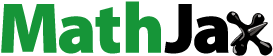
ABSTRACT
Anthocyanins exhibit natural antioxidants but exhibit poor stability and low oil solubility. Therefore, increasing the stability and fat solubility of anthocyanins is necessary to broaden their applications. In our experiment, an anthocyanin microemulsion system was constructed and its stability was investigated. At an oil phase and emulsifier ratio of 7:3, the hydrophilic oil-wet value reached 7.5 and the Km was 2:1. Furthermore, the microemulsion area was the largest. The bicontinuous region of the microemulsion exhibited optimal experimental conditions and resulted in a microemulsion containing 425.54 ± 1.58 μg/g anthocyanins. The anthocyanin microemulsion was a water-in-oil type, with a particle size of 0.07 ± 0.009 μm. Moreover, the temperature, light, and sample observation tests showed that the anthocyanin retention rate in the microemulsion was higher than that in the anthocyanin solution. Low-concentration NaCl and less than 9% of glucose and sucrose exerted no significant influence on the anthocyanin microemulsion stability.
Introduction
Microemulsions are composed of water, oil, surfactant, and cosurfactants; moreover, their homogeneous dispersion system exhibits thermodynamic stability, transparency, or translucency. Microemulsions are divided into three types, namely oil-in-water, water-in-oil (W/O), and bicontinuous.[1,2] Furthermore, microemulsions display many incomparable advantages compared with other preparations: (1) small particle size between 10 and 100 nm; (2) stable thermodynamics and is stratified after low-speed centrifugation for 30 min; and (3) microemulsion preparation can be formed spontaneously without energy.[Citation3,Citation4] Given its rapid development, microemulsion technology has been widely used in materials, cosmetics, pharmaceutical, food, and other industries.[Citation3–Citation5]
A ternary phase diagram is used to determine the composition of the microemulsion and the proportion of the oil phase, surfactant, and cosurfactant. Once the proportion is determined, a similar microemulsion can be produced. Short oil hydrocarbon chains result in deep organic phase insertions into the interface membrane.[Citation4,Citation6] Consequently, microemulsions are remarkably stable, but the long hydrocarbon chain oil phase is beneficial in increasing the dissolution. Therefore, microemulsion preparation should comprehensively consider drug dissolution. These conditions cannot be satisfied by the single oil phase; thus, different oil phases should be used.[Citation2,Citation6] The dosage and type of surfactants used in microemulsions directly influence the microemulsion formation size and toxicity. The common percentage of surfactants is 20–30% w/w. Short- and middle-chain alcohols, such as ethanol, glycerol, and n-butyl alcohol, are multiselected as cosurfactants in microemulsions.[Citation7–Citation9]
Anthocyanins are more and more welcomed to people due to its unique function, such as reducing risk for coronary heart disease[Citation10], visual improvement[Citation11], anti-mutagenic[Citation12], and anti-inflammatory effects.[Citation13] In recent years, research on the extraction and separation of anthocyanins, its antioxidant activity in vitro, and in vivo physiological activity has increased.[Citation1,Citation13] Stability studies reported that light, pH, temperature, NaCl, sugar, metal ion, and other factors affect anthocyanin solution stability.[Citation14,Citation15] Vectors (e.g., nanoliposome, microemulsion, and nanoemulsion) improve the stability of the functional ingredients and the biological activity of drugs, and current studies are focusing on creating them.[Citation1] Aditya et al. prepared a double emulsion to embed curcumin and catechin, and the results showed a remarkable enhancement of the stability and bioavailability of curcumin and catechin in a simulated gastrointestinal tract environment.[Citation13] Wu et al. studied the influence of pH and NaCl concentration on oil emulsion stability.[Citation16] Research on blueberry anthocyanin microemulsion preparation and its stability has not yet been reported. To enhance the utilization of anthocyanins, we prepared it as a microemulsion, which can be easily absorbed. The present study mainly aims to evaluate the ability of a natural surfactant to form microemulsions with anthocyanins and investigate the influence of light, temperature, sample storage, NaCl, and sugar on the properties of such a food-grade microemulsion system.
