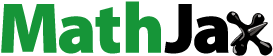
ABSTRACT
The effects and safety of electron beam irradiation (EBI) treatment on the detoxification of aflatoxin B1 (AFB1) in the peanut meal were evaluated in this article. The AFB1 degradation was predominantly affected by both initial AFB1 and water concentrations. The degradation of AFB1 in the selected concentrations (0.5–5 ppm) was proven to follow pseudo first-order reaction kinetics (R2 > 0.95). The AFB1 degradation was faster when the initial concentration was 5 ppm and the moisture content was 21.47%, in comparison with the initial concentration of 1 ppm and 0.5 ppm and the moisture content of 14.32% and 8.74%, respectively. The Ames and cytotoxicity tests were employed to evaluate the residual toxicity of EBI-treated peanut meal. The mutagenic activity of EB-treated samples was completely lost compared with that of untreated samples and the degradation products in peanut meal has almost no cell toxicity.
Introduction
Aflatoxins (AFs), which are secondary metabolites of Aspergillus flavus and Aspergillus parasiticus, are a group of highly toxic, mutagenic, and carcinogenic compounds.[Citation1] Aflatoxin B1 (AFB1) is the most potent teratogen, mutagen, and hepatocarcinogen among the various AFs, and is classified as a Group 1 carcinogen by the International Agency for Research in Cancer.[Citation2,Citation3] AFs may be a problem in many countries, but are especially prevalent in tropical and subtropical regions, where the temperature and humidity are optimum for the growth of the molds and the production of the toxin. Many important crops, specifically peanuts, are susceptible to such contaminations.[Citation4] Peanut meal, which is a by-product of peanut oil with 43.7% protein, is only used for animal feed, due to the contamination of AFs. The most rational and economic approach to avoiding hazards associated with AFs is to prevent food contamination by toxigenic fungi. However, this is not always possible under certain agronomic storage practices. One major concern is the salvation of food and feed stuff that were already contaminated by toxic fungal metabolites via removal or degradation procedures.[Citation1]
Physical, chemical, and biological approaches are available for the detoxification of AFs.[Citation5–Citation8] Compared with the chemical and biological methods, the physical decontamination of AFB1 seems more promising, such as ozonation,[Citation6] low-temperature radio frequency plasma,[Citation8] UV irradiation[Citation9] etc., which have gained practical applications and have been reported capable of effectively degrading AFB1 in artificial systems or real foods. Electron beam irradiation (EBI) technology, an advanced and novel processing method using nuclear technology, can be applied to destroy organic molecules through direct or indirect oxidation.[Citation10–Citation13] The advantages of EBI processing include short processing time, in-line processing, high effectiveness, few variables, low heat, low equipment costs, and dosage control.[Citation14] EBI has been proven as an effective method in AFs degradation in coconut agar.[Citation15] However, it is uncertain whether EBI could be used in peanut meal detoxification, as the exact nature of the radiation products and the toxicity of the degradation products remain unknown.
In our previous research, the effectiveness, nature, and safety of different AFB1 degradation products were determined in various media.[Citation16–Citation18] In this study, the efficiency, degradation kinetics, and mutagenesis of degradation of AFB1 in peanut meal were evaluated.
Materials and methods
Chemicals and reagents
AFB1 (2,3,6α,9α-tetrahydro-4-methoxycyclopenta[c]furo[2,3:4,5]furo[2,3-h]chromene-1,11-dione; purity > 98%) was purchased from Fermentek (Jerusalem, Israel). The peanut meal (traditional type, no AFB1 detected) was supplied by Shandong Luhua Group Co., Ltd. Deionized water (resistivity, 18 MΩ/cm) was obtained from Milli-Q SP Reagent Water system (Millipore, Bedford, MA, USA) and pre-filtered through a 0.2-μm membrane. High-performance-liquid-chromatography-grade acetonitrile and dimethyl sulfoxide (DMSO) were purchased from Sigma (St. Louis, MO, USA).
Preparation of peanut meal with AFB1
Standard stock solutions of 200 µg/ml AFB1 were stored, and then 1, 2, and 10 µg/ml of AFB1 acetonitrile solution were prepared according to Liu et al.[Citation9] In the degradation experiments, 5 ml of AFB1 acetonitrile solution was mixed thoroughly with 10 g of peanut meal (no AFB1 detected). The mixture was then vaporized in drying cabinet at 37°C to remove the acetonitrile and to finally obtain dry peanut meal.
