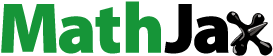
ABSTRACT
The purpose of this study was to evaluate the influence of oligochitosan (COS) as amino (–NH2) or carbonyl group (–C=O) donors on the antioxidant activities of COS Maillard reaction (MR) products. Two kinds of COS MR products (COS MRPs) were prepared by using COS and glucose/α-alanine, respectively. Their structures were characterized by Fourier transform infrared spectroscopy (FT-IR), their molecular weights (Mws) were measured by gel permeation chromatography, and the –NH2 contents of COS MRPs were determined. The antioxidant activities of COS MRPs were evaluated by measuring the scavenging activity of superoxide anion radical (O2−), 1,1-diphenyl-2-picrylhydrazyl radical, and the reducing power. The result showed that C=N absorbance can be observed in CG (MRPs of COS and glucose) and CA (MRPs of COS and α-alanine). The Mw of COS increased after MR. CG and CA showed dramatically enhanced antioxidant activity compared to COS, and the antioxidant activity of CG was better than that of CA. In other words, MR is an efficient way to enhance the antioxidant activity of COS. Moreover, it is a more efficient way to enhance the antioxidant activity of COS by using COS as amino group donor involved in MR. The results may provide some references to the modification and application of COS.
Introduction
Oligochitosan (COS) are linear co-oligomers of N-acetyl-d-glucosamine and d-glucosamine typically produced by partial hydrolysis from chitosan polymers. It typically differed from native chitosan by having an Mw of 10 kDa or less. They have recently attracted much attention because of its versatile positive biological activities[Citation1,Citation2] and have shown potential as scavenging agents, due to their ability to abstract hydrogen atoms from free radicals.[Citation3,Citation4] This ability has been reported as directly correlated with their structural properties – namely that the amino and hydroxyl groups can react with unstable free radicals to form stable macromolecule radicals.[Citation2,Citation5] Furthermore, their ready uptake by cells and the intestine, in addition with their claimed low toxicity, make COS very promising compounds for use as natural antioxidants.[Citation6,Citation7]
To improve the antioxidant activity of COS, COS derivatives including gallic acid-conjugated COS[Citation8] and -sulphated COS[Citation9] have been synthesized and their antioxidant activity was evaluated. The results do show that these COS derivatives exhibit higher antioxidant activity than COS. On the other hand, chemical modifications are generally not preferred for antioxidants used in food industry because of the formation of potential detrimental products.
Maillard reaction (MR) is a reaction involving the condensation between a carbonyl group of reducing sugars, aldehydes, or ketones and an amino group of amino acids, proteins, or any nitrogenous compound[Citation10] and is one of the main reactions taking place in food processing and storage. It had been recognized as a mild reaction with good controllability, no use of synthetic chemicals, and ease of operation in comparison with other chemical modification methods.
As amino group donor, the MR of COS or chitosan with monosaccharide, disaccharide, and polysaccharide has gained much attention.[Citation11,Citation12] The bioactivity such as antioxidant and antibacterial activities of obtained COS MRPs was changed compared with that of COS itself, and the change was related with the type of reducing sugar.[Citation13,Citation14] On the other hand, as its chemical structure possesses carbonyl group, COS can react with amino group leading to formation of MRPs. However, relevant researches are very little.[Citation15] In the present work, COS used as amino group (–NH2) or carbonyl group (–C=O) donor react with glucose (α-alanine) to inspect the influence on the antioxidant activity of COS MRPs. The result will provide some references for COS used as food preservative in food industry.
Materials and methods
Materials
COS was purchased from Zhejiang Jinke Biochemistry Co., Ltd, content ≥90%, degree of deacetylation (DD) – 70%. Luminol and DPPH (1,1-diphenyl-2-picrylhydrazyl) were purchased from Sigma-Aldrich. Glucose and α-alamine were purchased from Sinopharm Chemical Reagent Co., Ltd. Other reagents were all analytical grade.