Materials and methods
Materials and reagents
Isopropyl palmitate, isopropyl myristate, decanoyl glyceride, tween 20, tween 80, span 80, glycerol, polyethylene glycol 400, ethanol, methylene blue dye, Sudan red dye, propanol, copper-covered network, NaCl, sugar, 2% phosphotungstic acid, n-butyl alcohol, NaOH, and HCl were purchased from Guangzhou Chemical Reagent Factory. Anthocyanin standard monomers, namely cyanidin-3-glucoside (Chromadex 00011605, 98.5%), delphinidin-3-rutinoside (Chromadex 00016371, 98%), and malvidin-3-galactoside (Chromadex 00017345, 99%), were obtained from Polyphenol AS (Sandnes, Norway). Chromatographic-grade formic acid and methanol were purchased from Sigma-Aldrich Co. (Billerica, MA, USA). Deionized water was used throughout the experiment.
Anthocyanin microemulsion preparation method
The surfactant, cosurfactant, oil phase, and 200 mg/mL anthocyanin water solution were mixed using a magnetic stirrer. Deionized water was added while stirring, and the mixture changed from clear to cloudy. A red and transparent microemulsion was obtained.
Screening for surfactant, oil phase, and cosurfactant
Ethanol and isopropyl myristate were used as cosurfactant and oil phase, respectively. Tween 80 and tween 20 combined with span 80 were utilized as surfactant to draw the pseudo-ternary phase diagram. The appropriate surfactant was selected according to the size of microemulsion area. Ethanol served as the cosurfactant. On the basis of surfactant screening, isopropyl palmitate, isopropyl myristate, and decanoyl glyceride were added to determine the appropriate oil phase. On the basis of screening out the surfactant and oil phase, ethanol, propanol, and n-butyl alcohol were added to select the appropriate cosurfactant.
Determination of hydrophilic–lipophilic balance value (HLB)
According to the distribution principles of surfactants, mixing of span 80 and tween 80 at different ratios produces surfactant mixture with desirable HLB value.[Citation17,Citation18] The ratio of distribution is shown in supplementary Table 1. To assign the surfactant as the emulsifier and ethanol as cosurfactant, water titration was used in each group to prepare the microemulsion. According to the size of the microemulsion area, a pseudo-ternary phase diagram was created to determine the microemulsion zone.
Determination of the ratio of surfactant and cosurfactant (Km) value
The cosurfactant and surfactant were in accordance with the mass ratios of 1:1, 2:1, 3:1, and 4:1 for blending at 25°C. The surfactant–cosurfactant mixture was the vertex of the pseudo three-element phase diagram, whereas the oil and aqueous phases were the other two vertices. The Km value was determined by the percentage of each component at the critical point, and the critical points were linked into curves by using Origin 7.5 software; the pseudo three-element phase diagram was plotted.
