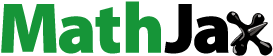
ABSTRACT
The current study compares the emulsifying properties of Persian gum (PG) and gum Arabic (GA) in emulsions. The effects of concentration (0.5–3% w/v PG, 2.5–15% w/v PG), pH (2–7), ionic strength [NaCl, CaCl2 (0–300 mM)], and temperature (40–90°C) were investigated. The surface–volume mean diameter (D32) of the emulsions showed that the minimum values were 9.89 ± 0.68 and 4.52 ± 0.03 µm for emulsions containing 1.5% w/v PG and 15% w/v GA, respectively. In addition, the zeta potential of PG and GA emulsion changed from −23.5 to −39.5 and −30.5 to −46.0 mV, respectively. The interfacial tensions of PG and GA emulsions were varied in the ranges of 34.0–15.0 and 29.0–9.0 mN/m, respectively. Changes in the D32 value of GA emulsions showed were not significantly different (p > 0.05) with respect to the effects of pH, NaCl, CaCl2, and temperature. The NaCl concentration had no significant effect on D32; but its value decreased from 13.11 to 5.70 µm as the CaCl2 concentration increased. The interfacial tension of PG significantly increased with decreasing pH 7–2 and increasing 0–300 mM salts. After heating (25–90°C), the D32 values of PG and GA emulsions changed to 11.04–14.54 and 4.21–4.21 μm, respectively. The results of this study can be useful for the application of PG as an emulsifier in beverage emulsions.
Introduction
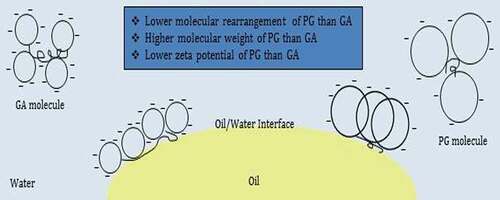
Among hydrocolloids, gum Arabic (GA), Tragacanth, Karaya, and Ghatti are isolated from plant exudates which have been used for thousands of years in the food and pharmaceutical products.[Citation5] GA, also known as Acacia gum (Acacia senegal and Acacia seyal), due to its excellent emulsifying properties is widely used as an emulsifier in emulsions. It is commonly used in beverage’s emulsions containing small amounts of oil.[Citation6] GA is expensive to be used in practice, because a rather high gum/oil ratio (~1:1) is required in order to produce fine stable emulsion droplets (D43≪1 µm).[Citation2] Due to the large amount of GA application and the relatively high cost, many scientists are trying to replace GA with other biopolymers.
PG is obtained from Amygdalus Scoparia spach tree exudate and also is known as Zedo, Shirazi, Farsi, and Angum gum. Depending on the amount of impurities, this gum exists in yellow, orange, and red colors. PG is an anionic and acidic hydrocolloid (e.g., the pH of 0.5 w/v % PG dispersion is 5.60 ± 0.05) having water soluble and insoluble parts.[Citation7–Citation9] It is consumed as a functional ingredient for nutritional and pharmaceutical purposes: appetite stimulant, crushing bladder stones, and so on. In recent years, several studies on physicochemical, rheological properties, emulsification, and also application of PG in probiotic yoghurt production and milk–orange juice mixture has been published.[Citation8–Citation14] We investigated the electrostatic complexion of β-lactoglobulin and PG in solution and oil-in-water emulsion systems.[Citation9] Also, the emulsifying properties of PG and β-lactoglobulin conjugates through the Maillard-type reaction and wet heating were improved.[Citation8,Citation15] Jafari et al.[Citation14] reported that increasing the GA level leads to a decrease in emulsion droplet size, while increasing the PG content results in bigger droplet sizes (no significant differences). In addition, droplet size distribution of PG-emulsified droplets at 2% PG and 5% d-limonene levels, had the lowest D32, D43, and the high specific surface area. Recently, Mirmajidi Hashtjin and Abbasi[Citation16] have reported that the soluble fraction of Persian and tregacanth gums (in combination) along with an emulsifier (Tween 80) could be used in the formation of nanoemulsions. In addition, the electrostatic interaction between water-soluble and water-insoluble fractions of Persian gum and milk proteins (β-lactoglobulin and whey protein isolate) were studied recently by several authors.[Citation17–Citation21]
Generally, food emulsions may encounter with a variety of environmental conditions (pH, ionic strength, heat treatment, and so on) during their processing, storage, transportation, and consumption. So, it is important for technologists to understand the impact of these factors on emulsion stability (ES). To our knowledge, the influence of environmental conditions on the emulsifying properties of PG has not yet been studied. Therefore, the objective of this study was to investigate the influence of gum concentration, pH, ionic strength (NaCl and CaCl2), and heat treatment on the droplet size distribution of PG and GA emulsions.
Materials and methods
Preparation of the PG powder
The PG sample was collected from the trunks and branches of Amygdalus scoparia Spach trees in Chenar-e Naz, Yazd province, Iran (August 2012). PG was manually sorted and lighter color samples were chosen to be ground with a hammer mill and were sieved (˂250 μm). PG powders were dissolved in deionized water (23°C ± 1°C) with gentle stirring (IKA® RH basic 2, Germany) for at least 2 h and then PG solution was stored 48 h at 4°C for complete hydration. The PG solution was then filtered through a filter cloth for removing impurities. Then, the filtrate was freeze dried at −40°C for 24 h (Dena Vacuum, Iran). The freeze dried powder was kept at 4°C until the experiments were performed.[Citation8,Citation9] The GA was purchased from Daejung (Shiheung, South Korea). Soya oil was purchased from the local supermarket in Isfahan, Iran. Other reagents used were of analytical grade.
Determination of moisture, protein, fat, ash, and mineral (Mg2+, Ca2+, Na+, and K+) content of PG and GA
The chemical compositions of PG and GA were measured using Official Methods of Analysis (AOAC). [Citation22] The moisture content was determined by oven drying at 105°C ± 1°C for 5 h. The protein content was determined by the Kejeldahl method (Velp, UDK 132, Italy) with a conversion factor of 6.25. Fat was measured by the Soxhlet extraction method using petroleum ether as solvent. To determine the total ash, samples were heated in electric ovens (at 550°C for 5 h). After calculating the total ash, it was used to analyze minerals (Na+, K+, Mg2+, and Ca2+). The residue from the ash content was dissolved in 12N HCl. Then, heated on a boiling water bath and filtrated with a Whatman filter (50 μm) after diluting with deionized water. The resulting extract was analyzed using a Perkin-Elmer® OptimaTM 7300DV ICP–OES instrument (Shelton, CT, USA) for the determination of the mineral content. The total carbohydrate percentage was calculated from the difference between all the previous factors from 100%. All experiments for chemical composition of PG and GA were performed in triplicate.
Preparation of PG and GA solutions
PG and GA solutions were prepared by moderately adding the gum powders in deionized water under constantly stirring with a magnetic bar (IKA® RH basic 2, Germany) for at least 2 h at room temperature (20°C ± 2°C). Then, the stock solutions were stored for at least 18 h at 4°C for complete hydration.
