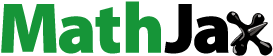
ABSTRACT
The effect of heating temperature and duration on the texture and protein composition of bighead carp (Aristichthys nobilis) muscle was investigated. Samples were heated at 90°C, 100°C, 110°C, and 120°C for different times. The results showed that cooking loss increased significantly with increasing temperature and heating time, and a fractional conversion model was used to describe the increase. Hardness, chewiness, and gumminess exhibited a similar four-phase change with two peaks at 90°C, 100°C, and 110°C, and the trend was not observed at 120°C. Analysis of sodium dodecyl sulfate polyacrylamide gel electropheresis (SDS-PAGE) revealed that contents of myosin heavy chain decreased entirely to disappear and contents of actin significantly decreased. Increasing the intensity of the heavy molecular weight bands indicated the aggregation of myosin. Content of water-soluble proteins presented an increasing trend, which reflected the protein degradation and the release of low-molecular-weight compounds.
Introduction
Bighead carp (Aristichthys nobilis) is one of the most important commercial aquaculture fish in China. Global production of farmed bighead carp was 3,253,143 tons in the year of 2014.[Citation1] The rapid growth, high yield, and low price of bighead carp make it attractive for processing into products. Nowadays, there is a growing demand for convenient foods, which do not need to undergo any treatment before consumption and are popular among consumers,[Citation2,Citation3] such as braised fish head in casserole cans and anchovies in tomato sauce on flexible packaging forms. To produce these instant products, longtime shelf life is a very important goal, which can be realized by sterilization. Products after sterilizing can be stored at normal temperature and have longer shelf life.[Citation4]
Thermal processing is one of the major processing approaches for preserving the nutritional value. Depending on the food storage conditions and the food properties, atmospheric pressure sterilization, high pressure sterilization, and other forms of sterilization are usually used. However, no matter what form of sterilization do have negative influences on products’ qualities, such as texture deterioration, color variation, flavor changes, loss of nutritional ingredients, and damages on sensory quality. Compared with marine fish and animal products, freshwater fishes have smaller muscle fiber diameter, less connective tissue, and tender taste,[Citation5] which lead to poor tolerance to heat and broken fiber muscles after heat sterilization. Lots of articles reported the effects of thermal processing on poultry meat and sea fish. Barbanti and Pasquini[Citation6] found that short cooking time (4 min) and temperatures of 130–150°C resulted in lower cooking loss and best meat tenderness in both not marinated and marinated chicken breast meat. Dai et al.[Citation7] found that ohmic heating-cooked meat had better color appearance, slightly enhanced hardness, and higher values of redness than water bath-cooked meat. According to Christensen et al.,[Citation8] whole meat shear force of beef semitendinosus was found to increase in two separate phases from 40°C to 50°C and again from 60°C to 80°C with a decrease in Warner-Bratzler shear force from 50°C to 60°C, which was similar with results found by Combes et al.[Citation9] and Schubring.[Citation10] An increase in collagen solubility and tenderness scores with decrease in shear force value and collagen content of semimembranosus muscle was observed with increases in cooking temperature and time.[Citation11] Kong et al.[Citation12] and Kong et al.[Citation13] evaluated the kinetics of reactions leading to changes in salmon quality during thermal processing and the sample color became less light as heating time increased from 10 to 60 min. High-temperature treatments damaged the main secondary protein (random coil) and protein fractions (MHC and actin) of the Pollock gels as temperature increases from 100°C to 120°C, leading to the protein aggregation and fragile network frames with bigger holes.[Citation3,Citation4] However, pieces of research of high temperature heating processing on freshwater fish are rare.
During the pasteurization or sterilization process, the main parameter which could be controlled was a combination of temperature and time.[Citation14] Pulgaretet et al.[Citation15] found that the combination of cooking temperature and time seemed to be more important in the textural and color parameters of pork cheeks. Therefore, different heating temperatures and heating times mean different intensities of sterilization or thermal intensity on meat quality. It is of great importance in practical significance and theoretical value to improve the qualities of canned fish products through heating sterilization, exploring the varying patterns under different heating temperatures with the heating time of muscle and designing the reasonable combination of heating time and temperature or sterilization technology for less damage to meat qualities.
The objective of this paper was to investigate effects of heating temperature and heating time on the quality of bighead carp muscle by analyzing texture, cooking loss, and changes in protein composition. This may design heating sterilization technology rationally and provide a theoretical basis for improving qualities of fish products after heating treatment.