Different moisture in peanut meal
The water was uniformly sprayed on the dry peanut meal containing AFB1, and then dried in the darkness to reach moisture contents of 21.47%, 14.32%, and 8.74%. The air oven method was used to measure the moisture content of peanut meal samples. Each sample (2 g) was dried in an oven (Binder, FP115, Germany) at 105°C for 6 h.
Degradation procedure using EBI
Peanut meal samples containing various concentrations of AFB1 were irradiated at 4°C using an industrial electron accelerator, 5 MeV (Wuxi EL PONT Radiation Technology Co., Ltd, Wuxi, China) with a current of 20 mA, a scan width of 1000 mm, and a dose rate of approximately 2 kGy/s−1. The irradiation equipment and operation conditions are same as described in the work done by Wang et al. [Citation8] Temperature and pressure were controlled during the irradiation. Negative experiments without EBI were performed in parallel under the same conditions.
Analysis of degradation products
After EBI treatment, the peanut meal samples were steeped in three volumes of acetonitrile and the degradation products were dissolved in acetonitrile. AFB1 was two times extracted from samples with equal volume of acetonitrile, and the average recoveries of this method are 92.07–103.25% (n = 6). The acetonitrile extracts were analyzed by ultra performance liquid chromatography (UPLC) tandem with time-of-fight mass spectrometry with MS data-acquistion mode (UPLC-Q-TOF MS). UPLC was performed on a Waters (Milford, MA, USA) Acquity. According to Liu et al.,[Citation16] they briefly introduce experimental conditions. The acetonitrile containing AFB1 degradation products was simultaneously dried under a jet of nitrogen and dissolved in DMSO for a safety evaluation.
Ames test for mutagenesis
The S9 Enzyme Activation Kit was obtained from EPBI (Ontario, Canada) and the total concentration of protein was 51.4 mg of protein per ml. In the mix, the final concentration of S9 was 300 µg/ml. The Ames test was operated using previous study by Liu et al.[Citation9] Samples, including untreated and EBI-treated trials, were re-dissolved in DMSO to give quantities of approximately 5, 10, 20, and 40 ng AFB1 per tube.
Cytotoxicity test
HepG2 cells were cultured as described by Liu et al.[Citation9] and treated by original and degraded AFB1 at 100 μM for 24 h. In brief, AFB1 and its degradation products were dissolved in DMSO and added to the culture media. The final concentration of DMSO in the media was less than 0.1%. All compounds were visibly soluble in the media and were detected by UPLC to confirm the concentration. Cell viability was determined using the MTT [3-(4,5-dimethylthiazol-2-yl)-2,5-diphenyltetrazolium bromide] assay [Citation9] (Gibco Life Technologies).
Experimental design and statistical analysis
The samples were tested three times for each independent experiment. The data were assessed using analysis of variance, and a means of comparison was performed with the Dunnet test using the Statistical Analysis System.[Citation19]
Results and discussion
Degradation of AFB1 at various initial concentrations
The effect of initial concentration on the degradation of AFB1 by EBI treatment is presented in . The initial concentrations of AFB1 were 0.5 ppm, 1.0 ppm, and 5.0 ppm, and there was no detectable difference shown in the blank experiment with different concentrations (results exemplified by that pertaining to an initial concentration of 0.5 ppm shown in ). Therefore, the declines of AFB1 quality can be observed in the AFB1 degradation curve during the degradation process. All degradation kinetics of AFB1 by EBI treatment can be described as a dynamic first-order kinetics model. Three degradation curves showed that the kinetics of the degradation of the degradation of AFB1 in EBI were better described with dynamic first-order kinetics model. Although the degradation behavior of AFB1 in the current study was similar as the AFB1 treated by the low-temperature plasma, the efficiency of two methods was not comparable, because of different operation conditions.[Citation8]
Degradation of AFB1 at different concentration of water in peanut meal
The degradation of AFB1 induced by water content in the peanut meal is shown in . The initial concentration of AFB1 was 1 ppm, and water concentrations in the peanut meal were 8.74%, 14.32%, and 21.47%. With the increase of water concentration, the degradation rate was raised (). Thus, the degradation rate of AFB1 negatively correlated with the water content in peanut meal.