Emulsion, preparation, and effect of environmental conditions
Gum solutions were prepared with 0.5%, 1%, 1.5%, 2%, 2.5%, and 3% w/v PG and 2.5%, 5%, 7.5%, 10%, 12.5%, and 15% w/v GA. About 40 ml gum solution and 10 ml soya oil were homogenized using an Ultra-Turrax homogenizer (T18, IKA, Germany) at 15000 rpm for 2 min. After choosing the gum concentration with the least droplet size, the effect of other parameters [NaCl and CaCl2 (0, 50, 100, 150, 200, and 300 mM)], pH (Citation2, Citation3, Citation4, Citation5, Citation6 and Citation7) and heat treatment (40°C, 50°C, 60°C, 70°C, 80°C, and 90°C)] on the droplet size of emulsions were separately investigated. All environmental conditions (pH, NaCl, and CaCl2) were adjusted before homogenizing using HCl and NaOH 1 N and precise amounts of salts. For salt and thermal stability of emulsions, the pH of each gum solutions was adjusted to 4.50 ± 0.05. For the uniformity of gum solutions, samples were mixed using a magnetic bar for 30 min. In order to evaluate the effect of heat treatment, emulsions were kept at a special temperature for 30 min in a water bath and then cooled to 20°C ± 2°C.
Emulsion activity (EA) and emulsion stability (ES)
For the determination of EA, emulsions were centrifuged at 1300 g for 5 min. Then, EA was calculated using the following equation:
The ES was determined by heating the prepared emulsions at 80°C for 30 min in a water bath and then kept in an ice bath for 15 min, followed by centrifuging at 1300 g for 5 min. ES was calculated according to the above equation.[Citation23] For a creaming index (CI), the emulsions were stored in a refrigerator (4°C) for two weeks and CI was assessed using the former equation.[Citation24]
Apparent viscosity measurement of emulsions
A Brookfield viscometer (Brookfield RV, DVII, USA) equipped with a small sample adaptor (spindle no. 21) was applied to measure the apparent viscosity of emulsions at 25°C. For temperature adjustment, a water bath and a water circulation pump were used. Readings were taken at various rotational speeds.
Droplet size distribution of emulsions
The particle size was analyzed using static light scattering (Horiba, LA-930, Japan). The refractive indices of soya oil and water were 1.520 and 1.330, respectively. To avoid flocculation, the emulsions were diluted with 0.1% w/v SDS solution (1:100) prior to injection. Then, they were shacked to ensure the homogeneity. Dilute emulsions were added to unit cells until the transmittance reached between 60% and 70% of the water blank.
Zeta-potential measurements
The electrical charge (ζ-potential) of PG- and GA-stabilized emulsions were determined by an instrument equipped with electrophoresis and phase analysis light-scattering (PALS) measurements (Zetasizer ZEN3600, Malvern Instruments, Malvern, UK) and the reproducibility was verified by performing 10 repetitions. Emulsions were diluted to 1:100 with deionized water before analysis. These tests were conducted in duplicate.
Interfacial tension measurements
The effect of gum concentration on the interfacial tension was measured by Wilhelmy’s plate method. The interfacial tensions between gum solutions and soya oil were measured using an automatic thermostated digital tensiometer (Data physics, DCAT 11, Germany) at 25°C. The gum solution was carefully covered by the oil phase and left 3 min later. The force needed to pull the Wilhelmy plate from one phase to another is equal to the interfacial tension. All tests were performed in duplicate.
Microstructure of emulsions
One droplet of PG and GA emulsions was placed with a sampler between a microscopic slide and a cover slip and then photographed. In order to capture microstructures of the emulsions, an optical microscope (Nikon ECLIPSE E600) equipped with a digital camera was used.
Statistical analysis
Statistical analysis of the dada was carried out using the ANOVA procedure. In addition, least significant difference (LSD) values were computed with significance defined at p < 0.05. All data analysis was carried out using statistical program SAS (SAS Institute, 2003, version 9.1). All charts were drawn using Microsoft office Excel 2010.
Results and discussion
Chemical composition
demonstrates the chemical compositions of the PG and GA powders used in this study. The protein and ash values of GA were significantly (p ˂ 0.05) higher than that of PG. Elemental analysis of gum showed that GA had significantly higher calcium, potassium, and sodium contents than PG; but magnesium is not different (p ˃ 0.05). The protein and ash values of PG in this study were higher than the reported values for Zedo gum with different colors (protein = 0.1971–0.2141% and ash = 1.3624–1.6750%).[Citation7] Calcium and potassium of PG were lower than Zedo gum, but magnesium and sodium were higher than the values reported by Fadavi et al.[Citation25] The quantities of the measured minerals in this study are as follows: calcium˃magnesium˃sodium˃potassium. The protein and ash values of GA were lower and higher, respectively, than the reported values for gum Arabic. In the case of elemental, only Magnesium values of gum Arabic were higher in the previous study.
Table 1. Chemical composition of GA and PG.
PG had 87.17% carbohydrate content that is not significant (p ˃ 0.05) compared to GA (86.44%). The amount of the total carbohydrate of other gum exudate e.g., Prunus Cerasus (71.51%), Prunus cerasoides (73.72%), and Prunus armeniaca (66.89%) was lower than PG.[Citation26–Citation28] However, Prunus dulis (92.36%) gum exudates and Prunus amygdalus gum (98.4%) had a high carbohydrate content compared to PG.[Citation29,Citation30] Milani et al.[Citation31] and Yebeyen et al.[Citation5] reported 85.4% and 85.7% total carbohydrate for anghouzeh gum and Acacia gum exudate, respectively, that are approximately similar to carbohydrate values of PG and GA. The high carbohydrate content showed that the purity of the PG and GA was relatively high and consisting mostly of saccharides. It has also been reported that PG is an arabinogalactan polysaccharide.[Citation11] This different chemical composition of hydrocolloids may be related to the gum source, the growth conditions and age of trees, different purification methods, and time of exudation.[Citation27]
The FTIR spectrum of PG showed that all typical bonds and peaks are characteristics of polysaccharides. For example, the results revealed the presence of carboxylic groups in the PG structure (data not provided). It could be concluded that PG is a biopolymer containing protein which was also confirmed by chemical analysis. The FTIR spectrum of PG previously discussed.[Citation11]
ES of emulsions
It is possible to accelerate the coalescence phenomenon using centrifugation. Nevertheless, as chemical and biological changes may occur in the long-term storage, it cannot be a good test for indication of the ES during long time of storage. However, it is widely used for evaluating the ES to coalescence because of being fast and easy.[Citation32] Based on , the EA, ES, and CI of PG emulsions increased significantly (p < 0.05) with an increase in the PG concentration probably due to an increase in the viscosity and a decrease in the velocity of droplet movements. Although, Huang et al.[Citation33] demonstrated that the viscosity of the hydrocolloid emulsion was not the only factor affecting ES. The EA, ES, and CI values of PG emulsions show that PG0.5% and PG1% were significantly lower than those of the other PG emulsions. In terms of GA, a considerable effect (p > 0.05) was not observed in EA, ES, and CI of GA emulsions, due to the lower viscosity of GA solutions with increasing GA concentration.
Figure 1. EA, ES, and CI of PG- and GA-stabilized emulsions at different concentrations.