Materials and methods
Materials
Live samples of bighead carp (Aristichthys nobilis) were purchased from Huarunwanjia Supermarket in Binhu District, Wuxi, Jiangsu, China. The fish were 2.5 ± 0.1 kg in weight, having similar color, firmness, odor, and overall appearance, and transported to the laboratory in ice. Then the fish were killed by a physical blow to the head, eviscerated, and washed. The skin was removed and the muscles were trimmed of visible fat and connective tissue. Picked fish back muscles were away from more than 5 mm of the skin and avoiding the fishbone part.
Tetramethylethylenediamine (TEMED) was purchased from Sigma-Aldrich (Shanghai, China). Protein molecular weight marker was obtained from TaKaRa Biotechnology Co., Ltd. (Dalian, China). NaCl, EDTA, MgCl2, NaOH, and formalin were of analytical grade and were purchased from Sigma-Aldrich (St. Louis, Mo, USA) of Sinopharm Chemical Reagent Co., Ltd. (Shanghai, China).
Sampling
The dorsal part muscles were cut to the size 3.0 × 2.0 × 1.0 cm with the fibers parallel to the longest axis. The fish blocks were individually weighed and packed into retort pouch (PET/CPP) with laboratory vacuum sealing machine (Golden Bridge Technology Co., Ltd., Guangdong, China). The vapor and oxygen permeability of the pouch were 3.59 g/(m· 24 h) and 97 cm3/(m2 · 24 h· 0.1 MPa), respectively. Samples were kept at 4°C for further analysis.
A water bath and a retort were used for the high-temperature treatment of samples. In this study, the samples heated in the water bath, at 90°C and 100°C, were placed horizontally and pressed under the water surface with a stainless steel frame, and those in retort, at 110°C and 120°C, were vertically placed at the bottom of the retort basket. Samples were heated with different high temperatures to make their central temperature achieve 90 ± 0.5°C, 100 ± 0.5°C, 110 ± 0.5°C, and 120 ± 0.5°C, respectively, with a thermocouple and kept for the corresponding heating time. After heating treatments, the samples in the water bath were took out and subsequently cooled in flowing water to room temperature, and in a retort samples were cooled automatically by the equipment with cooling water, and then kept refrigerated (4°C) until tested. Heating time schedule at different temperatures is provided in ; different duration times were aim to explore rules of changes between different temperatures under similar heating intensity.
Table 1. Heating time schedule at different temperatures.
Determination of the moisture and cooking loss
The moisture content was carried out according to Association of Official Agricultural Chemists (AOAC).[Citation16] The content of protein was determined by the biuret method according to Gornallet et al.[Citation17] Cooking loss was calculated as the percent in weight differences between the raw and cooked fish blocks.[Citation18] After thermal processing, the samples were first left until their temperature reaches the room temperature (25°C) and then weighted. According to the method of Kong et al.,[Citation13] cooking loss could be estimated using a fractional conversion model, in which a quality index, f, is used to express the extent of quality change at any time t:
For a first-order reaction, the logarithm of (1 – f) plotted against time is linear. The model becomes
where C0 represents the initial value at time zero, Ct is the value at time t, k is the rate constant, and C∞ is the non-zero equilibrium quality property after prolonged heating time. The temperature dependence of the rate constant was expressed by the Arrhenius equation:
Instrumental texture profile analysis (TPA)
Texture analysis of samples was carried out using a TA.XT Plus texture analyzer (Stable Micro systems, UK) as described by Dai et al.[Citation7] Samples were prepared at room temperature (20–25°C) before analysis and placed in the texture analyzer equipped with a flat-ended cylindrical probe P25 (25 mm diameter). Each sample was compressed twice at a constant depression speed of 1 mm/s to 30% of original height with a holding time of 5 s. In addition, the cross-head speed was 3 mm/s, the later speed was 5 mm/s, and the type of loaded probe was Auto-5 g. The measurements were conducted in six replications.