Preparation and characterization of COS MRPS
The CG (MRPs of COS and glucose) and CA (MRPs of COS and α-alanine) were prepared according the previous method with small modification.[Citation16] Briefly, COS solution (5%, w/v) were mixed with glucose (3.2%, w/v) and α-alanine solution (0.70%, w/v) at a weight ratio of 1:1, respectively. The molar ratio of amino group (carbonyl group) of COS to carbonyl group of glucose (amino group of α-alanine) was 1:1. The mixture was heated at 80°C in reflux reactor for 12 h. The reaction was stopped by keeping the solution in an ice bath. Then, the reaction products were purified by dialysis (cut-off of Mw of 3500 Da, Thermo Scientific Inc., USA) against redistilled water for 3 days and further freeze-dried as CG and CA, respectively. COS solution (5%, w/v) without glucose (or α-alanine) repeated the above-mentioned process and the reaction products were named as the COS-12.
The structure of CG and CA was confirmed by FT-IR spectroscopy, which was taken with KBr pellets on an EQUNOX55FT-IR-Raman spectrophotometer with a revolution of 2 cm−1 in the range of 400–4000 cm−1. Mws of CG and CA were determined by gel permeation chromatography (GPC). The GPC was performed on a Waters-515 Chromatograph equipped with Waters 2410 refractive index detector and Ultrahydrogel 500 and 120. Elution was carried out using 0.07% Na2SO4 solution as the mobile phase at a flow rate of 0.5 mL/min. The temperatures of the column and detector were both maintained at 40°C during the determination process. The reference standard was glucan (Mw: 473,000, 188,000, 76,900, 43,200, 10,500, and 4000 Da, respectively). The –NH2 contents of COS MRPs were determined according to the Toei’s method with small modification.[Citation17] Briefly, 20 mg of the sample was dissolved in 10 mL acetic acid (0.1 mol/L) and completely stirred for 1 h at room temperature. The mixture was diluted with 40 mL distilled water, then 5 mL of the diluted solution was withdrawn and one drop of 1% toluidine blue was added as an indicator. Potassium polyvinyl sulfate solution (PVSK, N/400) was successively added until the titration end point was reached. Since the consumption of the N/400 PVSK (A mL, Eq. (1)) might correspond to that A of glucosamine unit in the water-soluble chitosan, the total weight of a glucosamine unit (X g, Eq. (1)) in the solution was obtained by Eq. (1):
where F is the factor of N/400 PVSK, 161 is the Mw of the glucosamine, and 203 is the Mw of the N-acetyl d-glucosamine. The water-soluble chitosan unit (Y g) is expressed as given by Eq. (2):
Thus, –NH2 contents (DD) are calculated by the following equation:
Antioxidant activity of COS MRPS
Scavenging activity of superoxide anion radical
Superoxide anion radical (O2−) scavenging activity of COS MRPs was evaluated by flow injection chemiluminescence (CL) on a chemical luminometer (IFFM-D, Xi’an, China). O2− was produced by a luminol-enhanced autoxidation of pyrogallol. The chemiluminescencent reaction was processed in a Na2CO3–NaHCO3 (pH = 10.20, 0.05 mol/L) buffer solution at room temperature. The COS MRPs were dissolved in Na2CO3–NaHCO3 buffer solution to prepare scavenger solutions at different concentrations. The scavenging activity of COS MRPs against O2− was evaluated according to their quenching effects on the CL signal of the luminol–pyrogallol system. The percentage inhibition of O2− scavenging was calculated using the following formula: scavenging activity (%) = (1 − Ai/A0) × 100, where A0 is the absorbance of control without the tested samples and Ai is the absorbance in the presence of the tested samples. The O2− was proved by SOD, catalase, and mannitol.[Citation18]
Scavenging activity of DPPH radical
The DPPH radical scavenging activity of the samples was measured using the modified method of Yamaguchi et al.[Citation19] Two milliliters of ethanolic solution of DPPH (0.1 mM) was incubated with varying concentrations of test samples (2.0 mL). The reaction mixture was shaken well and then kept at room temperature for 30 min under the dark and the absorbance of the resulting solution was measured at 517 nm against a blank. The radical scavenging activity was measured as a decrease in the absorbance of DPPH and was calculated as follows: [1 – (A517 nm sample – A517 nm control)/A517 nm blank] × 100%, where A517 nm sample was the absorbance of sample, A517 nm control was the absorbance of the control, and A517 nm blank was the absorbance of the blank.