– For each parameter (EA, EC, and CI), similar letters for each gum are not significantly different at p < 0.05.
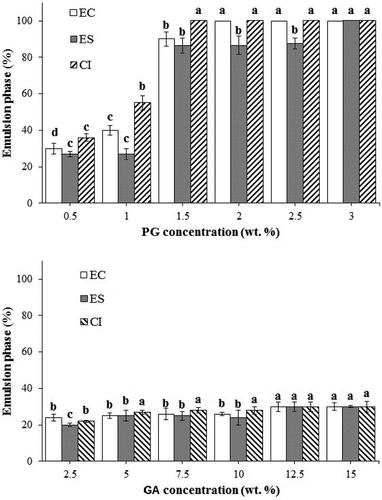
As can be seen in , ES is less than EA for all emulsions especially the ones with PG emulsions because the emulsions were held at high temperature (80°C, 15 min) for the stability test and the protective film around droplets might be destroyed, so the stability of emulsions was decreased. In fact, EA and ES tests showed that the surface activity is not the only factor determining the emulsion properties where polysaccharide is involved. The main contribution of polysaccharides to an emulsion is via water-holding and thickening properties. Similar results were observed by Wu et al.[Citation34] for the emulsifying properties of four commercial galactomannans (Fenugreek gum, Guar gum, Tara gum, and Locust Bean gum) and Mahfoudhi et al.[Citation30] for gum exudates from the trunk of the almond tree (Prunus dulcis).
CI is known to be related to the extent of the droplet aggregation in an emulsion. The CI values of the emulsions during two-week storage at 4°C revealed that PG emulsions at a concentration more than 1.5% w/v were completely (100%) stable and no creaming was observed. While GA even at the highest content (15% w/v) showed serum separation (). Therefore, PG emulsions were more stable compared to GA emulsions in terms of ES. Similar results were observed by Jafari et al.[Citation13] A high PG concentration would be increasing the viscosity of the intervening continuous liquid phase which restricts droplet movement preventing the approach and slows down the fluid draining between two droplets at the time of collision preventing re-coalescence.
Apparent viscosity of emulsion
The rheological properties of emulsions are important factors for considering the ES during storage because high viscosity conditions usually inhibit the coalescence of oil droplets. The apparent viscosity as a function of the shear rate is shown in . The hydrocolloid concentration had a direct positive effect on the apparent viscosity of emulsions. By increasing the PG and GA content, the apparent viscosity of all emulsions increased but these values for PG (2≥PG concentration) were higher than those of GA in all the studied range of shear rates. Compared to the reports on the Newtonian flow behavior of GA emulsions up to 7.5% concentration, PG showed a shear thinning behavior even at a concentration as low as 1% (W/W). These approved results were in agreement with the observations reported by Fadavi et al.[Citation11] In addition, Jafari et al.[Citation13] reported that PG emulsions had a higher apparent viscosity than their GA emulsion counterparts.
Figure 2. Apparent viscosity of PG- and GA-stabilized emulsions as a function of various shear rates.
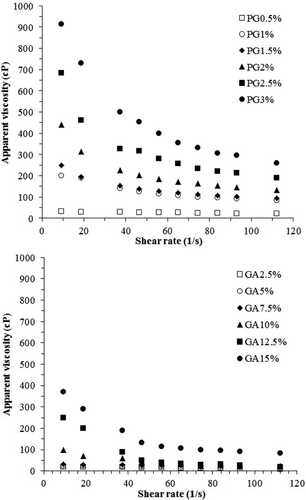
The apparent viscosity of the PG emulsions decreased with increasing shear rate and was found to exhibit a clearly pseudoplastic (shear-thinning) behavior. This pseudoplastic behavior is related to the breakage of droplet–droplet linkages that reached the Newtonian profile at higher shear rates. The hydrocolloid concentration dependency of the apparent viscosity of emulsions has been previously reported by some authors.[Citation35,Citation36] While GA did not have a significant impact on the apparent viscosity. For example, the 15% w/v GA emulsion had the same apparent viscosity as the 2% w/v PG emulsion ().
Effect of environmental conditions on droplet size, zeta potential, and interfacial tension emulsions
Effect of gum concentration
In general, it is important that emulsion droplets are made as small as possible to minimize the gravity creaming effect.[Citation2] Therefore, the aim of the initial studies on the emulsifying properties of gums was to find the relative efficiency of PG and GA in forming the emulsions during homogenization. In fact, we aimed to determine the minimum droplet size that could be produced by previous emulsifiers. Finally, we want to determine the minimum emulsifier content needed to attain a small droplet size. The effect of the gum concentration on the droplet size of PG and GA emulsions under the same homogenization conditions was evaluated which is shown in .
Figure 3. Cumulative droplet size distributions of the PG- and GA-stabilized emulsions at different gum concentrations.
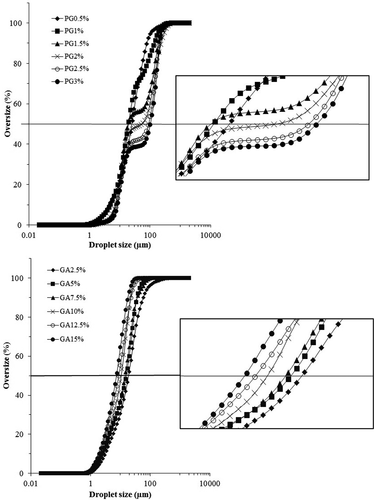
shows cumulative droplet size distributions of PG and GA emulsions (at various concentrations). In fact, the droplet size of emulsions where the cumulative distribution is 50% is known as the median droplet size (D0.5); therefore 50% of the droplets have a diameter less than that value. According to , the cumulative droplet size distribution curve shifts to left (smaller droplet size) with an increase in the GA content. For better illustration of this trend, the larger image of 50% cumulative frequency area was shown in front of . In addition, -A shows that the surface–volume mean diameter (D32) decreased significantly when the GA concentration increased (p < 0.05). Also, median (D0.5) values were decreased significantly (p > 0.05). Therefore, as can be seen in and , the GA emulsion containing 15% w/v gum had the least droplet size. It is reported in a previous study on the emulsifying properties of GA that 14% of GA is sufficient for covering the total area of the interfacial region of a 20% oil-in-water emulsion.[Citation37]
Figure 4. Effect of gum concentration on the mean diameter (D32) and median (D0.5) (A-1 and A-2), interfacial tension (B-1 and B-2), and zeta potential (C-1 and C-2) of PG (A-, B-, and C-1) and GA (A-, B-, and C-2) stabilized emulsions.