SDS-PAGE analysis
Cooked muscle samples (3 g) were homogenized in 5% SDS (27 mL) at 11,000 rpm for 60 s with a high-speed Ultra-Turrax homogenization mixer (IKA, Guangzhou, China). All mixtures were incubated at 85°C for 1 h and then centrifuged at 6100 × g for 10 min. The protein content of the supernatant was analyzed according to the Biuret method[Citation19], and adjusted to 18 mg/mL SDS-PAGE was carried out by the method reported in the literature.[Citation20,Citation21] The supernatants were dissolved at a ratio of 1:1 (v/v) with the SDS-PAGE sample buffer (0.125 mol/L Tris-HCl (pH 6.8), containing 4% (w/v) SDS, 10% (v/v) β-mercaptoethanol, 20% (v/v) glycerol, and 0.005% (w/v) bromophenol blue) and boiled for 5 min. The samples (10 μL, 9 mg/mL) were loaded onto the 4% stacking and 10% separating gels and then subjected to electrophoresis using a mini vertical apparatus (Bio-Rad, USA). After electrophoresis, the gels were stained with 0.125% (w/v) Coomassie Brilliant Blue R-250 in 50% (v/v) methanol and 10% (v/v) acetic acid, and then discolored with 50% methanol and 10% acetic acid for 2–3 h until the background is clear.
Extraction of the water-soluble proteins
The protein components in fish were fractionated according to the method of Chin et al.[Citation22] with a slight modification. Chopped samples (4 g) were added into 10 mL 50 mmol/L cold phosphate buffer (0.1 mol/L NaCl, 1 mmol/L EDTA, 2 mmol/L MgCl2, pH 7.0) using a high-speed Ultra-Turrax homogenization mixer (IKA, Guangzhou, China) at 10,000 rpm for 2 min. The homogenate was centrifuged at 8000 rpm for 15 min at 4°C. The extraction process was repeated twice. The supernatants were collected, which were the water-soluble proteins.
Statistical analyses
A completely randomized design was used in this study. For all parameters, the determinations were done in duplicate, except for cooking loss, TPA and Shear force value (SFV) analysis, where the analyses were carried out with six determinations. Statistical analysis was performed using SAS version 8.0 (1999, SAS Institute, Inc., Cary, NC, USA). ANOVA was performed using the general linear model procedure. Comparison of group means was obtained by Duncan’s multiple range test.
Results and discussion
Cooking loss and moisture content analysis
) shows the cooking loss and ) shows the moisture content under different heating temperatures and heating times of bighead carp muscles. The cooking loss is one of the most common phenomena in thermal processing. According to Purslow et al., meat lost volume and weight during the cooking process by expulsion of fluid.[Citation23] This change in fluid content brought about modifications in the textural qualities of meat which were, in addition to the heat-induced changes in protein and fat, leading to rough taste and poor economic benefits. Four cooking loss curves of 90°C, 100°C, 110°C, and 120°C showed an upward trend in ), indicating greater cooking loss with higher temperature at the same heating time. This might be due to random aggregation caused by the thermal shock and fiber contraction. Cooking loss increased with a drastic rate at the beginning, and then showed almost no changes, or remained unchanged, when the heating time reached 80, 55, 50, and 40 min at four heating temperatures of 90°C, 100°C, 110°C, and 120°C, respectively. Thermal denaturation of muscle proteins was the primary mechanism leading to the cooking loss.[Citation24] Heating caused denaturation of myosin and shrinkage of myofibrils, leading to the loss of moisture; thereby, cooking loss increased sharply and mostly occurred at the beginning. After that, the loss gradually reached equilibrium, which was 28.6%, 30.8%, 32.8%, and 34.8% for 90°C, 100°C, 110°C, and 120°C, respectively. The changes of equilibrium cook loss C∞ with heating temperature followed the below relationship:
C∞ = 0.206t + 10.12, R2 = 0.9994 (4)
The rate constants corresponding to the four temperatures, Ea and k0 values, and the coefficient of correlation are shown in . The activation energy of 32.33 kJ/mol is similar to that of salmon reported by Kong[Citation13] to be 36.98 kJ/mol, and the frequency factor k0 is 1119.7/min. After incorporating the above values, the equation becomes
Table 2. First-order kinetic parameters for cooking loss of bighead carp after heating treatments at four temperatures.
Cooking loss can then be estimated using the equation
Ct = (1–e–kt)× C∞ (6)
This model gives a good fit to the cooking loss data when samples were heated from 90°C to 120°C. The result for the moisture content of the bighead carp muscle is shown in (b). As heating temperature and heating time increased, the moisture content of the bighead carp muscle decreased slightly, and then followed by no significant changes, which was in accordance with the changes of cooking loss.