Reducing power
The reducing power of all samples was determined by the method of Yen and Chen.[Citation20] Different concentrations of COS MRPs solutions (2.0 mL) were mixed with 2.5 mL sodium phosphate buffer (pH 6.6, 200 mM) and 2.5 mL of 1% potassium ferricyanide, and the mixtures were incubated at 50°C for 20 min. Trichloroacetic acid (10%, 2.5 mL) was added and the mixtures were centrifuged at 3000 rpm for 10 min. The supernatant (2.0 mL) was mixed with 2.5 mL distilled water and 0.5 mL of 0.1% ferric chloride solution and the absorbance was measured at 700 nm. Increased absorbance of the reaction mixture indicated increased reducing power.
Statistical analysis
All experiments were carried out in triplicate, and average values with standard deviation errors were reported. This means separation and significance were analysed using the IBM SPSS 20 software. Independent t-test was used to determine the level of significance (Originpro 8.0, p < 0.05).
Results and discussion
FT-IR, Mw, and the –NH2 contents of COS MRPS
FT-IR was used for the analysis of polymeric molecule in the reaction. As shown in , the characteristics adsorption bands at around 1158 and 892 cm−1 assigned to the C–O stretching and the b-D-configuration of COS were also observed in CG (MRPs of COS and glucose) and CA (MRPs of COS and α-alanine). The absorption bands at around 1621 cm−1, around 1515 cm−1 and around 1379 cm−1 in COS are assigned to amide Ι(C=O), amide ΙΙ(–NH2), and amid ΙΙΙ(C–N).[Citation21] A decrease of the band at about 1518 cm−1 corresponding to primary amine groups was detected in CG, indicating the successful interaction between the carbonyl group of the reducing sugar and the amine group of COS.[Citation22] Moreover, the band at around 1630 cm−1 showed an increase due to the appearance of a new band corresponding to C=N linkage,[Citation23] indicating the formation of Schiff base in CG. The band at around 1621 cm−1 in CA was shifted to around 1630 cm−1 indicating the formation of Schiff base in CA. A decrease of the band at about 1515 cm−1 and a band at around 1621 cm−1 was shifted to around 1630 cm−1 indicating the formation of Schiff base in COS-12.
The Mw of COS and its derivatives was measured by GPC. The Mw of COS was 8370 Da, and the Mw of CG, CA, and COS-12 was 12440, 11080, and 10500 Da, respectively. The Mw of COS MRPs increased after MR. The increased Mws may be related to the condensation of COS and glucose (α-alanine) during MR. The higher Mw of CG than that of CA may be related to the higher content of amino group (DD: 70%) in COS. Compared to MR between COS and small molecular (glucose and α-alanine), the MR between COS itself is more difficult because of steric hindrance. This may be the result for the lower Mw of COS-12.
The –NH2 content of COS was 0.70, and the –NH2 contents of CG, CA, and COS-12 were 0.29, 0.73, and 0.50, respectively. The –NH2 content of CG decreased sharply during MR between COS and glucose. The –NH2 content of CA showed a slight increase during MR.