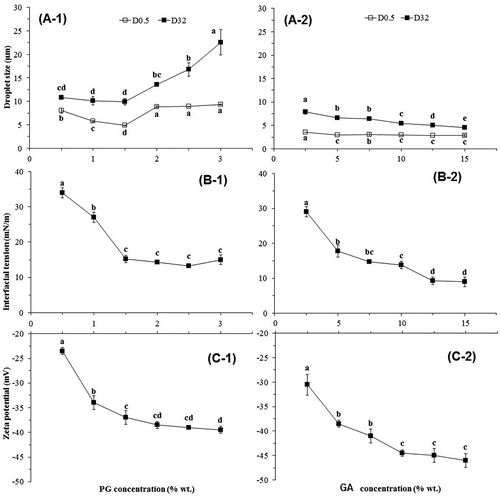
The droplet size distribution of PG emulsions with an increase in the PG concentration was assessed. Due to its higher viscosity enhancing PG, the effect of gum concentration on the droplet size of emulsions was evaluated in the range of 0.5–3% w/v PG concentration. As can be seen in , the cumulative droplet size distribution curve of PG emulsions shift to the left (smaller particle size) with an increase in the PG content from 0.5% to 1.5% w/v concentration. PG emulsions with more than 1.5% w/v PG had a bigger droplet size. -A shows that the D32 value decreased significantly (p < 0.05) with an increase in PG concentration (0.5–1.5% w/v) from 10.86 ± 0.13 to 9.89 ± 0.68 µm. Afterward, D32 of the PG emulsions increased significantly upon increasing the PG content from 1.5% to 3% w/v concentration. In addition, emulsion with 1.5% w/v PG concentration (p < 0.05) had the least D0.5 value (4.88 ± 0.03 µm) significantly. According to , the curve of the PG emulsions appear as an S-shaped curve from 1.5% to 3% w/v PG concentration, and inclined toward the larger droplets.
Typically, the dependence of the mean droplet size after homogenization on the initial emulsifier concentration can be divided into two regions: region I – the droplet size progressively decreases with increasing emulsifier concentration, because there is insufficient emulsifier present to cover all the newly created droplet surfaces; region II – the droplet size remains fairly constant, because the emulsifiers are more than enough to cover all of the droplets formed. In region I, the droplet diameter is limited by the total amount of emulsifier available to cover the oil–water interface formed, but in region II, the droplet diameter is limited by the maximum disruptive forces generated by the homogenizer.[Citation38] Jafari et al.[Citation14] reported that volume mean droplet size of 5% d-limonene-stabilized emulsion of PG increased by increasing the gum concentration in the range of 1–5% (D43 = 4.242–8.191 μm). In addition, Shao et al.[Citation39] reported that the mean droplet size of emulsions decreased until the Ulva faciata polysaccharide reached 3%, and after that resulted in a slight increase of the average droplet size of 4% polysaccharide.
The droplet size distribution of PG- and GA-stabilized emulsions show that GA is more effective than PG in making small oil droplets, which is in agreement with the faster kinetics of adsorption onto the oil droplets. The better capacity of GA may be due to its properties such as the number of hydrophilic and hydrophobic groups on the biopolymer chain (the different contents of arabino-galactan-proteins), molecular weight, and rate of absorption, conformational balance, and higher surface concentration.[Citation14] Moreover, the higher viscosity of the continuous phase can decrease the homogenization efficiency.[Citation1] However, the velocity of layer formation around an oil droplet is less at low emulsifier concentration, thus causing serious progress. Jafari et al.[Citation14] reported that the interesting result of higher PG contents (3% w/v) was that “over-processing” during emulsification was higher compared to a lower concentration (1.5% w/v) of PG. The interfacial activity of biopolymers is mainly controlled by the accessibility of the protein moiety of the interface and the net surface charge of adsorbing molecules. High-pressure homogenization treatment can be used for breaking some molecular association and making a higher proteinaceous fraction available to better emulsification properties.[Citation40]
It is noteworthy that droplet size distributions (volume percentage) of emulsions in all GA concentration were mono-peaked, while PG emulsions with more than 1.5% were bimodal (). Previously, bimodal distribution of PG emulsions was mentioned by Jafari et al.[Citation14] for d-limonene-stabilized emulsions (1–5% PG and 5–10% d-limonene). This trend in the cumulative droplet size distribution curve of the PG emulsion is shown in . Bimodal distributions was observed by Huang et al.[Citation33] for hydrocolloids such as xanthan and by Osano et al.[Citation41] for a new polysaccharide from basil seed (Ocimum bacilicum L.) at above 0.3% w/v gum concentration.
In general, hydrocolloids decrease the interfacial tension between oil and water phases and facilitate emulsion formation. The interfacial tensions of PG and GA solutions were influenced by the hydrocolloid concentrations. Based on , an increase in the concentration of both the hydrocolloids resulted in a significant decrease in the interfacial tension values. The interfacial tension values of PG and GA solutions were varied in the ranges of 34–15 and 29–9 mN/m, respectively. Both hydrocolloids had a protein part in their structure that is adsorbed into the oil-in-water interface and reduces the interfacial tension between two immiscible phases, thereby simplifying the disruption of emulsion droplets during the homogenization process. Osano et al.[Citation41], Vasile et al.,[Citation42] and Shao et al.[Citation39] found the ability of Basil seed gum, Prosopis alba, to exudate gum, and that of Ulva faciata polysaccharide in reducing the interfacial tension, respectively. The interfacial tension of PG solutions slightly increased at 3% concentration, possibly related to the interfacial activity measurement which became a problem due to viscosity effects, because it is very hard to pull the Du Nouy ring through the viscose PG solution. Huang et al.[Citation33] reported that hydrocolloids such as xanthan and carrageenan dramatically increased their interfacial tension at higher concentration.
The better hydrocolloid behavior in the stabilization of emulsions could be attributed to the negative charges in its molecular structure, mainly due to the presence of a high fraction of hydroxyl and carboxyl radicals. The combination of a high molecular weight, with an appropriate carbohydrate/protein ratio and predominantly negative charge, could be critical for improving the stability of disperse systems like emulsions.[Citation42] The role of electrostatic interactions in stabilizing the emulsions was examined by measuring the electrical charge of the droplets. The zeta potential values of the emulsions immediately after preparation are shown in -C. The results showed that PG- and GA-stabilized emulsions exhibited a very similar behavior in terms of variation in zeta-potential as a function of concentration. As shown in , zeta potential values of the PG-stabilized emulsions varied from −23.50 to −39.50 mV and were dependent on the PG concentration (0.5–3 w/v %). However, the zeta potential of GA emulsions was significantly lower than the ones obtained for PG (−30.50 to −46.00 mV for 2.5–15 w/v % GA-stabilized emulsions). The increase in the gum concentration leads to the increase in the negative surface charge of emulsion droplets and, consequently, negatively charged zeta potential which stabilizes the emulsion system. The lower negative charge of the PG molecules may be related to their uronic acid content. The zeta potential values for both PG and GA in all studied concentrations are below −30 mV (except 0.5% PG), which is the value that is usually indicated as the limit for significant droplet stabilization by electrostatic repulsion.[Citation43] Similar to most gum exudate, the natural pH of the PG solution is acidic. The pH values of 0.5–3 w/v % PG dispersions changed in the range of 5.60–4.56, but in the case of GA, 2.5–15 w/v % dispersions were present at pH = 5.45–4.38. Based on and -A–C, PG and GA had their best emulsifying performance at 1.5% and 15% w/v, respectively, and were selected for next steps.