Transmission electron microscopic analysis of anthocyanin microemulsion
Transmission electron microscopic analysis was performed on the anthocyanin microemulsion. A drop of nanometer compound was obtained from the copper-covered network with a membrane and subjected to natural air and 2% phosphotungstic acid dye for 30 min in the copper network carrying the nanometer compound. A filter paper was used to absorb the excess dye. Afterward, the sample was scanned with a transmission electron microscope at an acceleration voltage of 80 kV.[Citation19,Citation20]
Physical properties of anthocyanin microemulsion
Anthocyanin microemulsion was centrifuged at 2000 rpm for 30 min. This microemulsion was not stratified. Methylene blue dye and Sudan red dye were used in the same volume of microemulsion. The microemulsion configurations were determined according to the red and blue dye diffusion velocities. When the red dye spread rapidly, the microemulsion can be a W/O and vice versa.[Citation16,Citation17,Citation21] The microemulsion was diluted 10 times and oscillated for mixing. The particle size distribution of microemulsion was measured using a nanoparticle analyzer at 25°C; 0.5 mL deionized water was dripped into the microemulsion and mixed at 30°C to determine the electrical conductivity. A rheometer was used to determine the viscosity of the microemulsion at a shear rate of 60 s−1 at 25°C. The pH of the microemulsion was measured using a pH meter at 25°C.[Citation22]
Anthocyanin microemulsion demulsification method
Anhydrous sodium sulfate (2.5 g) was added into 2 mL anthocyanin microemulsion and heated to 50°C. When the oil and water formed layers after 20 min, the microemulsion system was damaged. After demulsification, 3 mL of 0.1% trifluoroacetic acid–methanol solution was extracted thrice as the upper liquid. The extracted liquid was collected in a beaker. The trifluoroacetic acid–methanol solution was dried at 40°C to obtain the anthocyanins.[Citation23,Citation24]
HPLC method for determining the content of blueberry anthocyanin in microemulsion
As established in our laboratory, HPLC was used to determine the anthocyanin content in the microemulsion.[Citation25] The specific conditions were as follows: mobile phase (acetonitrile:water, which contains 0.1% trifluoroacetic acid), 75:25; flow rate, 1 mL/min; and column temperature, 30°C. Anthocyanins showed good linear relationship in the range of 0.089–9.37 mg/mL concentration. According to this method, the regression equation was Y(A) = 127349X (mg/mL)–4157 (R2 = 0.9994). A microemulsion of approximately 2 mL blueberry anthocyanin was demulsified, and 0.1% trifluoroacetic acid–acetonitrile solution was added to dissolve the anthocyanins. Reconstituted extracts were filtered through a 0.2-μm nylon membrane. Filtering was conducted prior to determination. The peak area was generated into the standard curve equation to calculate the relative anthocyanin content.
Temperature test to determine the anthocyanin retention rate
Approximately 50 mL of anthocyanin solution and anthocyanin microemulsion with the same concentration were added into beakers, which were subsequently placed in 4°C, 25°C, and 60°C without light for 10 days. The anthocyanin retention rate was determined every 2 days.
Light test to determine the anthocyanin retention rate
Approximately 50 mL of anthocyanin solution and anthocyanin microemulsion with the same concentration were added into beakers, adjusted to obtain a pH of 3 by adding 1 mol/L HCl, and stored for 25 days under natural light, ultraviolet light, and dark conditions. Demulsification was conducted on the anthocyanin microemulsion, and anthocyanin retention rate was determined every 5 days.
Sample observation test to determine the anthocyanin retention rate
Approximately 50 mL of anthocyanin solution and anthocyanin microemulsion with the same concentration were added into beakers and stored at 4°C and 25°C for 3 months. Microemulsion form was observed at 0, 1, 2, and 3 months. The anthocyanin retention rate was determined after demulsification.
Influence of NaCl on anthocyanin microemulsion stability
Anthocyanin microemulsion was placed in nine test tubes. Each tube contained 10 mL microemulsion. Afterward, different amounts of NaCl, namely 0, 0.05, 0.1, 0.2, 0.3, 0.5, 1.0, 1.5, and 1.0 mol/L, were added and dissolved completely. The test tubes were placed under 25°C for 1 h to determine the microemulsion particle size and distribution.[Citation26]
Influence of sugar on anthocyanin microemulsion stability
Glucose and sucrose (0%, 1%, 3%, 6%, and 9%) were added in five test tubes. Subsequently, the anthocyanin microemulsion was added to a final of 10 g in each tube. The test tubes were placed under 25°C for 1 h to determine the microemulsion particle size and distribution.[Citation27]
Statistical analysis
Final data are expressed as the mean ± standard deviation ( ± SD). ANOVA was used to assess the differences among groups. A t-test was performed to compare the means of the two independent samples. Statistical analysis was performed using SPSS 21.0. Significant differences were denoted by p < 0.05.