Scavenging activity of superoxide anion radical
Superoxide anion radical is formed in almost all aerobic cells and is a major agent in the mechanism of oxygen toxicity.[Citation24] Superoxide anion radical shows as a relatively weak oxidant and exhibits limited chemical reactivity, but it can generate dangerous species, including singlet oxygen and hydroxyl radicals, which will cause the peroxidation of lipids.[Citation25,Citation26] Compared with other oxygen radicals, superoxide anion has a longer lifetime, can move to an aim at a longer distance, and thus is more dangerous. Therefore, it is very important to study the scavenging of superoxide anion. The COS MRPs showed good O2− scavenging activity in a dose-dependent manner (). The 50% inhibiting concentration (IC50) of CG (MRPs of COS and glucose), CA (MRPs of COS and α-alanine), COS-12 and COS was 2.1, 4.9, 6.2 and 10.6 mg/mL, respectively. CG showed the highest O2− scavenging activity. The COS-12 showed stronger O2− scavenging activity than COS and weaker O2− scavenging activity than CA. The order of O2− scavenging activity was as follows: CG > CA > COS-12 > COS. The antioxidant activity of COS MRPs mainly related to the content of active hydroxyl and amino group in the polymer chains.[Citation27] In fact, the antioxidant activity of chitosan derivatives increased with increasing of the DD caused by the increasing primary amino groups[Citation28] and with the decreasing of Mw causing the inter- and intramolecular hydrogen bonds partly destroyed.[Citation29,Citation30] In this study, MR of COS with glucose caused the decreasing amino group content and the increasing Mw in CG, which is not conducive to the improvement of antioxidant of CG. CG showed the best antioxidant activity may be related to the fact that the O2− scavenging activity of COS MRPs might be attributed to the hydrogen-donating ability of COS that broke the free radical chain and donated a hydrogen atom.[Citation31] After MR, the inter- and intramolecular hydrogen bonds in COS were partly destroyed, more hydroxyl and amino groups might be exposed, resulting in stronger hydrogen donating ability. Moreover, the intermediate MRPs are known as reductones that exhibited high antioxidant activity due to their ability to donate hydrogen atoms.[Citation32] Those facts may result in the improvement of O2− scavenging activity. The further mechanism should be studied in the future.
Scavenging activity of DPPH radical
DPPH possesses a proton-free radical with characteristic absorption, decreasing significantly when exposure to proton radical scavengers. Hence, the relatively stable DPPH radical has been widely used to test the ability of a compound as free radical scavenger or hydrogen donor to evaluate the antioxidant activity. showed that the DPPH scavenging activity of COS increased after MR and the CG (MRPs of COS and glucose) showed highest DPPH scavenging activity. The order of DPPH scavenging activity was as follows: CG > CA > COS-12 > COS. The result has the same trend with the O2− scavenging activity of COS MRPs.
Reducing power
Antioxidants with reducing power are electron donors and reduce the oxidized intermediates of lipid peroxidation, as primary and secondary antioxidants.[Citation33] The reducing properties are generally associated with the presence of reductones, which have been shown to exert antioxidant action by breaking the free radicals’ chain by donating a hydrogen atom.[Citation34] It can be determined by measuring the formation of Perls’ Prussian blue at 700 nm. A higher absorbance indicates a higher ferric reducing power.[Citation35] The reducing power of COS MRPs is shown in . The absorbance of CG (MRPs of COS and glucose), CA (MRPs of COS and α-alanine), COS-12 and COS at the 2.0 mg/mL concentration was 0.98, 0.66, 0.38 and 0.35, respectively. Higher absorbance value indicates stronger reducing power. This showed the order of the reducing power was as follows: CG > CA > COS-12 > COS. The reducing power of COS MPRs might be attributed to the hydrogen-donating ability of COS that broke the free radical chain and donated a hydrogen atom.[Citation36] The improved reducing power could be explained by the conjugates, contributed to the advanced MRPs (melanoidins).