Texture profile analysis
shows the results of TPA of bighead carp. Figs. 2(a–2(c) show changes in hardness, chewiness, and gumminess under different heating intensities, respectively. The three indicators showed a similar four-phase change with two peaks at 90°C, 100°C, and 110°C, while the values of the second peak were small or not obvious, which was also reported in the study of salmon by Kong et al.[Citation13] In the range of 5–15 min, a gradual increasing trend was observed, which might due to the thermal denaturation of the protein lead to random aggregation and dehydration of muscle fibers, resulting in hardening of the meat, and the subsequent decline might be mainly due to the structural changes and dissolution of collagen, leading to the loose muscle fibers. The collagen solubility increased with temperature and time of cooking in the water baths as found by Vasanthi et al.[Citation11] The second peaks might be caused by the aggregation of protein, as seen from SDS-PAGE data, shown in , which was in agreement with the result found by Zhang et al.[Citation25] However, with the prolongation of heating time, the degradation of protein leading to the muscle fiber bundles was seriously damaged; muscle fiber fractured and became loose, and eventually leading to the decreased hardness. And the increase of water-soluble protein () reflected the protein degradation and the release of low-molecular-weight nitrogen compounds.[Citation25]
Figure 3. Changes in protein SDS-PAGE profiles of bighead carp muscle with different heating times at 90°C, 100°C, and 120°C.
M: molecular weight standards, R: raw bighead carp meat protein. (a) a–g: 5, 20, 40, 70, 100, 130, and 160 min, respectively, heating at 90°C; (b) a–g: 5, 15, 30, 55, 80, 105, and 130 min, respectively, heating at 100°C; (c) a–g: 5, 15, 30, 50, 70, 90, and 120 min, respectively, heating at 110°C; (d) a–g: 5, 15, 20, 40, 60, 80, and 100 min, respectively, heating at 120°C
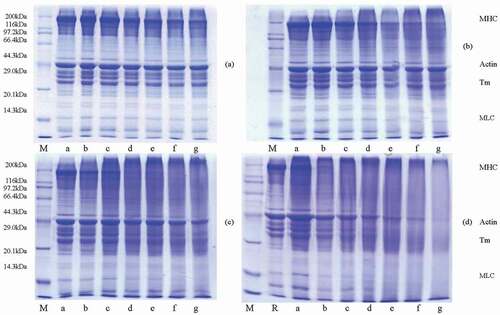
In general, peak valleys and second peaks found in curves turned forward with the increasing treating temperature, and the peak values became less obvious with the increasing temperature. On the other hand, there was no drastically decrease in hardness under lower temperatures, such as 90°C and 100°C, even heating for a long time (> 100 min), while it decreased seriously when temperature increased. Traditional thermal sterilization technology was directly putting fish on constant temperature sterilization, such as 121.1°C, but when the central temperature reached 121.1°C, the outside of fish muscle has been under a long-time heating treatment and would damage greatly in quality. Based on the above results, the idea of gradient sterilization procedure was put forward, such as setting heating gradient as 90–100–110–120°C, holding a certain time at each temperature, in order to reduce heating intensity of fish meat surface and then to improve textural qualities after sterilization. As shown in (d)–2(f), the springiness, adhesiveness, and cohesiveness declined fluctuating as heating time increased. No significant changes in springiness were displayed between different temperatures.
Sodium dodecyl sulfate-polyacrylamide gel electrophoresis analysis
The SDS-PAGE electrophoresis analysis on fish with the different heating intensities was conducted to estimate the changes in the protein composition. )–) show the SDS-PAGE electrophoretic analysis under different heating times at 90°C, 100°C, 110°C, and 120°C, respectively. Significantly changes in bighead carp protein composition were observed along with increasing heating temperature and heating time. Many protein bands were gradually thinner and lighter or even disappeared.