Results and discussion
Effect of surfactant on microemulsion formation zone
Surfactant can reduce the interfacial tension and structure interfacial film to promote the formation of microemulsion. Previous studies shown that the proportion of surfactants in the microemulsion system were relatively high, ranged from 10% to 30%.[Citation21] According to , when tween 80/span 80 was used as surfactant, microemulsion area was the largest. Stability was lost when a component changed in the microemulsion system. Tween 80/span 80 was utilized as surfactant to adjust the microemulsion system well.
Effect of oil phase on microemulsion formation zone
The microemulsion area was optimal when HLB was 7.0 and with isopropyl palmitate as the oil phase (). Oil phase size is an important factor in microemulsion formation because both oil and surfactant penetrate to form an oil film, which affects the microemulsion formation. An oil phase with an appropriately long chain easily embeds into the interface between the oil and water layers to form a stable micellar microemulsion. Thus, this phase is conducive to the formation of microemulsion.[Citation2] Compared with the tween 80 and span 80 distribution system, isopropyl palmitate exhibited suitable chain length and volume size to form a microemulsion. Moreover, isopropyl palmitate is a safe oil phase and is often chosen for oral drugs or as a functional composition for microemulsion carriers.[Citation28]
Effect of cosurfactant on microemulsion formation zone
Ethanol, propanol, and n-butyl alcohol were used as cosurfactants to prepare the microemulsion, as shown in . An appropriate cosurfactant can be placed between the surfactants to benefit the formation of a mixed adsorption layer to promote the formation of microemulsion. Ethanol, propanol, and n-butyl alcohol, as cosurfactants, can all form microemulsion systems. These cosurfactants are all short-chain –OH alcohols. Therefore, one –OH alcohol used as a cosurfactant, together with an increasing number of carbon atom, should result in an excellent performing emulsion. Nevertheless, with extensions in the carbon chain, the weak –OH alcohol polarity causes considerable toxicity to humans.[Citation16,Citation23] Therefore, considering safety and convenience, we used ethanol as the surfactant.
Effect of HLB and Km on microemulsion formation zone
When ethanol was used as cosurfactant, it existed in the interface membrane under low concentration. With the increased ethanol concentration, oil and water phases showed a remarkable relationship, thereby increasing the microemulsion stability. However, the excess ethanol was soluble in water phase, which broke the interface membrane between the oil phase and water phase and led to the demulsification of microemulsion.[Citation2,Citation28] The small surfactant content resulted in considerable influence of Km values on the formation of microemulsion. The microemulsion region was optimal only at a Km value of 2:1. Moreover, the microemulsion system was considerably stable ().
Effects of tween 80 and span 80 composite emulsifiers with different HLB values on the microemulsion stability and region were investigated, as shown in . When the HLB was 7.5, the microemulsion area was optimal. The low HLB value of the surfactant showed weak hydrophilicity, which was unfavorable to the formation of hydrogen bonds between the water phase and surfactant. Such weak hydrophilicity reduced the water load in the microemulsion and subsequently influenced the microemulsion phase areas. The high HLB value due to the weak lipophilicity was not conducive to the formation of micelles because of the interface layer and oil phase permeability, which affected the formation of microemulsion.[Citation3,Citation7]
Evident morphology of blueberry anthocyanin microemulsion