Conclusion
Even though the precise mechanism of radical scavenging activity of COS is not clear, radical scavenging activity is attributed to that amino and hydroxyl groups attached to C-2, C-3 and C-6 positions of the pyranose ring, which react with unstable free radicals to form stable macromolecule radicals. The amino groups were partially consumed during MR of COS with glucose and the –NH2 content of CG (MRPs of COS and glucose) was the lowest, but antioxidant activity of CG is increased and stronger than that of CA (MRPs of COS and α-alanine) (when COS as a donor of carboxyl group). The results may be related to the different structure of the COS MRPs. In the future, the mechanism controlling the above observations clearly requires additional study.
Highlights
MR of oligochitosan as amino (carbonyl) group donators occured.
Antioxidant activity of product is better as oligochitosan as amino group donator.
Antioxidant activity increased although amino group of oligochitosan was consumed.
Additional information
Funding
References
- Eaton, P.; Fernandes, J. C.; Pereira, E.; Pintado, M. E.; Xavier, M. F. Atomic Force Microscopy Study of the Antibacterial Effects of Chitosans on Escherichia coli and Staphylococcus aureus. Ultramicroscopy. 2008, 108, 1128–1134. DOI: 10.1016/j.ultramic.2008.04.015.
- Kim, S. K.; Rajapakse, N. Enzymatic Production and Biological Activities of Chitosan Oligosaccharides (COS): A Review. Carbohydr. Polym. 2005, 62, 357–368. DOI: 10.1016/j.carbpol.2005.08.012.
- Huang, R.; Mendis, E.; Kim, S. K. Improvement of ACE Inhibitory Activity of Chitooligosaccharides (COS) by Carboxyl Modification. Bioorgan Med. Chem. 2005, 13, 3649–3655. DOI: 10.1016/j.bmc.2005.03.034.
- Kjell, M. V.; Mette, H. O.; Olav, S. Water-Solubility of Partially N-Acetylated Chitosans as a Function of pH: Effect of Chemical Composition and Depolymerisation. Carbohydr. Polym. 1994, 25, 65–70. DOI: 10.1016/0144-8617(94)90140-6.
- Je, J. Y.; Park, P. J.; Kim, S. K. Free Radical Scavenging Properties of Hetero-Chitooligosaccharides Using an ESR Spectroscopy. Food Chem. Toxicol. 2004, 42, 381–387. DOI: 10.1016/j.fct.2003.10.001.
- Chae, S. Y.; Son, S.; Lee, M.; Jang, M. K.; Nah, J. W. Deoxycholic Acid-Conjugated Chitosan Oligosaccharide Nanoparticles for Efficient Gene Carrier. J. Control Release. 2005, 109, 330–344. DOI: 10.1016/j.jconrel.2005.09.040.
- Fernandes, J. C.; Eaton, P.; Nascimento, H.; Belo, L.; Rocha, S.; Vitorino, R.; Amado, F.; Gomes, J.; Santos-Silva, A.; Pintado, M. E.; et al. Effects of Chitooligosaccharides on Human Red Blood Cell Morphology and Membrane Protein Structure. Biomacromolecules. 2008, 9, 3346–3352. DOI: 10.1021/bm800622f.
- Ngo, D. H.; Qian, Z. J.; Vo, T. S.; Ryu, B. M.; Ngo, D. N.; Kim, S. K. Antioxidant Activity of Gallate-Chitooligosaccharides in Mouse Macrophage RAW264.7 Cells. Carbohydr. Polym. 2011, 84, 1282–1288. DOI: 10.1016/j.carbpol.2011.01.022.
- Lu, X.; Guo, H.; Zhang, Y. Protective Effects of Sulfated Chitooligosaccharides against Hydrogen Peroxide-Induced Damage in MIN6 Cells. Int. J. Biol. Macromol. 2012, 50, 50–58. DOI: 10.1016/j.ijbiomac.2011.09.020.