Degradation kinetics of AFB1
The plot of ln (Ct/C0) versus irradiation dose is given in , for AFB1 at different initial concentrations and different water concentrations, and the deduced parameters are listed in . The linear relationship between ln (Ct/C0) and irradiation dose indicates that the degradation followed a pseudo first-order kinetics well (R2 ≥ 0.95), given by the equation:
Table 1. Parametric equations of AFB1 degradation at different initial concentrations in the peanut meal.
where C0 and Ct are the concentrations at times 0 and t, t is the irradiation time and A & B are the pseudo first-order rate constants. It was found that the values of A and B dramatically changed when different initial AFB1 and water concentrations in the peanut meal were used (). The degradation rate of AFB1 was decreased in the order of 5 ppm > 1 ppm > 0.5 ppm of initial AFB1 concentrations and 21.47% > 14.32% > 8.74% of water contents in the peanut meal. Therefore, the degradation of AFB1 strongly depends on the initial AFB1 and water concentrations in the peanut meal.
Degradation products of AFB1 in peanut meal
Since AFB1 could be degraded to water-soluble or more polar products,[Citation20] acetonitrile was used to extract the degraded products, which were analyzed by UPLC-Q-TOF-MS. However, did not reveal the formation of any degraded products, suggesting that AFB1 was most likely broken down to products with chemical properties different from that of AFB1. This result is different from the degradation of AFB1 in water and acetonitrile solution under UV irradiation, as detected in our previous articles.[Citation21,Citation22] A postulated mechanism for this reaction could involve a cleavage of the double bond on AFB1, as a consequence of the EBI treatment (). After the formation of primary ozonide, this product may rearrange to a molozonide derivative to yield a variety of carbonyl compounds (e.g., aldehydes and ketones). The proposed degradation pathway indicates that R–COOH, R–CHO, R–CO–R′, and CO2 were not detected in this study. The minor compounds in might be the final degradation products of AFB1 from UV irradiation treatment on the peanut surface, which is in agreement with the previous report.[Citation20]
Ames test for mutagenicity
The dose–response of AFB1 mutagenicity in tester strains TA98 and TA100 (named untreated extract) is shown in the oblique line part on . The spontaneous mutation rates (0 ng per tube) were 20–70 and 70–150 revertant per plate for TA98 and TA100, respectively, which are in agreement with those of Maron and Ames.[Citation23]
Figure 6. Revertant of Salmonella trphimurium tester strains TA98(A) and TA100(B) of AFB1 and the degradation in the peanut meal. *p < 0.05, **p < 0.001 versus AFB1.
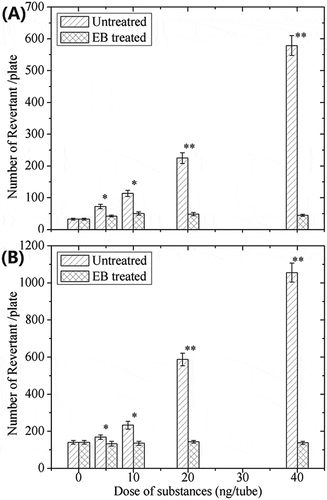
The mutagenic response of Salmonella typhimurium tester strains TA98 and TA100 are presented in . AFB1 concentrations at 5, 10, 20, and 40 ng per tube were used as positive control mutagens for mutation induction (medium lineaobliqua), which agreed with previous results.[Citation24] Numbers of revertantin EBI-treated samples with initial quantities of 5, 10, 20, 40 ng per tube (dense graticule) were reduced to ~46 for TA98 and ~115 for TA100, respectively, which is in agreement with the study of Méndez-Albores.[Citation20] The numbers of revertant are significantly lower than the numbers of the corresponding untreated samples, and are within the ranges of the spontaneous revertant count (negative control). The proposed reason for this is that the minor compounds have lost the toxic structure to induce the toxicity at the same time (shown in ).
Cytotoxicity test
Cell viability in the presence of AFB1 and its degradation products were assessed by the MTT assay. After the incubation for 24 h, the viability of HepG2 cells decreased in a concentration-dependent manner with the increase dose of AFB1 (0–100 μM) (). The viability of HepG2 cells was reduced nearly 50% after treatment with 100 μM AFB1, compared to that of untreated cells (0 μM) (p < 0.001). However, both the untreated cells and cells with degraded products performed similar viabilities. There is a significant difference on the mortality rates of HepG2 cells that presented in the AFB1 degraded products in the peanut meal (p < 0.01).