As shown in ), there were no obvious bands for protein to be thinner or lighter after heating for 40 min at 90°C, which indicated that the protein structure of bighead carp did not change significantly. When heating time reached above 70 min, myosin heavy chain (MHC, 200 kDa) bands appeared thinner and the trend intensified with the prolongation of heating time. As can be seen in )–), with the increase of heating temperature, the time of MHC bands turning to be thinner was gradually earlier, which were 30 min (100°C), 15 min (110°C), and 5 min (120°C), respectively. Moreover, when the heating time was 50 min (110°C) and 10 min (120°C), MHC bands were slightly visible and disappear in 90 and 20 min, respectively, which was in accordance with the study reported by Runglerdkriangkrai et al.[Citation26], who found that protein bands of MHC in fish balls were remarkable lighter when F0 was 3 min under sterilization at 116°C, and almost disappeared when time reached 6 min. With increase in heating temperature from 100°C to 120°C for 10 min, MHC in SDS-PAGE analysis of Alaska Pollock surimi gel gradually disappeared.[Citation27]
The thermal stability of actin protein was found to be remarkable higher than that of myosin protein above four temperatures. The actin bands (43 kDa) were observed slightly thinner only in 90°C and 100°C later heating period and significantly thinner in heating time reaching 90 min of 110°C and 40 min of 120°C, while they were still noticeable even when heating to 120°C for 100 min. This result was consistent with the findings of Runglerdkriangkraiet et al.,[Citation28] which reported that the disappearance of actin bands was slower than that of myosin protein during thermal processing. In addition, many bands in the range of broad molecular weights gradually disappeared or became thinner. In the later heating period of 110°C and 120°C, few bands were remaining, such as actin bands. These phenomena above revealed that myosin degradation might happen, which was in agreement with the improvement of protein solubility ().
However, as the heating temperature and the heating time increased, the bands of the heavy molecular weight (above 200 kDa) were found, and the appearing times of 90°C, 100°C, 110°C, and 120°C were 100, 55, 30, and 5 min, respectively. It could be explained by the formation of protein aggregation due to protein cross-linking as found by Zhang et al.[Citation25] With the extension of heating time, the colors of bands (above 200 kDa) turned darker first, and then become thinner after 90 and 80 min of 110°C and 120°C, respectively, which might be due to the serious degradation caused by the prolonged heating time. The increase in protein solubility in reflected the degradation of protein.
The muscle fiber break might be directly caused by protein degradation, leading to poor indexes, such as hardness. On the contrary, protein aggregation would make shrink of muscle fiber bundle and increase the hardness. Therefore, it was presumed to be interactive effects between degradation and aggregation, which might commendably explain why the emergence of the double peak value happened (). The previous study also found that under high-temperature conditions, the changes in texture and network structure were related to the degradation of collagen.[Citation29]
Protein solubility analysis
shows the effects of protein solubility under different heating intensities. Previous studies have found that long-term heating would lead to protein denaturation, producing lower molecular weight component and thereby affecting solubility.[Citation25] The trend of water-soluble protein in muscle was increasing. The protein content at 90°C and 100°C took a slight upward trend, while the content increased faster and then showed a relatively flat trend under 110°C and 120°C treatment. At higher heating temperatures, a greater amount of soluble protein was observed over time, reaching about 5%, much higher than the 1–2% level at the beginning of the heating period. This reflected the protein degradation and the release of low-molecular-weight compounds, which was also found by Zhang et al.[Citation25]
Conclusion
With the increase of heating temperature and time, the trend of hardness, chewiness, and gumminess exhibited a similar four-phase change with two peaks at 90°C, 100°C, and 110°C, and the trend was not observed at 120°C. The peak values became less obvious with the increasing temperature. There was no drastically decrease in hardness under lower temperatures, such as 90°C and 100°C, even heating for a long time (> 100 min), while it decreased seriously when temperature increased. Therefore, a gradient heating procedure of sterilization could be designed, such as setting heating gradient as 90–100–110–120°C, in order to reduce the damage of muscle texture during preheating period. Analysis of SDS-PAGE showed that after cooking at different temperatures, the intensity of MHC band gradually decreased and finally diminished, inferring that the meat protein had changed. And the intensity of the heavy molecular weight bands increased, indicating the aggregation of myosin in the heating process. With the increase of heating time, the content of water-soluble proteins increased significantly. This indicated that cooking at high temperature for long time led to degradation of muscle proteins, causing the decreasing trend of texture and quality deterioration. In general, it might be the two interactive effects between degradation and aggregation of protein, causing the appearance of second peak and resulting the four-stage changes in hardness. These results may enrich and supply the theory of freshwater fish processing industry, providing a good basis for studying and producing fish products with longer shelf life.
Additional information
Funding
References
- FAO Fishery and Aquaculture Statistics, Roma, Fao Inter-Departmental Working Group, 2016, 30.