The anthocyanin microemulsion was dark red and transparent with good liquidity. Anthocyanin microemulsion was not hierarchical after 4000 rpm centrifugation for 30 min and thus identified as a microemulsion. Sudan red dye showed faster diffusion velocity than that of methylene blue dye in the microemulsion, thereby confirming that the anthocyanin microemulsion was a W/O type. According to the HPLC result of anthocyanin standard, anthocyanin microemulsion, and blank microemulsion, the content of anthocyanin in the microemulsion was 425.54 ± 1.58 μg/g. The size of microemulsion was 0.07 ± 0.009 μm, which was less than 0.1 μm and under the microemulsion category.[Citation5,Citation16] The anthocyanin microemulsion particle size distribution was direct, narrow, and normally distributed (), which suggested an ideal microemulsion preparation. The transmission electron microscopy result of anthocyanin microemulsion was shown in . Anthocyanin microemulsion, as a single entity rather than in cluster form, presents a spherical particle size with a dispersed system. Moreover, other physical and chemical properties of the blueberry anthocyanin microemulsion, including conductivity (321 μs/cm), refractive index (1.379 nd), viscosity (223 mPa.s), and pH value (3.8), were measured. According to the microemulsion quality standard system, particle size distribution, conductivity, viscosity, refractive index, and pH value are the most common indexes to evaluate the quality of a microemulsion.[Citation7]
Temperature stability of blueberry anthocyanin microemulsion
showed that anthocyanins could be preserved for a long time under 4°C. Anthocyanin stability time remained higher than 85% after 10 days. As temperature increased, the anthocyanin stability decreased. Temperature has a significant effect on the stability of anthocyanin. A rise in temperature promotes the conversion of the positive ions of anthocyanin into chalcone and consequently leads to anthocyanin degradation. When the anthocyanin solution was under 60°C, the anthocyanin retention rate was only 40% after 10 days (p < 0.05). According to , the anthocyanins showed a slightly improved retention rate after microemulsion embedding under 4°C and 25°C. Anthocyanins exhibited a significantly increased retention rate from 43.1% to 62.4% at 60°C for 10 days after microemulsion embedding (p < 0.05). The improved microemulsion embedding can improve the thermal stability of anthocyanins.
Light stability of blueberry anthocyanin microemulsion
According to , the anthocyanin retention rate slowly decreased under dark storage. The retention rate remained higher than 60% after 25 days (p > 0.05). This rate rapidly decreased under natural and ultraviolet light (p < 0.05). This result illustrated that light decreases anthocyanins, which could be because exposure to light energy changes the anthocyanin molecular structure and eventually causes anthocyanin degeneration. showed that the anthocyanin retention rate generally increased after microemulsion embedding. This observation suggested that microemulsion embedding can increase light stability (p < 0.05), possibly because the value of the refractive index of anthocyanin microemulsion (1.379) was similar to that of the aqueous solution (1.333), which was higher than that of the anthocyanin solution (1.124).
Figure 5. Effects of light on anthocyanin and its microemulsion (A: anthocyanin solution, B: anthocyanin microemulsion).
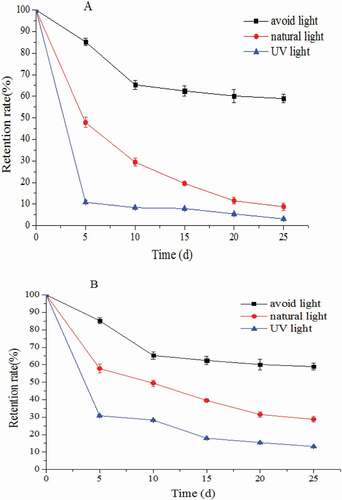
The anthocyanin microemulsion could accept light energy, but light energy was absorbed in the oil phase and cosurfactant and away from the microemulsion, and this could guarantee the anthocyanin stability.[Citation2,Citation18] The appearance of anthocyanin microemulsion was unchanged under 4°C and 25°C. This microemulsion was dark red and uniformly transparent with good liquidity. illustrated that the anthocyanin retention rates decreased in the anthocyanin microemulsion and anthocyanin solution and that the decrease rate was high under 25°C. The decrease in anthocyanin retention rate in the microemulsion was lower than that in the anthocyanin solution (). This result showed that anthocyanin stability was enhanced after microemulsion and the storage stability was improved under 4°C ().