- Hodge, J. E. Dehydrated Foods. Chemistry of Browning Reactions in Model Systems. J. Agr. Food Chem. 1953, 1, 625–651. DOI: 10.1021/jf60015a004.
- Chang, H. L.; Chen, Y. C.; Tan, F. J. Antioxidative Properties of a Chitosan–Glucose Maillard Reaction Product and Its Effect on Pork Qualities during Refrigerated Storage. Food Chem. 2011, 124, 589–595. DOI: 10.1016/j.foodchem.2010.06.080.
- Li, S. L.; Lin, J.; Chen, X. M. Effect of Chitosan Molecular Weight on the Functional Properties of Chitosan-Maltose Maillard Reaction Products and Their Application to Fresh-Cut Typha latifolia L. Carbohydr. Polym. 2014, 102, 682–690. DOI: 10.1016/j.carbpol.2013.10.102.
- Wu, S.; Hu, J.; Wei, L.; Du, Y.; Shi, X.; Zhang, L. Antioxidant and Antimicrobial Activity of Maillard Reaction Products from Xylan with Chitosan/Chitooligomer/Glucosamine Hydrochloride/Taurine Model Systems. Food Chem. 2014, 148, 196–203. DOI: 10.1016/j.foodchem.2013.10.044.
- Sun, T.; Qin, Y. Y.; Xu, H. L.; Xie, J.; Hu, D. M.; Xue, B.; Hua, X. Y. Antibacterial Activities and Preservative Effect of Chitosan Oligosaccharide Maillard Reaction Products on Penaeus vannamei. Int. J. Biol. Macromol. 2017, 105, 764–768. DOI: 10.1016/j.ijbiomac.2017.07.100.
- Jung, W. K.; Park, P. J.; Ahn, C. B.; Je, J. Y. Preparation and Antioxidant Potential of Maillard Reaction Products from (MRPs) Chitooligomer. Food Chem. 2014, 145, 173–178. DOI: 10.1016/j.foodchem.2013.08.042.
- Sun, T.; Chen, C. H.; Zhu, Y.; Xie, J. Antioxidant Activity Assessment of Chitosan Oligosaccharide Derivatives from Maillard Reaction with Glucose. Food Sci. 2011, 32, 14–18.
- Toei, K.; Sawada, M. A Turbidimetric Method for Colloid Titrations. Anal. Chim. Acta. 1976, 83, 59–65. DOI: 10.1016/S0003-2670(01)84736-X.
- Sun, T.; Zhu, Y.; Xie, J.; Yin, X. Antioxidant Activity of N-Acyl Chitosan Oligosaccharide with Same Substituting Degree. Bioorgan Med. Chem. 2011, 21, 798–800. DOI: 10.1016/j.bmcl.2010.11.097.
- Yamaguchi, T.; Takamura, H.; Matoba, T.; Terao, J. HPLC Method for Evaluation of the Free Radical-Scavenging Activity of Foods by Using 1, 1-Diphenyl-2-Picrylhydrazyl. Biosci. Biotech. Bioch. 1998, 62, 1201–1204. DOI: 10.1271/bbb.62.1201.
- Yen, G. C.; Chen, H. Y. Antioxidant Activity of Various Tea Extracts in Relation to Their Antimutagenicity. J. Agric. Food Chem. 1995, 43, 27–32. DOI: 10.1021/jf00049a007.
- Zheng, Z.; Luo, Y.; Yao, L.; Lu, J.; Bu, G. Effects of Maillard Reaction Conditions on the Functional Properties of WPI Chitosan Oligosaccharide Conjugates. J. Food Sci. Technol. 2014, 51, 3794–3802. DOI: 10.1007/s13197-013-0946-6.
- Chang, X. H.; Chen, D. R.; Jiao, X. L. Chitosan-Based Aerogels with High Adsorption Performance. J. Phys. Chem. B. 2008, 112, 7721–7725. DOI: 10.1021/jp8011359.