- Lorentzen, G.; Ytterstad, E.; Olsen, R. L.; Skjerdal, T. Thermal Inactivation and Growth Potential of Listeria Innocua in Rehydrated Salt-Cured Cod Prepared for Ready-To-Eat Products. Food Cont. 2010, 21, 1121–1126. DOI: 10.1016/j.foodcont.2010.01.006.
- Zhang, T.; Xue, Y.; Li, Z. J.; Wang, Y. M.; Yang, W. G.; Xue, C. H. Effects of Ozone on the Removal of Geosmin and the Physicochemical Properties of Fish Meat from Bighead Carp (Hypophthalmichthysnobilis). Inn. Food Sci. Emerging Technol. 2016, 34, 16–23. DOI: 10.1016/j.ifset.2016.01.001.
- Zhang, L. L.; Zhang, F. X.; Wang, X. Changes of Protein Secondary Structures of Pollock Surimi Gels under High-Temperature (100 °C and 120 °C) Treatment. J Food Eng. 2016, 171, 159–163. DOI: 10.1016/j.jfoodeng.2015.10.025.
- Tornberg, E.;. Effects of Heat on Meat Proteins - Implications on Structure and Quality of Meat Products. Meat Sci. 2005, 70, 493–508. DOI: 10.1016/j.meatsci.2004.11.021.
- Barbanti, D.; Pasquini, M. Influence of Cooking Conditions on Cooking Loss and Tenderness of Raw and Marinated Chicken Breast Meat. LWT Food Sci. Technol. 2005, 38, 895–901. DOI: 10.1016/j.lwt.2004.08.017.
- Dai, Y.; Lu, Y.; Wu, W.; Lu, X. M.; Han, Z. P.; Liu, Y.; Li, X. M.; Dai, R. T. Changes in Oxidation, Color and Texture Deteriorations during Refrigerated Storage of Ohmically and Water Bath-Cooked Pork Meat. Inn. Food Sci. Emerging Technol. 2014, 26, 341–346. DOI: 10.1016/j.ifset.2014.06.009.
- Christensen, M.; Purslow, P. P.; Larsen, L. M. The Effect of Cooking Temperature on Mechanical Properties of Whole Meat, Single Muscle Fibres and Perimysial Connective Tissue. Meat Sci. 2000, 55, 301–307.
- Combes, S.; Lepetit, J.; Darche, B.; Lebas, F. Effect of Cooking Temperature and Cooking Time on Warner-Bratzler Tenderness Measurement and Collagen Content in Rabbit Meat. Meat Sci. 2004, 66, 91–96. DOI: 10.1016/S0309-1740(03)00019-6.
- Schubring, R. Comparative Study of the DSC Pattern, Color, Texture and Water-Binding Capacity of Rainbow Trout Muscle during Heating. J. Food Process. Preservation 2008, 32, 190–218. DOI: 10.1111/j.1745-4549.2007.00174.x.
- Vasanthi, C.; Venkataramanujam, V.; Dushyanthan, K. Effect of Cooking Temperature and Time on the Physico-Chemical, Histological and Sensory Properties of Female Carabeef (Buffalo) Meat. Meat Sci. 2007, 76, 274–280. DOI: 10.1016/j.meatsci.2006.11.018.
- Kong, F. B.; Oliveira, A.; Tang, J.; Rasco, B.; Crapo, C. Salt Effect on Heat-Induced Physical and Chemical Changes of Salmon Fillet (O-Gorbuscha). Food Chem. 2008, 106, 957–966. DOI: 10.1016/j.foodchem.2007.07.008.
- Kong, F. B.; Tang, J.; Rasco, B.; Crapo, C. Kinetics of Salmon Quality Changes during Thermal Processing. J Food Eng. 2007, 83, 510–520. DOI: 10.1016/j.jfoodeng.2007.04.002.
- Barbosa-Canovas, G. V.; Medina-Meza, I.; Candogan, K.; Bermudez-Aguirre, D. Advanced Retorting, Microwave Assisted Thermal Sterilization (MATS), and Pressure Assisted Thermal Sterilization (PATS) to Process Meat Products. Meat Sci. 2014, 98, 420–434. DOI: 10.1016/j.meatsci.2014.06.027.
- Del Pulgar, J. S.; Gazquez, A.; Ruiz-Carrascal, J. Physico-Chemical, Textural and Structural Characteristics of Sous-Vide Cooked Pork Cheeks as Affected by Vacuum, Cooking Temperature, and Cooking Time. Meat Sci. 2012, 90, 828–835. DOI: 10.1016/j.meatsci.2011.11.024.