Effect of pH
For showing the effect of pH on the droplet size of emulsions, the pH of gum solutions was adjusted before homogenization and was considered as the pH of the emulsion, because adding oil did not change the pH. Based on , the droplet size of GA emulsions at pH = 2–7 was significantly constant (p > 0.05), which is in agreement with the current research studies on the pH stability of biopolymer-based oil-in-water emulsions.[Citation38,Citation44] Nevertheless, there was a significant effect (p < 0.05) on the droplet size at pH = 2, which is due to the lesser negative charge on the oil droplets stabilized with GA. With a decrease in pH to pKa GA, some carboxyl groups lost their negative charge, which is the reason why the ES decreased. Moreover, a decrease in the emulsifying capacity is due to the increase in the needed amount of biopolymer on the oil droplet surface that has been reported previously (the amount of GA needed for oil droplet coverage went higher upon reducing the pH to lower than neutral pH).[Citation45]
Figure 6. Effect of pH on the mean diameter (D32), interfacial tension and zeta potential of PG- and GA-stabilized emulsions.
– For each gum, similar letters of each parameter are not significantly different at p < 0.05.
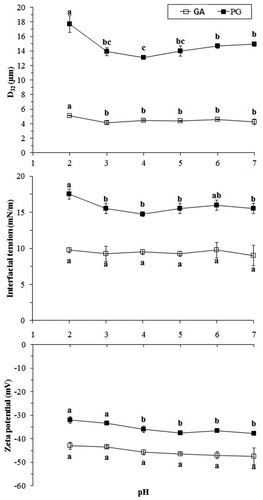
It is clear in that the influence of pH on the droplet size of PG emulsions is quite different. The D32 value was dependent on pH, and this value was significantly increased at pH = 2 (p < 0.05). The mean droplet size of emulsions showed that PG was able to create stable emulsions at pH = 3–7. So, it is possible to use PG as an emulsifier in food systems at acidic pH. At pH = 2, the mean droplet size increased, which was possibly related to the reduction of the electrostatic repulsion between oil droplets. In fact, a possible explanation for the pH stability of biopolymer emulsions is that oil droplets are coated with thick hydrophilic biopolymer layers, and that they are stable due to conformational repulsions rather than electrostatic ones. A thick layer of biopolymers could decrease the van der Waals attractive force between oil droplets. In addition, it is steric hindrance rather than electrostatic repulsion that is the main stabilizing mechanism independent of pH for gum.[Citation14] Abdolmaleki et al.[Citation35] similarly reported the instability for sunflower oil-in-water emulsions prepared with tragacanth at pH = 4. Hosseini-Parvar et al.[Citation3] showed that the Basil seed gum-stabilized emulsions were more sensitive to low pHs (≤6.0), than to the alkaline conditions (pH>7.0).
The dependence of the interfacial tension on pH is shown in . The results showed that the interfacial tension of the PG emulsion significantly increased with a decrease in pH to 2, but interfacial tension changes for GA emulsions were not significant (p˂0.05). Therefore, the pH of the emulsion has a slight effect on the interfacial properties of PG and AG. Abdolmaleki et al.[Citation35] reported that the interfacial tension of an emulsion prepared with tragacanth gum was increased at acidic pH.[Citation35]
The effect of pH on the zeta potential of oil-in-water emulsions stabilized by PG and GA is presented in . The zeta potential values of the PG and GA emulsions decreased in the range of −37.75 to −32.00 mV and −47.50 to −43 mV, with pH decreasing from 7 to 2, respectively. This change was only significant for PG emulsions at 0.05. As the decrease in pH will lead to a decrease in electrostatic repulsion, it results in the reduction of zeta potential. In addition, this reduction may be related to the shielding of negative charges of PG and GA by positive charges of medium to acidic pH. It should be noted that the zeta potential of PG and GA remained negative in all ranges of pH values, possibly because of the negatively charged (COO–) groups on the acidic polysaccharide. The results above are in agreement with the previous observation by Abdolmaleki et al.[2016, Citation35] Interestingly, the GA had a much higher negative charge than the PG at all pH values, which may be related to the higher charge density of the GA molecules compared to PG. This phenomenon is very important for the interactions of biopolymer-coated oil droplets with other charged groups, such as metal ions that promote lipid oxidation.[Citation38]
Effect of NaCl
The determination of the salt concentration effect on the emulsifying properties of hydrocolloids is necessary. The stability of the hydrocolloid to salinity suggested that it is a good candidate for use in some industries that involve salt. This feature would be of great interest in food production that produces a large amount of salted food. According to , a slight variation occurred in the droplet size of GA and PG emulsions with an increase in NaCl. Changes in the mean droplet size of GA emulsions were slightly increased at 0–50 mM NaCl concentrations, and were thereafter constant (no significant differences at p > 0.05) until 150 mM. Afterward, the GA emulsion with 200 and 300 mM NaCl had the highest D32 significantly, but the NaCl concentration had no significant differences (p < 0.05) on the droplet size of the PG emulsions only at 300 mM. At high salt concentration, an increase in droplet size may be related to the charge density of gum and salting out effects. Qian et al.[Citation38] found a small change in the droplet size of emulsions stabilized by GA, along with increasing ionic strength for NaCl (0–500 mM). NaCl sensitivity has been reported in Basil seed gum-stabilized emulsions by Hosseini-Parvar et al.[Citation3], and sugar beet pectin (SBP)-stabilized emulsions by Nakauma et al.[Citation45]
Figure 7. Effect of NaCl on the mean diameter (D32), interfacial tension, and zeta potential of PG- and GA-stabilized emulsions (pH = 4.50 ± 0.05).
– For each gum, similar letters of each parameter are not significantly different at p < 0.05.
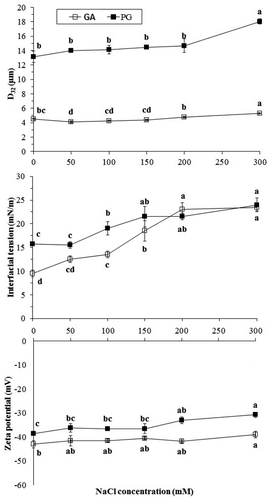
The dependence of interfacial tension of PG- and GA-stabilized emulsions on NaCl concentration is shown in . The results showed that the interfacial tension increased significantly (p < 0.05) with an increase in the NaCl concentration, and that PG emulsions had higher values at all concentrations. Interestingly, the interfacial tension of PG emulsions at 200 mM NaCl was lower than that of GA emulsions. Therefore, salting of emulsions has a strong effect on the interfacial properties of PG and GA. Abdolmaleki et al.[Citation35] reported that the interfacial tension of emulsions prepared with tragacanth increased at high salt concentration.
The effect of NaCl concentration on the zeta potential of emulsions stabilized by PG and GA is presented in . The zeta potential values decreased with an increase in NaCl concentration. The zeta potential values of PG and GA emulsions decreased significantly (p˂0.05) in the ranges of −38.50 to −30.75 mV and −43.00 to −39.00 mV, along with the 0–300 mM increase in NaCl, respectively. These results are in agreement with previous reports by Abdolmaleki et al.[Citation35] for emulsions stabilized by gum tragacanth. There was little change in the zeta potential of the PG- and GA-stabilized emulsions with an increase in NaCl concentration (0–200 mM), which may be related to charge compensation effects such as a change in the interfacial structure or composition with an increase in ionic strength.