- Umemura, K.; Kawai, S. Modification of Chitosan by the Maillard Reaction Using Cellulose Model Compounds. Carbohydr. Polym. 2007, 68, 242–248. DOI: 10.1016/j.carbpol.2006.12.014.
- Fridovich, I. The Biology of Oxygen Radicals. Science 1978, 201, 875–880.
- Banerjee, A.; Dasgupta, N.; De, B. In Vitro Study of Antioxidant Activity of Syzygium Cumini Fruit. Food Chem. 2005, 90, 727–733. DOI: 10.1016/j.foodchem.2004.04.033.
- Singh, N.; Rajini, P. S. Free Radical Scavenging Activity of an Aqueous Extract of Potato Peel. Food Chem. 2004, 85, 611–616. DOI: 10.1016/j.foodchem.2003.07.003.
- Feng, T.; Du, Y.; Li, J.; Hu, Y.; Kennedy, J. F. Enhancement of Antioxidant Activity of Chitosan by Irradiation. Carbohydr. Polym. 2008, 73, 126–132. DOI: 10.1016/j.carbpol.2007.11.003.
- Yen, M. T.; Yang, J. H.; Mau, J. L. Antioxidant Properties of Chitosan from Crab Shells. Carbohydr. Polym. 2008, 74, 840–844. DOI: 10.1016/j.carbpol.2008.05.003.
- Chien, P. J.; Sheu, F.; Huang, W. T.; Su, M. S. Effect of Molecular Weight of Chitosans on Their Antioxidative Activities in Apple Juice. Food Chem. 2007, 102, 1192–1198. DOI: 10.1016/j.foodchem.2006.07.007.
- Xing, R.; Liu, S.; Guo, Z.; Yu, H.; Zhong, Z.; Ji, X.; Li, P. Relevance of Molecular Weight of Chitosan-N-2-Hydroxypropyl Trimethyl Ammonium Chloride and Their Antioxidant Activities. Eur. J. Med. Chem. 2008, 43, 336–340. DOI: 10.1016/j.ejmech.2007.03.025.
- Ying, G. Q.; Xiong, W. Y.; Wang, H.; Sun, Y.; Liu, H. Z. Preparation, Water Solubility and Antioxidant Activity of Branched-Chain Chitosan Derivatives. Carbohydr. Polym. 2011, 83, 1787–1796. DOI: 10.1016/j.carbpol.2010.10.037.
- Silván, J. M.; Van, L. J.; Olano, A.; Del, Castillo, M. D. Corrigendum to “Analysis and Biological Properties of Amino Acid Derivates Formed by Maillard Reaction in Foods”. J. Pharm. Biomed. Anal. 2007, 43, 1543–1551. DOI: 10.1016/j.jpba.2006.11.001.
- Farvin, K. H. S.; Jacobsen, C. Phenolic Compounds and Antioxidant Activities of Selected Species of Seaweeds from Danish Coast. Food Chem. 2013, 138, 1670–1681. DOI: 10.1016/j.foodchem.2012.10.078.
- Duh, P. D.; Du, P. C.; Yen, G. C. Action of Methanolic Extract of Mung Bean Hulls as Inhibitors of Lipid Peroxidation and Non-Lipid Oxidative Damage. Food Chem. Toxicol. 1999, 37, 1055–1061. DOI: 10.1016/S0278-6915(99)00096-4.
- Ercan, B.; Köksal, E. Evaluation of Reducing Power and Radical Scavenging Activities of Water and Ethanol Extracts from Sumac (Rhus coriaria L.). Food Res. Int. 2010, 44, 2217–2221. DOI: 10.1016/j.foodres.2010.11.001.
- Jarmila, V.; Vavríková, E. Chitosan Derivatives with Antimicrobial, Antitumour and Antioxidant Activities – A Review. Curr. Pharm. Design. 2011, 17, 3596–3607. DOI: 10.2174/138161211798194468.