- Association of Official Analytical Chemists (AOAC) Official Methods of Analysis of the Association of Official Analytical Chemists. 16th edn. 1995, 69-74, Washington, DC: Assn of Official Analytical Chemists.
- Gornall, A. G.; Bardawill, C. J.; David, M. M. Determination of Serum Proteins by Means of Biuret Reaction. J. Biol. Chem. 1949, 177, 751–766.
- Mendes, R.; Vieira, H.; Pereira, J.; Teixeira, B. Water Uptake and Cooking Losses in Octopus Vulgaris during Industrial and Domestic Processing. LWT Food Sci. Technol. 2017, 78, 8–15. DOI: 10.1016/j.lwt.2016.11.087.
- Robinson, H. W.; Hogden, C. G. The Biuret Reaction in the Determination of Serum Proteins. 1. A Study of the Conditions Necessary for the Production of a Stable Color Which Bears a Quantitative Relationship to the Protein Concentration. J. Biol. Chem. 1940, 135, 707–725.
- Fritz, J. D.; Swartz, D. R.; Greaser, M. L. Factors Affecting Polyacrylamide Gel Electrophoresis and Electroblotting of High-Molecular-Weight Myofibrillar Proteins. Anal. Biochem. 1989, 180, 205–210.
- Laemmeli, U. K. Cleavage of Structural Proteins during the Assembly of the Head of Bacteriophage T4. Nature 1970, 227, 680–685.
- Chin, K. B.; Go, M. Y.; Xiong, Y. L. L. Konjac Flour Improved Textural and Water Retention Properties of Transglutaminase-Mediated, Heat-Induced Porcine Myofibrillar Protein Gel: Effect of Salt Level and Transglutaminase Incubation. Meat Sci. 2009, 81, 565–572. DOI: 10.1016/j.meatsci.2008.10.012.
- Purslow, P. P.; Oiseth, S.; Hughes, J.; Warner, R. D. The Structural Basis of Cooking Loss in Beef: Variations with Temperature and Ageing. Food Res. Int. 2016, 89, 739–748. DOI: 10.1016/j.foodres.2016.09.010.
- Bell, J. W.; Farkas, B. E.; Hale, S. A.; Lanier, T. C. Effect of Thermal Treatment on Moisture Transport during Steam Cooking of Skipjack Tuna (Katsuwonas Pelamis). J. Food Sci. 2010, 66, 307–313. DOI: 10.1111/j.1365-2621.2001.tb11337.x.
- Zhang, J. J.; Yao, Y. J.; Ye, X. Q.; Fang, Z. X.; Chen, J. C.; Wu, D.; Liu, D. H.; Hu, Y. Q. Effect of Cooking Temperatures on Protein Hydrolysates and Sensory Quality in Crucian Carp (Carassiusauratus) Soup. J Food Sci Technol. 2013, 50, 542–548. DOI: 10.1007/s13197-011-0376-2.
- Runglerdkriangkrai, J.; Banlue, K.; Raksakulthai, N. Quality of Fish Ball from Surimi as Affected by Starch and Sterilizing Conditions. Kasetsart Univ. Fish. Res. Bull. 2008, 32(1), 39-47.
- Zhang, L. L.; Xue, Y.; Xu, J.; Li, Z. J.; Xue, C. H. Effects of High-Temperature Treatment (≥100°C) on Alaska Pollock (Theragra Chalcogramma) Surimi Gels. J Food Eng. 2013, 115, 115–120. DOI: 10.1016/j.jfoodeng.2012.10.006.
- Runglerdkriangkrai, J.; Itoh, Y.; Kishi, A.; Obatake, A. Polymerization Behavior of Various Actomyosin through Disulfide Bonding upon Heating. Fish. Sci. 1999, 65, 304–309. DOI: 10.2331/fishsci.65.304.
- Jiang, Q. X.; Ma, Z. R.; Wang, S. S.; Xu, Y. S.; Tang, F. Y.; Xu, X. Q.; Yu, P. P.; Xia, W. S. Effect of Temperature on Protein Compositional Changes of Bighead Carp (Aristichthys Nobilis) Muscle and Exudates. Food Sci. Technol. Res. 2014, 20, 655–661. DOI: 10.3136/fstr.20.655.