Effect of CaCl2
Generally, the addition of CaCl2 usually decreases the electrostatic repulsions between oil droplets and causes instability of emulsions. Therefore, emulsions are unstable at high concentrations of CaCl2. As shown in , CaCl2 had no significant effect (p > 0.05) on the mean droplet size of GA emulsions. These results are similar to previous studies.[Citation44,Citation45] Whereas the D32 values of PG decreased significantly (p < 0.05) as the CaCl2 concentration increased (0–300 mM) from 13.11 ± 0.79 to 5.70 ± 0.04 µm, which is a very striking trend. One of the reasons for the improvement of the emulsion properties of PG at a high CaCl2 concentration could be related to the increase in the chain flexibility of biopolymers and their smoother movement within the interfacial area. When CaCl2 was increased to 200–300 mM, monomodal distribution was obtained and the mean droplet size decreased. Although the droplet size of PG emulsions was decreased with an increase in CaCl2 concentration, it cannot be expected to have long-term stability during storage considering its minimum droplet size.
The dependence of the interfacial tension of PG- and GA-stabilized emulsions on CaCl2 concentration is shown in . The interfacial tension of GA emulsions increased significantly (p < 0.05) with an increase in the CaCl2 concentration to 100 mM and then decreased (100–200 mM), so finally it is increased at 300 mM CaCl2 concentration again. The interfacial tension of PG was significant at 0–300 mM CaCl2 concentration. PG emulsions had lower interfacial tension values in 0–100 mM concentration than the AG emulsion. Interestingly, the interfacial tension of PG emulsions at 150–300 mM CaCl2 was higher than the AG emulsion. Therefore, the salt of systems has a strong effect on the interfacial properties of PG and AG.
Figure 8. Effect of CaCl2 on the mean diameter (D32), interfacial tension, and zeta potential of PG- and GA-stabilized emulsions (pH = 4.50 ± 0.05).
– For each gum, similar letters of each parameter are not significantly different at p < 0.05.
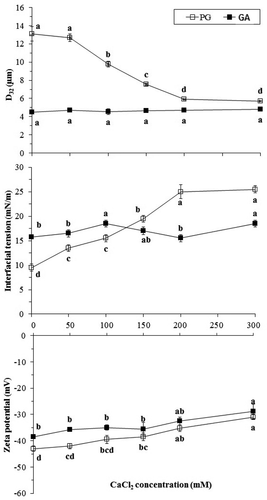
Our results also showed that CaCl2 had a significant effect on the zeta potential of emulsions stabilized with PG and GA. When the CaCl2 concentration was increased from 0 to 300 mM, there was a dramatic decrease in the negative value of the zeta potential on PG- and GA-stabilized droplets (), which could have occurred as a result of two different phenomena: electrostatic screening and ion binding. The zeta potential on the emulsion droplets would contribute to electrostatic repulsion, but the magnitude would not be large enough on its own to stabilize emulsions. These results suggest that steric repulsion is a more significant stabilizing force in PG-stabilized emulsions than electrostatic repulsion. The above results revealed that the effect of the two salts (NaCl and CaCl2) on the PG emulsion is different together and also showed different behaviors on D32 of PG emulsions.
Effect of temperature
Most of the food emulsions are exposed to heat treatment during production processing (e.g., sterilization, pasteurization, or cooking). In this study, the temperature had no significant effect (p > 0.05) on the GA emulsions, which is due to the formation of a protective film around oil droplets (). In the case of the PG emulsion, the temperature caused no significant changes (p < 0.05) to the droplet size of the emulsions only at 90°C. After heat treatments ranging from 25°C to 90°C, the mean droplet sizes of PG and GA emulsions changed from 11.04 ± 0.01 to 14.54 ± 0.09 and from 4.21 ± 0.04 to 4.21 ± 0.11 μm, respectively. This can be related to the fact that hydrocolloid-stabilized emulsions are not unfolded to expose non-polar groups at higher temperatures.
Figure 9. Effect of heat treatment on the mean diameter (D32) of PG- and GA-stabilized emulsions (gray: GA, white: PG).
– For each gum, similar letters are not significantly different at p < 0.05.
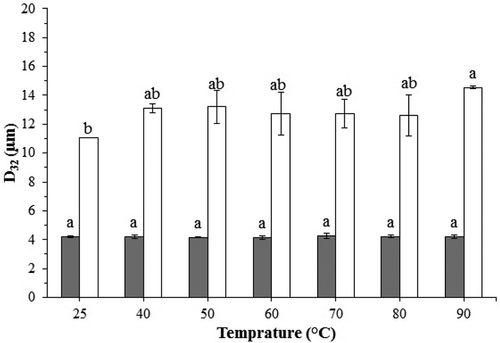
Therefore, mechanisms of instability (flocculation, coalescence, and Ostwald ripening) had a low impact on the size of oil droplets of GA- and PG-stabilized emulsions. Furthermore, the emulsions stabilized with hydrocolloids were not affected by denaturation at high temperatures; in fact, heating favors hydrophobic interactions, which can lead to droplet aggregation.[Citation1] These results are similar to previous studies.[Citation44] Also, Qian et al.[Citation38] reported that orange oil-in-water emulsions were relatively stable to droplet aggregation and creaming after heat treatment (30–90°C). Hosseini-Parvar et al. (2016) observed the largest droplet size in the heated Basil seed gum emulsions, which may be related to the droplet coalescence and irreversible flocculation induced by heating.[Citation3]
Microstructure of emulsions
shows microscopic observations of PG- and GA-stabilized emulsions. The microstructure of the droplet emulsions was produced at different concentrations, pHs, and salt contents, which indicated that images are in agreement with droplet size analysis. Based on the microscopic observations, PG was able to promote relatively larger droplet sizes at higher concentration compared to GA. As can be shown in -1–3, the droplet size of the GA emulsion decreased with an increase in gum concentration. But, for PG emulsions, oil droplets increased at higher PG concentration. In the case of pH, NaCl and CaCl2, microscopic observations demonstrated that - to 9 had higher droplet size compared to - with similar 15% GA concentration, although some large droplets remained. In comparison of -10 to 12 with - (with similar 1.5% PG concentration), microscopic observations demonstrated some large oil droplets in the PG emulsion in the presence of low pH and higher 300 mM NaCl concentration. –12 show that the droplet size of the emulsion decreased in the presence of 300 mM CaCl2 salt that is in accordance with the droplet size distribution of PG emulsions.
Conclusion
The present study compared the emulsifying properties of PG and GA hydrocolloids by evaluating the emulsion stabilizing properties in terms of droplet size distribution, zeta potential, interfacial tension, viscosity, microscopic observation, and centrifugation technique. The emulsifying properties were studied as a function of concentration, pH, ionic strength (NaCl and CaCl2), and heat treatment. The stabilized emulsions with a small mean droplet size required 1.5% w/v PG concentration that is 10-fold lower than GA concentration. However, the final mean diameters of 15% w/v GA and 1.5% w/v PG-stabilized emulsions were 4.52 ± 0.03 and 9.89 ± 0.68 μm, respectively. Droplet size distributions of emulsions stabilized with PG remained approximately constant over the temperature range, the pH values, and the NaCl and CaCl2 concentrations studied, confirmed by droplet size analysis, zeta potential, and interfacial tension properties. Moreover, heat treatment had no significant effect on the droplet size distribution of the PG emulsion. In terms of the apparent viscosity, PG solutions had a much higher viscosity than GA. According to the above results, PG can be introduced as a food emulsifier with potential application in emulsion systems with acidic pH, high salt content, and thermal processing. Also, it can be suggested as an emulsifying and/or stabilizing agent, both alone and combined with GA in emulsion systems with low viscosity. The mixture of PG and GA with an optimum ratio can be a good emulsifying agent that further researches to illustrate that its functionalities are needed.