NaCl stability of blueberry anthocyanin microemulsion
showed that 0–1.0 mol/L NaCl exerted no evident effect on the stability of anthocyanin microemulsion and that the microemulsion particle size was almost the same. In 1.5 and 2.0 mol/L NaCl, the size of anthocyanin microemulsion was significantly increased (p < 0.01), which may be because NaCl enhanced the interaction with water to damage the emulsion system.
Sugar stability of blueberry anthocyanin microemulsion
Sugar is a polar organic compound. Large sugar concentrations will lower water activity and decrease emulsion stability.[Citation16,Citation29] and showed that when less than 9% of glucose and sucrose were combined, the anthocyanin microemulsion particle size was almost the same. This result suggested that sugar exerted no significant effect on the stability of microemulsion.
Conclusion
Pseudo-ternary phase diagram was created to determine the optimal formula of anthocyanin microemulsion: isopropyl myristate as oil phase, tween 80/span 80 distribution as surfactant, ethanol as cosurfactant, HLB = 7.5, and Km = 2:1. Anthocyanin microemulsion appeared dark red and clear. This microemulsion was a W/O type with good liquidity. Anthocyanin content in microemulsion was 425.54 μg/g, the particle size was 0.07 μm, and the droplets were round balls with a uniform size. During temperature, light, and sample observation experiments, the decrease in anthocyanin retention rate in the microemulsion was lower than that in the anthocyanin solution. This observation indicated that the anthocyanin stability was enhanced after the microemulsion, and low-temperature storage stability was an ideal option. Common food additives, such as low-concentration NaCl (less than 1.0 mol/L) and less than 9% concentration of glucose and sucrose, exerted no evident effect on the stability of anthocyanin microemulsions.
Declaration of interest
The authors declare that they have no conflict of interest.
Supplemental Table
Download ()Supplementary material
Supplemental data for this article can be accessed here.
Additional information
Funding
References
- Yang, X.; Tian, H.; Ho, C. T.; Huang, Q. Inhibition of Citral Degradation by Oil-In-Water Nanoemulsions Combined with Antioxidants. Journal of Agricultural and Food Chemistry 2011, 59, 6113–6119. DOI:10.1021/jf2012375.
- Acharya, B.; Guru, P. S.; Dash, S. Tween-80-N-Butanol-Diesel-Water Microemulsion System—A Class of Alternative Diesel Fuel. Journal of Dispersion Science and Technology 2014, 35, 1492–1501. DOI:10.1080/01932691.2013.858348.
- Margulis, K.; Srinivasan, S.; Ware, M. J.; Summers, H. D.; Godin, B.; Magdassi, S. Active Curcumin Nanoparticles Formed from a Volatile Microemulsion Template. Journal of Materials Chemistry B 2014, 2, 3745–3752. DOI:10.1039/c4tb00267a.
- Pemartin, K.; Solans, C.; Alvarez-Quintana, J.; Sanchez-Dominguez, M. Synthesis of Mn-Zn Ferrite Nanoparticles by the Oil-In-Water Microemulsion Reaction Method. Colloids and Surfaces a-Physicochemical and Engineering Aspects 2014, 451, 161–171.
- Kazerooni, H.; Rouhi, A.; Khodadadi, A. A.; Mortazavi, Y. Effects of Combustion Catalyst Dispersed by a Novel Microemulsion Method as Fuel Additive on Diesel Engine Emissions, Performance, and Characteristics. Energy & Fuels 2016, 30, 3392–3402. DOI:10.1021/acs.energyfuels.6b00004.
- Chang, C. W.; Chen, Y. C.; Liu, C. Y. Separation and On-Line Preconcentration of Nonsteroidal Anti-Inflammatory Drugs by Microemulsion Electrokinetic Chromatography. Electrophoresis 2015, 36, 2745–2753. DOI:10.1002/elps.201500160.
- Huang, X.; Jiang, G.; Deng, Z. Oil Extraction from Oil-Contaminated Drill Cuttings Using a Recyclable Single-Phase O/W Microemulsion. Tenside Surfactants Detergents 2015, 52, 454–463. DOI:10.3139/113.110397.