Conclusion
This study demonstrated that the EBI treatment was able to significantly degrade AFB1 in the peanut meal. The initial AFB1 and water concentrations in the peanut meal greatly impacted the AFB1 degradation rate. The degradation of AFB1 at the selected concentrations was proven to follow pseudo first-order reaction kinetics (R2 > 0.95), and the AFB1 degradation rate was lowered as the initial AFB1 and water concentrations decreased. The Ames and cytotoxicity tests were employed to evaluate the residual toxicity of the AFB1 sub-products in the peanut meal, and the results indicate that the mutagenic activity and the cytotoxicity of EBI-treated samples were completely diminished, in comparison with that of untreated samples. Overall, EBI treatment is a potentially applicable method to effectively and safely degrade the AFB1 in the peanut meal.
Acknowledgments
The authors declare that they have no conflicts of interest.
Additional information
Funding
References
- Iys, R.;. Aflatoxin in Food and Feed: Occurrence, Legislation and Inactivation by Physical Methods. Food Chemistry 1997, 59(1), 57–67. DOI: 10.1016/S0308-8146(96)00096-9.
- Cancer, I. A. F. O. Some Naturally Occurring Substances: Food Items and Constituents, Heterocyclic Aromatic Amines and Mycotoxins. Carcinógenos 1993, 56, 41-489.
- Dandrifosse, G.; Peulen, O.; Kheffif, E.; Deloyer, P.; Grandfils, C. European Commission Directorate General DG XII Sciences Food and Agriculture Organization of the United States: USA. 2000.
- Schwartzbord, J. R.; Dan, L. B. Aflatoxin Contamination in Haitian Peanut Products and Maize and the Safety of Oil Processed from Contaminated Peanuts. Food Control 2015, 56, 114–118. DOI: 10.1016/j.foodcont.2015.03.014.
- Samarajeewa, U.; Sen, A. C.; Cohen, M. D.; Wei, C. I. Detoxification of Aflatoxins in Foods and Feeds by Physical and Chemical Methods. Journal of Food Protection 1990, 53(6), 489–501. DOI: 10.4315/0362-028X-53.6.489.
- Luo, X.; Wang, R.; Wang, L.; Li, Y.; Wang, Y. Detoxification of Aflatoxin in Corn Flour by Ozone. Journal of the Science of Food and Agriculture 2014, 94(11), 2253–2258. DOI: 10.1002/jsfa.6550.
- Milani, J.; Maleki, G. Effects of Processing on Mycotoxin Stability in Cereals. Journal of the Science of Food and Agriculture 2014, 94(12), 2372–2375. DOI: 10.1002/jsfa.2014.94.issue-12.
- Wang, S. Q.; Huang, G. Q.; Li, Y. P.; Xiao, J. X.; Zhang, Y.; Jiang, W. L. Degradation of Aflatoxin B 1 by Low-Temperature Radio Frequency Plasma and Degradation Product Elucidation. European Food Research and Technology 2015, 241(1), 103–113. DOI: 10.1007/s00217-015-2439-5.
- Liu, R. J.; Wang, R. Q.; Lu, J.; Chang, M.; Q Z, J.; Z B, D.; Wang, S. S.; Li, Q.; Wang, X. Degradation of AFB1 in Aqueous Medium by Electron Beam Irradiation: Kinetics, Pathway and Toxicology. Food Control 2016, 66, 151–157. DOI: 10.1016/j.foodcont.2016.02.002.
- Longiéras, N.; Sebban, M.; Palmas, P.; Rivaton, A.; Gardette, J. L. Degradation of Epoxy Resins under High Energy Electron Beam Irradiation: Radio-Oxidation. Polymer Degradation and Stability 2007, 92(12), 2190–2197. DOI: 10.1016/j.polymdegradstab.2007.01.035.
- Bhat, R.; Sridhar, K. R.; Tomita-Yokotani, K. Effect of Ionizing Radiation on Antinutritional Features of Velvet Bean Seeds (Mucuna Pruriens). Food Chemistry 2007, 103(3), 860–866. DOI: 10.1016/j.foodchem.2006.09.037.
