DOI: 10.1016/j.jfoodeng.2010.10.029.
- Bahnasawy, A. H. Some Physical and Mechanical Properties of Garlic. International Journal of Food Engineering 2007, 3(6), 1136. DOI: 10.2202/1556-3758.1136.
- ASABE Standards; Standards Engineering Practices Data. Compression Test of Food Materials of Convex Shape. America Society of Agricultural and Biological Engineers (ASABE) 2008, 671–678: St. Joseph, MI, 2008.
- Hassanpour, A.; Esmaiili, M.; Modarres, M. A.; Rahmani, D. A.; Nasiri, M. Determination of Poisson’s Ratio and Modulus of Elasticity during Maturation of White Seedless Grapes. Iranian Food Science and Technology Research Journal 2011, 6(4), 308–316.
- Kabas, O.; Celik, H. K.; Ozmerzi, A.; Akinci, I. Drop Test Simulation of a Sample Tomato with Finite Element Method. Journal of the Science of Food and Agriculture 2008, 88(9), 1537–1541. DOI: 10.1002/jsfa.3246.
- Ragni, L.; Berardinelli, A. PH—Postharvest Technology: Mechanical Behaviour of Apples, and Damage during Sorting and Packaging. Journal of agricultural engineering research 2001, 78(3), 273–279. DOI: 10.1006/jaer.2000.0609.
- Ihueze, C. C.; Mgbemena, C. E. Design for Limit Stresses of Orange Fruits (Citrus Sinensis) under Axial and Radial Compression as Related to Transportation and Storage Design. Journal of the Saudi Society of Agricultural Sciences 2017, 16(1), 72–81. DOI: 10.1016/j.jssas.2015.02.005.