- Hunt, S. T.; Roman-Leshkov, Y. Reverse Microemulsion-Mediated Synthesis of Monometallic and Bimetallic Early Transition Metal Carbide and Nitride Nanoparticles. Jove-Journal of Visualized Experiments 2015. DOI:10.3791/53147.
- Iwunze, M. O.;. Enhancement of Curcumin Fluorescence by Ascorbic Acid in Bicontinuous Microemulsion. Journal of Fluorescence 2015, 25, 891–895. DOI:10.1007/s10895-015-1569-2.
- Sun, J.; Liu, R. H. Apple Phytochemical Extracts Inhibit Proliferation of Estrogen-Dependent and Estrogen-Independent Human Breast Cancer Cells through Cell Cycle Modulation. Journal of Agricultural and Food Chemistry 2008, 56, 11661–11667. DOI:10.1021/jf8021223.
- Matsumoto, H.; Nakamura, Y.; Tachibanaki, S.; Kawamura, S.; Hirayama, M. Stimulatory Effect of Cyanidin 3-Glycosides on the Regeneration of Rhodopsin. Journal of Agricultural and Food Chemistry 2003, 51, 3560–3563. DOI:10.1021/jf034132y.
- Lou, H.; Hu, Y.; Zhang, L.; Sun, P.; Lu, H. Nondestructive Evaluation of the Changes of Total Flavonoid, Total Phenols, ABTS and DPPH Radical Scavenging Activities, and Sugars during Mulberry (Morus Alba L.) Fruits Development by Chlorophyll Fluorescence and RGB Intensity Values. LWT-Food Sci Technol 2012, 47, 19–24. DOI:10.1016/j.lwt.2012.01.008.
- Aditya, N. P.; Macedo, A. S.; Doktorovov, S.; Souto, E. B.; Kim, S.; Chang, P.-S.; Ko, S. Development and Evaluation of Lipid Nanocarriers for Quercetin Delivery: A Comparative Study of Solid Lipid Nanoparticles (SLN), Nanostructured Lipid Carriers (NLC), and Lipid Nanoemulsions (LNE). LWT-Food Sci Technol 2014, 59, 115–121. DOI:10.1016/j.lwt.2014.04.058.
- Tiwari, B. K.; O’Donnell, C. P.; Patras, A.; Cullen, P. J. Anthocyanin and Ascorbic Acid Degradation in Sonicated Strawberry Juice. Journal of Agricultural and Food Chemistry 2008, 56, 10071–10077. DOI:10.1021/jf801824v.
- Yang, Y.; Yuan, X. H.; Xu, Y. Q.; Yu, Z. Y. Purification of Anthocyanins from Extracts of Red Raspberry Using Macroporous Resin. International Journal of Food Properties 2015, 18, 1046–1058. DOI:10.1080/10942912.2013.862632.
- Wu, Q.; Uluata, S.; Cui, L.; Wang, C.; Li, D.; McClements, J.; Decker, E. A. Physical and Oxidation Stability of Self-Emulsifying Krill Oil-In-Water Emulsions. Food & Function 2016, 7, 3590–3598. DOI:10.1039/C6FO00045B.
- Abbasi, S.; Radi, M. Food Grade Microemulsion Systems: Canola Oil/Lecithin: N-Propanol/Water. Food Chemistry 2016, 194, 972–979. DOI:10.1016/j.foodchem.2015.08.078.
- Nazar, M. F.; Saleem, M. A.; Bajwa, S. N.; Yameen, B.; Ashfaq, M.; Zafar, M. N.; Zubair, M. Encapsulation of Antibiotic Levofloxacin in Biocompatible Microemulsion Formulation: Insights from Microstructure Analysis. Journal of Physical Chemistry B 2017, 121, 437–443. DOI:10.1021/acs.jpcb.6b09326.