- Palekar, M. P.; Cabrera-Diaz, E.; Kalbasi-Ashtari, A.; Maxim, J. E.; Miller, R. K. Effect of Electron Beam Irradiation on the Bacterial Load and Sensorial Quality of Sliced Cantaloupe. Journal of Food Science 2004, 69(9), M267–M273. DOI: 10.1111/j.1365-2621.2004.tb09941.x.
- Skowron, K.; Paluszak, Z.; Olszewska, H.; Wieczorek, M.; Zimek, Z.; Śrutek, M. Effectiveness of High Energy Electron Beam against Spore Forming Bacteria and Viruses in Slurry. Radiation Physics and Chemistry 2014, 101(31), 36–40. DOI: 10.1016/j.radphyschem.2014.04.001.
- Kim, H. J.; Jung, S.; Yong, H. I.; Bae, Y. S.; Kang, S. N.; Kim, I. S.; Jo, C. Improvement of Microbiological Safety and Sensorial Quality of Pork Jerky by Electron Beam Irradiation and by Addition of Onion Peel Extract and Barbecue Flavor. Radiation Physics and Chemistry 2014, 98(5), 22–28. DOI: 10.1016/j.radphyschem.2014.01.003.
- Rogovschi, V. D.; Aquino, S.; Nunes, T. C. F.; Gonçalez, E.; Corrêa, B. Villavicencio A L.C.H. Use Of Electron Beam On Aflatoxins Degradation In Coconut Agar. International Nuclear Atlantic Conference 2009.
- Liu, R. J.; Jin, Q. Z.; Tao, G. J.; Shan, L.; Liu, Y. F.; Wang, X. G. LC–MS and UPLC–Quadrupole Time-Of-Flight MS for Identification of Photodegradation Products of Aflatoxin B1. Chromatographia 2010, 71(1–2), 107–112. DOI: 10.1365/s10337-009-1354-y.
- Liu, R. J.; Chang, M.; Jin, Q. Z.; Huang, J. H.; Liu, Y. F.; Wang, X. G. Degradation of Aflatoxin B1 in Aqueous Medium through UV Irradiation. European Food Research and Technology 2011, 233(6), 1007–1012. DOI: 10.1007/s00217-011-1591-9.
- Wang, R. Q.; Liu, R. J.; Chang, M.; Jin, Q. Z.; Huang, J. H.; Liu, Y. F.; Wang, X. G. Ultra-Performance Liquid Chromatography Quadrupole Time-Of-Flight MS for Identification of Electron Beam from Accelerator Degradation Products of Aflatoxin B1. Applied Biochemistry and Biotechnology 2015, 175(3), 1548–1556. DOI: 10.1007/s12010-014-1377-1.
- Mosmann, T. Rapid Colorimetric Assay for Cellular Growth and Survival: Application to Proliferation and Cytotoxicity Assays. Journal of Immunology 1983, 65(s 1–2), 55–63. DOI: 10.1016/0022-1759(83)90303-4.
- Schlotzhauer, S. D.; Littell, R. C. SAS System for Elementary Statistical analysis/Sandra D. Schlotzhauer,Ramón C. Littell. Technometrics 1999, 32(2), 230–231.
- Méndez-Albores, A.; Arámbula-Villa, G.; Loarca-Piña, M. G. F.; Castaño-Tostado, E.; Moreno-Martínez, E. Safety and Efficacy Evaluation of Aqueous Citric Acid to Degrade B-Aflatoxins in Maize. Food and Chemical Toxicology International Journal of Published British Industrial Biology Research Association 2005, 43(2), 233–238. DOI: 10.1016/j.fct.2004.09.009.
- Wu, J. C.; Hseu, Y. C.; Chen, C. H.; Wang, S. H.; Chen, S. C. Comparative Investigations of Genotoxic Activity of Five Nitriles in the Comet Assay and the Ames Test. Journal of Hazardous materials 2009, 169(1–3), 492–497. DOI: 10.1016/j.jhazmat.2009.03.121.
- Maron, D. M.; Ames, B. N. Revised Methods for the Salmonella Mutagenicity Test. Mutation Research, Fundamental and Molecular Mechanisms of Mutagenesis 1983, 113(3–4), 173–215.
- CardadorMartínez, A.; CastañoTostado, E.; LoarcaPiña, G. Antimutagenic Activity of Natural Phenolic Compounds Present in the Common Bean (Phaseolus Vulgaris) against Aflatoxin B1. Food Additives and Contaminants 2002, 19(1), 62–69. DOI: 10.1080/02652030110062110.