Acknowledgments
This research is a part of the “Industrialization of PG in beverage emulsions formulation” project.
Additional information
Funding
References
- McClements, D. J.;. Food Emulsions: Principles, Practices, and Techniques; CRC Press, USA: Florida, Boca Paton, 2004.
- Dickinson, E.;. Hydrocolloids as Emulsifiers and Emulsion Stabilizers. Food Hydrocolloids 2009, 23, 1473–1482. DOI: 10.1016/j.foodhyd.2008.08.005.
- Hosseini-Parvar, S. H.; Osano, J. P.; Matia-Merino, L. Emulsifying Properties of Basil Seed Gum: Effect of pH and Ionic Strength. Food Hydrocolloids 2016, 52, 838–847. DOI: 10.1016/j.foodhyd.2015.09.002.
- Dickinson, E.;. Hydrocolloids at Interfaces and the Influence on the Properties of Dispersed Systems. Food Hydrocolloids 2003, 17, 25–39. DOI: 10.1016/S0268-005X(01)00120-5.
- Verbeken, D.; Dierckx, S.; Dewettinck, K. Exudate Gums: Occurrence, Production, and Applications. Applied Microbiology and Biotechnology 2003, 63, 10–21. DOI: 10.1007/s00253-003-1354-z.
- Garti, N.; Leser, M. E. Emulsification Properties of Hydrocolloids. Polymers for Advanced Technologies 2001, 12, 123–135. DOI: 10.1002/1099-1581(200101/02)12:1/2<>1.0.CO;2-L.
- Fadavi, G.; Mohammadifar, M.; Zargaran, A.; Azadnia, E. The Study of Composition, Molecular Weight and Rheological Characteristics of Zedo Gum Exudates from Amygdalus Scoparia. Iranian Journal of Nutrition Sciences & Food Technology 2014, 7, 35–40.
- Golkar, A.; Nasirpour, A.; Keramat, J. β-lactoglobulin-Angum Gum (Amygdalus Scoparia Spach) Complexes: Preparation and Emulsion Stabilization. Journal of Dispersion Science and Technology 2015, 36, 685–694. DOI: 10.1080/01932691.2014.919587.
- Golkar, A.; Nasirpour, A.; Keramat, J. Emulsifying Properties of Angum Gum (Amygdalus Scoparia Spach) Conjugated to β-lactoglobulin through Maillard-Type Reaction. International Journal of Food Properties 2015, 18, 2042–2055. DOI: 10.1080/10942912.2014.962040.
- Abbasi, S.; Mohammadi, S. Stabilization of Milk–Orange Juice Mixture Using Persian Gum: Efficiency and Mechanism. Food Bioscience 2013, 2, 53–60. DOI: 10.1016/j.fbio.2013.04.002.
- Fadavi, G.; Mohammadifar, M. A.; Zargarran, A.; Mortazavian, A. M.; Komeili, R. Composition and Physicochemical Properties of Zedo Gum Exudates from Amygdalus Scoparia. Carbohydrate Polymers 2014, 101, 1074–1080. DOI: 10.1016/j.carbpol.2013.09.095.
- Gasempour, Z.; Alizadeh, M.; Bari, M. R. Optimisation of Probiotic Yoghurt Production Containing Zedo Gum. The International Journal of Dairy Technology 2012, 65, 118–125. DOI: 10.1111/j.1471-0307.2011.00740.x.
- Jafari, S. M.; Beheshti, P.; Assadpoor, E. Rheological Behavior and Stability of D-Limonene Emulsions Made by a Novel Hydrocolloid (Angum Gum) Compared with Arabic Gum. Journal of Food Engineering 2012, 109, 1–8. DOI: 10.1016/j.jfoodeng.2011.10.016.
- Jafari, S. M.; Beheshti, P.; Assadpour, E. Emulsification Properties of a Novel Hydrocolloid (Angum Gum) for D-Limonene Droplets Compared with Arabic Gum. International Journal of Biological Macromolecules 2013, 61, 182–188. DOI: 10.1016/j.ijbiomac.2013.06.028.
- Golkar, A.; Nasirpour, A.; Keramat, J. Production of Reduced-Fat Mayonnaise Using Electrostatic and Covalent Complexes of β-lactoglobulin and Farsi Gum. Iranian Journal of Nutrition Sciences & Food Technology 2015, 10, 103–114.
- Hashtjin, A. M.; Abbasi, S. Nano-Emulsification of Orange Peel Essential Oil Using Sonication and Native Gums. Food Hydrocolloids 2015, 44, 40–48. DOI: 10.1016/j.foodhyd.2014.08.017.
- Azarikia, F.; Abbasi, S. Mechanism of Soluble Complex Formation of Milk Proteins with Native Gums (Tragacanth and Persian Gum). Food Hydrocolloids 2015, 59, 35–44. DOI: 10.1016/j.foodhyd.2015.10.018.
- Hadian, M.; Hosseini, S. M. H.; Farahnaky, A.; Mesbahi, G. R.; Yousefi, G. H.; Saboury, A. A. Isothermal Titration Calorimetric and Spectroscopic Studies of β-lactoglobulin–Water-soluble Fraction of Persian Gum Interaction in Aqueous Solution. Food Hydrocolloids 2015, 55, 108–118. DOI: 10.1016/j.foodhyd.2015.11.006.
- Khalesi, H.; Emadzadeh, B.; Kadkhodaee, R.; Fang, Y. Effects of Biopolymer Ratio and Heat Treatment on the Complex Formation between Whey Protein Isolate and Soluble Fraction of Persian Gum. Journal of Dispersion Science and Technology 2016, 38, 1234–1241. DOI: 10.1080/01932691.2016.1230064.
- Khalesi, H.; Emadzadeh, B.; Kadkhodaee, R.; Fang, Y. Whey Protein isolate-Persian Gum Interaction at Neutral pH. Food Hydrocolloids 2015, 59, 45–49. DOI: 10.1016/j.foodhyd.2015.10.017.
- Raoufi, N.; Fang, Y.; Kadkhodaee, R.; Phillips, G. O.; Najafi, M. N. Changes in Turbidity, Zeta Potential and Precipitation Yield Induced by Persian Gum‐Whey Protein Isolate Interactions during Acidification. Journal of Food Processing and Preservation 2016, 41, 1–8.
- AOAC. Official Methods of Analysis, 17; Washington, DC: Association of Official Analytical Chemists, USA, 2003.
- Mishra, S.; Mann, B.; Joshi, V. Functional Improvement of Whey Protein Concentrate on Interaction with Pectin. Food Hydrocolloids 2001, 15, 9–15. DOI: 10.1016/S0268-005X(00)00043-6.