- Chen, J.; Duan, C.; Guan, Y. Sorptive Extraction Techniques in Sample Preparation for Organophosphorus Pesticides in Complex Matrices. Journal of Chromatography B-Analytical Technologies in the Biomedical and Life Sciences 2010, 878, 1216–1225. DOI:10.1016/j.jchromb.2010.02.031.
- Jenkins, A. L.; Yin, R.; Jensen, J. L. Molecularly Imprinted Polymer Sensors for Pesticide and Insecticide Detection in Water. The Analyst 2001, 126, 798–802. DOI:10.1039/b008853f.
- Wang, W.; Cai, Y.; Liu, Y.; Zhao, Y.; Feng, J.; Liu, C. Microemulsions Based on Paeonol-Menthol Eutectic Mixture for Enhanced Transdermal Delivery: Formulation Development and in Vitro Evaluation. Artificial Cells, Nanomedicine, and Biotechnology 2017, 45, 1–6. DOI:10.1080/21691401.2016.1226178.
- Naeem, M.; Rahman, N. U. R.; Tavares, G. D.; Barbosa, S. F.; Chacra, N. B.; Loebenberg, R.; Sarfraz, M. K. Physicochemical, In Vitro and In Vivo Evaluation of Flurbiprofen Microemulsion. Anais Da Academia Brasileira De Ciencias 2015, 87, 1823–1831. DOI:10.1590/0001-3765201520130436.
- Duangjit, S.; Chairat, W.; Opanasopit, P.; Rojanarata, T.; Panomsuk, S.; Ngawhirunpat, T.; Development, C. Skin Interaction of Capsaicin-Loaded Microemulsion-Based Nonionic Surfactant. Biological & Pharmaceutical Bulletin 2016, 39, 601–610. DOI:10.1248/bpb.b15-00961.
- Pirogov, A.; Sokolova, L.; Sokerina, E.; Tataurova, O.; Shpigun, O. Determination of Flavonoids as Complexes with Al3+ in Microemulsion Media by HPLC Method with Fluorescence Detection. Journal of Liquid Chromatography & Related Technologies 2016, 39, 220–224. DOI:10.1080/10826076.2016.1147462.
- Chen, J.; Zhao, Y.; Tao, X. Y.; Zhang, M.; Sun, A. D. Protective Effect of Blueberry Anthocyanins in a CCL4-induced Liver Cell Model. LWT-Food Sci Technol 2015, 60, 1105–1112. DOI:10.1016/j.lwt.2014.10.010.
- Kopjar, M.; Orsolic, M.; Pilizota, V. Anthocyanins, Phenols, and Antioxidant Activity of Sour Cherry Puree Extracts and Their Stability during Storage. International Journal of Food Properties 2014, 17, 1393–1405. DOI:10.1080/10942912.2012.714027.
- Turfan, O.; Turkyilmaz, M.; Yemis, O.; Ozkan, M. Effects of Clarification and Storage on Anthocyanins and Color of Pomegranate Juice Concentrates. Journal of Food Quality 2012, 35, 272–282. DOI:10.1111/j.1745-4557.2012.00451.x.
- Wang, Z.; Mu, H. J.; Zhang, X. M.; Ma, P. K.; Lian, S. N.; Zhang, F. P.; Chu, S. Y.; Zhang, W. W.; Wang, A. P.; Wang, W. Y.; et al. Lower Irritation Microemulsion-Based Rotigotine Gel: Formulation Optimization and in Vitro and in Vivo Studies. International Journal of Nanomedicine 2015, 10, 633–644.
- Randar, A.; Almasi-Kashi, M. Dynamic and Spectroscopic Studies of Nano-Micelles Comprising Dye in Water/Dioctyl Sodium Sulfosuccinate/Decane Droplet Microemulsion at Constant Water Content. Journal of Molecular Structure 2017, 1128, 257–262. DOI:10.1016/j.molstruc.2016.08.076.