- Lee, P.-E.; Choo, W.-S. Characterization of Flaxseed Oil Emulsions. The Journal of Food Science and Technology 2014, 52, 1–9.
- Yebeyen, D.; Lemenih, M.; Feleke, S. Characteristics and Quality of Gum Arabic from Naturally Grown Acacia Senegal (Linne) Willd. Trees in the Central Rift Valley of Ethiopia. Food Hydrocolloids 2009, 23, 175–180. DOI: 10.1016/j.foodhyd.2007.12.008.
- Fathi, M.; Mohebbi, M.; Koocheki, A. Introducing Prunus Cerasus Gum Exudates: Chemical Structure, Molecular Weight, and Rheological Properties. Food Hydrocolloids 2016, 61, 946–955. DOI: 10.1016/j.foodhyd.2016.07.004.
- Fathi, M.; Mohebbi, M.; Koocheki, A. Some Physico-Chemical Properties of Prunus Armeniaca L. Gum Exudates. International Journal of Biological Macromolecules 2016, 82, 744–750. DOI: 10.1016/j.ijbiomac.2015.09.068.
- Malsawmtluangi, C.; Thanzami, K.; Lalhlenmawia, H.; Selven, V.; Palanisamy, S.; Kandasamy, R.; Pachuau, L. Physicochemical Characteristics and Antioxidant Activity of Prunus Cerasoides D. Don Gum Exudates. International Journal of Biological Macromolecules 2014, 69, 192–199. DOI: 10.1016/j.ijbiomac.2014.05.050.
- Bouaziz, F.; Koubaa, M.; Helbert, C. B.; Kallel, F.; Driss, D.; Kacem, I.; Ghorbel, R.; Chaabouni, S. E. Purification, Structural Data and Biological Properties of Polysaccharide from Prunus Amygdalus Gum. International Journal of Food Science and Technology 2015, 50, 578–584. DOI: 10.1111/ijfs.2015.50.issue-3.
- Mahfoudhi, N.; Chouaibi, M.; Donsı`, F.; Ferrari, G.; Hamdi, S. Chemical Composition and Functional Properties of Gum Exudates from the Trunk of the Almond Tree (Prunus Dulcis). Food Science and Technology International 2012, 18, 241–250. DOI: 10.1177/1082013211415173.
- Milani, J.; Ghanbarzadeh, B.; Maleki, G. Rheological Properties of Anghouzeh Gum. International Journal of Food Engineering 2012, 8, DOI: 10.1515/1556-3758.2071.
- Mcclements, D. J.;. Critical Review of Techniques and Methodologies for Characterization of Emulsion Stability. Critical Reviews in Food Science and Nutrition 2007, 47, 611–649. DOI: 10.1080/10408390701289292.
- Huang, X.; Kakuda, Y.; Cui, W. Hydrocolloids in Emulsions: Particle Size Distribution and Interfacial Activity. Food Hydrocolloids 2001, 15, 533–542. DOI: 10.1016/S0268-005X(01)00091-1.
- Wu, Y.; Cui, W.; Eskin, N.; Goff, H. An Investigation of Four Commercial Galactomannans on Their Emulsion and Rheological Properties. Food Research International 2009, 42, 1141–1146. DOI: 10.1016/j.foodres.2009.05.015.
- Abdolmaleki, K.; Mohammadifar, M. A.; Mohammadi, R.; Fadavi, G.; Meybodi, N. M. The Effect of pH and Salt on the Stability and Physicochemical Properties of Oil-In-Water Emulsions Prepared with Gum Tragacanth. Carbohydrate Polymers 2016, 140, 342–348. DOI: 10.1016/j.carbpol.2015.12.081.
- Castel, V.; Rubiolo, A. C.; Carrara, C. R. Droplet Size Distribution, Rheological Behavior and Stability of Corn Oil Emulsions Stabilized by a Novel Hydrocolloid (Brea Gum) Compared with Gum Arabic. Food Hydrocolloids 2017, 63, 170–177. DOI: 10.1016/j.foodhyd.2016.08.039.
- McNamee, B. F.; O’Riorda, E. D.; O’Sullivan, M. Emulsification and Microencapsulation Properties of Gum Arabic. The Journal of Agricultural and Food Chemistry 1998, 46, 4551–4555. DOI: 10.1021/jf9803740.
- Qian, C.; Decker, E. A.; Xiao, H.; McClements, D. J. Comparison of Biopolymer Emulsifier Performance in Formation and Stabilization of Orange Oil-In-Water Emulsions. Journal of the American Oil Chemists’ Society 2011, 88, 47–55. DOI: 10.1007/s11746-010-1658-y.
- Shao, P.; Shao, J.; Jiang, Y.; Sun, P. Influences of Ulva Fasciata Polysaccharide on the Rheology and Stabilization of Cinnamaldehyde Emulsions. Carbohydrate Polymers 2016, 135, 27–34. DOI: 10.1016/j.carbpol.2015.08.075.
- Al-Assaf, S.; Sakata, M.; McKenna, C.; Aoki, H.; Phillips, G. O. Molecular Associations in Acacia Gums. Structural Chemistry 2009, 20, 325–336. DOI: 10.1007/s11224-009-9430-3.
- Osano, J. P.; Hosseini-Parvar, S. H.; Matia-Merino, L.; Golding, M. Emulsifying Properties of a Novel Polysaccharide Extracted from Basil Seed (Ocimum Bacilicum L.): Effect of Polysaccharide and Protein Content. Food Hydrocolloids 2014, 37, 40–48. DOI: 10.1016/j.foodhyd.2013.09.008.
- Vasile, F. E.; Martinez, M. J.; Ruiz-Henestrosa, V. M. P.; Judis, M. A.; Mazzobre, M. F. Physicochemical, Interfacial and Emulsifying Properties of a Non-Conventional Exudate Gum (Prosopis Alba) in Comparison with Gum Arabic. Food Hydrocolloids 2016, 56, 245–253. DOI: 10.1016/j.foodhyd.2015.12.016.
- Guzey, D.; McClements, D. J. Impact of Electrostatic Interactions on Formation and Stability of Emulsions Containing Oil Droplets Coated by β-lactoglobulin− Pectin Complexes. The Journal of Agricultural and Food Chemistry 2007, 55, 475–485. DOI: 10.1021/jf062342f.
- Chanamai, R.; McClements, D. Comparison of Gum Arabic, Modified Starch, and Whey Protein Isolate as Emulsifiers: Influence of pH, CaCl2 and Temperature. The Journal of Food Science 2006, 67, 120–125. DOI: 10.1111/j.1365-2621.2002.tb11370.x.
- Nakauma, M.; Funami, T.; Noda, S.; Ishihara, S.; Al-Assaf, S.; Nishinari, K.; Phillips, G. O. Comparison of Sugar Beet Pectin, Soybean Soluble Polysaccharide, and Gum Arabic as Food Emulsifiers. 1. Effect of Concentration, pH, and Salts on the Emulsifying Properties. Food Hydrocolloids 2008, 22, 1254–1267. DOI: 10.1016/j.foodhyd.2007.09.004.